Chapter 3 César Fernández-de-las-Peñas; José L. Arias-Buría; Jan Dommerholt Although this textbook’s primary focus is on trigger point (TrP) dry needling (DN), in clinical practice healthcare providers are using DN for several other indications, including fascial adhesions, scar tissue, tendinopathies, or enthesopathies. Already in 1979, Lewit described needling of many structures in the body (Lewit, 1979), and it is remarkable that it has taken a few decades before clinicians started using DN routinely for other conditions. There are only a few published case reports on fascial dry needling (Finnoff & Rajasekaran, 2016; Anandkumar & Manivasagam, 2017), but the theoretical basis for using needles in the treatment of fascial adhesions and scar tissue has developed sufficiently to consider its use in the clinic (Chiquet et al., 2003; Grinnell, 2003; Finando & Finando, 2011; Langevin et al., 2011). During the past decade, there has been a worldwide focus on fascial function and dysfunction with many new insights and clinical guidelines (Chaudhry et al., 2007; Huijing, 2009; Findley, 2012; Findley et al., 2012; Willard et al., 2012; Adstrum et al., 2017). The literature of DN for tendinopathy is slightly more advanced compared with that for fascial needling; however, it is still in its infancy (Nagraba et al., 2013; Krey et al., 2015). There is poor consensus on possible mechanisms of tendon DN and on optimal treatment parameters, indications, and contraindications. Tendinopathies are very common, but there is little evidence for any of the commonly used therapeutic interventions, including eccentric loading (Camargo et al., 2014), manipulations and mobilisations (Pfefer et al., 2009), friction massage (Joseph et al., 2012), fascial manipulation (Pedrelli et al., 2009), ultrasound (Desmeules et al., 2015), laser therapy (Pfefer et al., 2009), orthotics (Simpson & Howard, 2009), or antiinflammatory and glucocorticoid injections (Andres & Murrell, 2008). Eccentric strengthening and low-level laser therapy look promising, but higher-quality studies are needed (Andres & Murrell, 2008; Haslerud et al., 2015). There is only anecdotal evidence for DN for enthesopathies. This chapter provides an overview of pertinent fascia, tendinopathy, and enthesopathy literature in the context of DN and describes needling procedures using solid filament needles. It is perhaps a bit surprising that there are no entries for the word ‘fascia’ in the index of Travell and Simons Trigger Point Manuals, even though Travell and Rinzler already realised the importance of fascia for myofascial pain conditions in the 1950s (Travell & Rinzler, 1952; Travell & Simons, 1992; Simons et al., 1999). To be fair to Travell, knowledge about fascia was practically nonexistant when she developed the myofascial pain construct. Much has changed during the past decades, and today the role of fascia as a potential peripheral source of nociception is widely recognised (Tesarz et al., 2011). During the 2015 4th Fascia Research Congress in Washington, DC, fascia was defined anatomically as ‘a sheath, a sheet, or any number of other dissectible aggregations of connective tissue that forms beneath the skin to attach, enclose, separate muscles and internal organs’ (Stecco & Schleip, 2016). This definition was not necessarily acceptable to all clinicians and researchers (Scarr, 2017); thus a new committee was formed to define ‘the fascial system’, which was described in 2017 as: The fascial system consists of the three-dimensional continuum of soft, collagen-containing, loose and dense fibrous connective tissues that permeate the body. It incorporates elements such as adipose tissue, adventitiae and neurovascular sheaths, aponeuroses, deep and superficial fasciae, epineurium, joint capsules, ligaments, membranes, meninges, myofascial expansions, periostea, retinacula, septa, tendons, visceral fasciae, and all the intramuscular and intermuscular connective tissues including endo-/peri-/epi-mysium. The fascial system interpenetrates and surrounds all organs, muscles, bones and nerve fibres, endowing the body with a functional structure, and providing an environment that enables all body systems to operate in an integrated manner (Adstrum et al., 2017). Dry needling (DN) and manual TrP therapy may alter the viscoelastic properties or behaviour of fascia tissue, which implies that manual fascial techniques should be incorporated into TrP therapy. As the definition of the fascial system indicates, fascia and muscles are intimately intertwined and connected. The epimysium surrounds all muscles, whereas individual muscle fibre bundles are situated within the perimysium, and individual muscle fibres are contained within the endomysium (Stecco et al., 2016a). The epimysium, perimysium, and endomysium are part of the deep fascia (Schleip et al., 2006; Roman et al., 2013), which must be distinguished from the superficial and visceral fascia (Stecco et al., 2016b). It is noteworthy that the definition of the fascial system does not include any reference to the sensory aspects of fascia. Yet to be a source of pain, fascia must contain sensory fibres and nociceptors (Tesarz et al., 2011). In reviewing the myofascial pain literature, it may appear that muscles are the main source of nociception, but several studies point to fascia instead. Weinkauf and colleagues (2015) found that mechanical hyperalgesia was more pronounced in the tibial fascia than in the muscle after injecting nerve growth factor (NGF). NGF injections in the fascia of the erector spinae muscles also caused significant hyperalgesia, as well as a decreased pressure threshold and exercise-induced pain for 1 week and persistent sensitisation to chemical and mechanical stimuli for 2 weeks (Deising et al., 2012). The thoracolumbar fascia was more sensitive than the tibial fascia (Weinkauf et al., 2015). In another study comparing the tibial fascia with muscle, injections of hypertonic saline caused a greater delayed onset of muscle pain with anterior fascia injections compared with muscle injections (Gibson et al., 2009). Schilder and colleagues (2014) confirmed that hypertonic saline injections into the fascia resulted in significantly more pain than injections in the subcutis and muscle. Danielson and colleagues (2009) found peptidergic sensory nerve endings with antibodies for calcitonin gene-related peptide (CGRP) and substance P in the loose connective tissue of the patellar tendon. Also of interest is that, under pathological conditions, fascia is able to establish new nociceptive fibres that are immunoreactive to substance P (Sanchis-Alfonso & Rosello-Sastre, 2000). Ruffini, Pacini and free nerve endings have been identified in the deep fascia, retinacula, and bicipital aponeurosis (Stilwell, 1957; Yahia et al., 1993; Sanchis-Alfonso & Rosello-Sastre, 2000; Stecco et al., 2008). There is some evidence that Pacinian receptors may be involved in high-velocity manipulation (Schleip, 2003), but there are no studies investigating whether manual TrP therapy or DN specifically target Pacinian receptors. The free nerve endings are likely mechanoreceptors. Each muscle has specific connections with fascia (Stecco et al., 2007; Stecco et al., 2010, 2013b; Wilke et al., 2016); through these connections, force transmissions take place from muscles to bones and to deeper fascial layers. Close to 40% of muscle force is transmitted to adjacent structures and not through the muscle’s tendon (Smeulders et al., 2005). Often, muscle fibres do not run from one end of the muscle to the other end and therefore frequently do not connect directly to the muscle’s tendon. Instead, these fibres attach to other muscle fibres or to the intramuscular connective tissue (Hijikata & Ishikawa, 1997), which leads to epimuscular myofascial force transmission to connective tissues outside the muscle parameters (Huijing & Jaspers, 2005). The contractile force distribution onto other fascial structures contributes to increased joint stability and facilitates movement involving adjacent muscles (Findley, 2011). Changes in fascial pliability can lead to altered movement patterns, local overuse, and a loss of strength and coordination. Considering the aponeurotic fascia such as the thoracolumbar fascia, decreased mobility between its fascial layers will lead to stiffness and limited mobility. The aponeurotic fascia consists of two or three layers of parallel collagen fibre bundles oriented in different directions (Stecco et al., 2013a) and is directly connected to muscles and tendons (Willard et al., 2012; Vleeming et al., 2014). The deep fascia contains multiple mechanoreceptors (Langevin, 2006), and the ability of fascia to process mechanoreceptive input is entirely dependent on structural relationship with bone tissue and muscles (van der Wal, 2009). It transmits forces over greater distances than the epimysial fascia (Huijing & Baan, 2008). Interestingly, Maas and Sandercock (2009) demonstrated that although the soleus muscle in a cat does have strong mechanical connections with synergistic muscles, the force transmission from the soleus muscle does not appear to be affected by length changes of its synergists, which means that apparently not all muscles use the same mechanisms for force transmission. It is not possible to separate the epimysal fascia from the underlying muscle due to the many connections between the muscle and various fibrous septa of the epimysium, perimysium, and endomysium (Turrina et al., 2013). A decrease in flexibility of the epimysium may result in decreased mobility, especially in the elderly. The epimysium of older rats was much stiffer than the same structures in younger rats (Gao et al., 2008). Dense epimysium can restrict muscles in their function (Stecco et al., 2013a). Although the epimysium does not have any Pacini and Ruffini corpuscles, it plays an important role in proprioception because of its direct connections with muscle spindles (Maier, 1999). The perimysium can also increase muscle stiffness and appears to be more sensitive to changes in mechanical tension than the other intramuscular connective tissues, in spite of having direct connections with the epimysium and muscle fibres (Passerieux et al., 2007). Perimysium has a high density of fibroblasts (Schleip et al., 2006), which suggests that fascia may play a significant role in muscle contractibility and possibly in the formation of TrPs (Schleip et al., 2005, 2006). Fibroblasts are located within the extracellular matrix and play a key role in the synthesis of collagen, ground substance, elastin, and reticulin. They register force-induced deformations in their extracellular matrix, and mechanical stretching of fibroblasts regulates key extracellular matrix genes by stimulating the release of multiple substances such as paracrine growth factor (Schleip et al., 2006). Langevin and colleagues showed that the effects of needling can at least partially be explained by mechanical stimulation of fibroblasts (Langevin et al., 2001a, 2010). Under high tension, fibroblasts feature stress fibres and focal adhesions and appear lamellar in shape, whereas under low stress, they are more or less rounded structures (Grinnell, 2003; Miron-Mendoza et al., 2008; Langevin et al., 2010). Lamellar fibroblasts can differentiate into myofibroblasts complete with a contractile apparatus of actin microfilaments and nonmuscle myosin (Tomasek et al., 2002). In other words, fascia is not just a passive structure but can perform very slow contractions (Schleip et al., 2008). In fact, fibroblasts are also involved in wound closure, muscle contractions (Yahia et al., 1992, 1993), and adhesions of scars. There is a paucity of studies describing how DN may influence fascia. The work by Langevin and colleagues is exceptional in this regard as they evaluated the results of mechanical stimulation of fascia with an acupuncture needle (Langevin et al., 2001b, 2004, 2006). Rotating a needle causes tissue bundles, especially collagen, to adhere to the needle and create a mechanical bond between the needle and the tissue (Kimura et al., 1992; Langevin et al., 2002). Further rotating, or pistoning, the needle as is commonly used in DN will pull the tissues along the needling direction and create a mechanical stretch of the intermuscular connective tissue layers. Retaining the needle will provide a more continuous stretch of the fascia and muscle fibres and remodelling of the cytoskeleton. Langevin and colleagues (2001b) observed pulling of the subdermal tissue without structural changes in the dermis and muscle. Collagen fibres in a particular layer are oriented in the same plane and direction; however, they are at a 78 degree angle with the fibre direction in adjacent layers (Purslow, 2010; Benetazzo et al., 2011). This may have implications for manual TrP therapy. Chaudhry and colleagues found that a greater fibre angle makes collagen fibres more resistant to longitudinal stretching (Chaudhry et al., 2007, 2008, 2012). Because the muscle fibre direction does not necessarily match the fascia fibre direction, further research should examine whether stretching exercises after manual TrP release or DN have evidence-based value and, if so, what the optimal stretching methods entails. Rotating a needle that has been placed in a taut band or a TrP is often advocated in DN courses as the most direct method of stretching the taut band or muscle contracture (Gunn & Milbrandt, 1977). As every muscle fibre bundle is surrounded by fascial layers, the question emerges whether rotation of the needle actually stretches the taut band and muscle fibres or the deeper connective tissue fibres, or perhaps both muscle and fascia. In this context it is noteworthy that massage therapy can activate the mechano-transduction signaling pathways focal adhesion kinase (FAK) and extracellular signal–regulated kinase 1/2 (ERK1/2), potentiated mitochondrial biogenesis signaling (nuclear peroxisome proliferator–activated receptor g coactivator 1a [PGC-1a]) and increase mitochondrial biogenesis (Crane et al., 2012). Increasing the number of mitochondria would improve the energy metabolism in the muscle, which in turn may reduce focal adhesions (Jafri, 2014). It is not known whether DN triggers a similar response. Massage can also reduce the production of the inflammatory cytokines such as tumour necrosis factor-α and interleukin-6, which has also been described for DN (Shah & Gilliams, 2008). Stretching skeletal myocytes activates NADPH oxidase, which promotes the production of reactive oxygen species and accessibility of ryanodine receptors (Prosser et al., 2013; Jafri, 2014). Empirically, adhesions of scar tissue can be treated effectively with DN by placing a needle directly into each adhesion or densification and rotating the needle unidirectional as much as possible to the pain tolerance level of the patient (Fig. 3.1). When the needle releases, periodic tightening is indicated until the adhesion has been resolved. Again, rotating the needle causes mechanical stress, which may restore the scar’s mobility and pliability and immediately reduce the subject’s hyperalgesia and allodynia. The torque increases exponentially with rotating the needle, which can be objectively measured up to several centimetres from the needle location (Langevin et al., 2004). Persistent adhesions are likely due to dysregulation of extracellular matrix proteins. Rotating the needle also inhibits Rho-dependent kinase and suppresses the induction of the tenascin-C gene. One of the best approaches to accomplish the transcription of the tenascin-C gene and to positively affect fibronectin and collagen XII is to cyclically stretch fibroblasts in the extracellular matrix. Fibroblasts feature integrins, which are critical for mechanical force patterning (Chiquet et al., 2003). Tendinopathy is a common orthopaedic term that includes different conditions such as tendinitis, paratenonitis, and tendinosis. The most accepted term used to be tendinosis, which can be defined as an unsuccessful healing response within the tendon tissue (Khan et al., 2002); however, the term ‘tendinopathy’ is preferred as it does not imply a certain aetiology or pathology (Morrey et al., 2013). The clinical presentation of a tendinopathy includes localised tendon pain (particularly with loading), local tenderness to palpation, and impaired function. Several changes can occur with repetitive overuse of the tendons and a subsequent development of tendinopathy, including collagen disorientation, fibre disorganisation, fibre separation, increased concentration of proteoglycans, increased prominence of cells, focal area with neovascularisation, and even cell apoptosis (Cook et al., 2004). Nevertheless, structural changes of the tendon are not associated with pain. Therefore current theories suggest a role of altered nociceptive processing in pain associated with tendinopathies (Rio et al., 2014), which is supported by the presence of several algogenic substances and proinflammatory mediators in the pathological tendon (Danielson, 2009; Christensen et al., 2015). Because tendon abnormalities observed in subjects with tendinopathy generally include degeneration and a disordered arrangement of collagen fibres, cell infiltration, tenocytes disruption, or vascularity changes, several therapeutic biological approaches have been proposed for the management of tendinopathy, including platelet-rich plasma (PRP) injections (Lee, 2016) and percutaneous electrolysis (EPE) (Arias-Buría et al., 2015) combined with biomechanical stimuli such as eccentric exercises. PRP injections use a volume of autologous plasma with a platelet concentration higher than the baseline. EPE is a newly developed treatment approach with a galvanic current stimulation delivered into the tendon via a needle. Several questions arise regarding common biological interventions; for example, there is no agreement on the volume of PRP injections, platelet activation, the number of centrifugation steps during the preparation, the buffering of the PRP, and postinjection rehabilitation protocols (Peck et al., 2016). Similarly, no consensus exists on the dosage of galvanic electrical current with EPE, the optimal duration of treatments, or the frequency of the sessions. Furthermore, preliminary evidence suggests that injection of PRP or glucocorticoids is not superior to placebo injections for reducing pain in individuals with lateral epicondylalgia (Krogh et al., 2013a,b). Dunning and colleagues (2014) advocated using just a needle without the addition of biological agents for the treatment of tendons and connective tissues. Needling therapies for tendons include DN, sometimes referred to as tendon fenestration, and percutaneous needle tenotomy. Percutaneous needle tenotomy describes a technique using the bevel of a needle, usually referred to as a miniscalpel, to section or cut the affected tendon. Several researchers explored the effect of needle tenotomy using beveled needles of 20 to 22 gauge. A case series including individuals with chronic tendinopathy, who had failed physical therapy treatments, showed that ultrasound-guided percutaneous needle tenotomy, using a 22-gauge needle with a local anaesthetic, was effective for improving symptoms (Housner et al., 2009). In this study the patellar (n = 5) and Achilles (n = 4) tendons were the most affected (Housner et al., 2009), which was in agreement with the findings by Testa and colleagues (1999, 2002). Another study reported that ultrasound-guided percutaneous needle tenotomy combined with corticosteroid injections was also effective for patients with lateral elbow tendinosis for whom all other nonsurgical treatments had failed (McShane et al., 2006). Ultrasound-guided percutaneous needle tenotomy is recommended to ensure that the abnormal area of the tendon is accurately targeted with the needle. In fact, ultrasound identification of well-defined tendon abnormalities was a positive predictor for a successful ultrasound-guided needle tenotomy using a 22-gauge beveled needle (Kanaan et al., 2013). The presence or absence of hyperemia on Doppler imaging did not have the same predictive value. On the contrary, tendon fenestration consists of repeated insertions with a solid needle into an abnormal tendon with the goal of converting a degenerative process into an acute inflammatory condition. With the appropriate treatment, the inflammatory condition may progress to proper tendon healing. Although some authors have proposed to use ultrasound guidance for tendon fenestrations with a solid needle, it is mostly indicated for tenotomies with beveled needles (Jacobson et al., 2016). It is interesting to note that as early as 1979, Lewit described the application of DN, not only for TrPs, but also for many other tissues, including ligaments, scars, periosteum, tendons, entheses, and even joints. This historic paper described the onset of immediate analgaesia in 87% of the painful structures after the insertion of a solid needle into the targeted tissue (Lewit, 1979). Several other case reports have suggested that tendon DN with an acupuncture needle, alone or combined with other injection modalities, was effective for calcifications in the elbow (Zhu et al., 2008a) and the supraspinatus (Zhu et al., 2008b). A more recent case study reported that ultrasound guided tendon DN combined with exercise was effective for an individual with a supraspinatus tendinopathy (Settergren, 2013). Lubojacky (2009) reported that needling therapy was as effective as arthroscopic surgery for rotator cuff tendinopathy. Several high-quality studies of tendon needling therapies have been published in the past few years. Stenhouse and colleagues (2013) found that tendon needling with an acupuncture needle was equally effective to autologous conditioned plasma injections for decreasing pain and disability in subjects with refractory lateral elbow pain. Jacobson and colleagues (2015) recently found that ultrasound-guided tendon fenestration of gluteus medius, gluteus minimus, proximal hamstring, and tensor fascia latae tendon was effective for hip and pelvic pain. A recent meta-analysis of the treatment of chronic tendinopathies highlighted significant advantages of PRP vs DN in the short term; however, no significant differences with placebo or DN were observed after 6 months (Tsikopoulos et al., 2016). The analysis included patients with rotator cuff, patellar, and lateral epicondyle tendinopathies. Interestingly, a subgroup analysis found marginal benefits of PRP in those with rotator cuff tendinopathy but not within patellar or lateral epicondyle tendons (Tsikopoulos et al., 2016). A review by Krey and colleagues (2015) concluded that tendon needling was effective for improving self-reported outcomes in subjects with lateral epicondyle, Achilles and rotator cuff tendinopathies. A 2017 systematic review of percutaneous tendon tenotomies in patients with lateral epicondylalgia did not identify any controlled trials comparing percutaneous tenotomy to placebo or conservative treatment, which means that the authors could not reach any conclusions about its effectiveness (Mattie et al., 2017). There is no consensus on the different technical aspects of tendon needling, including the number of insertions, the optimum dosage and frequency of treatment sessions, or the duration and the intensity of needling (Krogh et al., 2013a,b). How many needles should be used? Should the needles be left in the tendon? Should needles be rotated as is commonly done with fascial DN? Jacobson and colleagues (2015) recommended 20 to 40 needle passes, whereas Stenhouse and colleagues (2013) described 40 to 50 needle insertions through the long axis of the tendon. Mishra and colleagues (2014) found, however, that needling a tendon only five times was sufficient and effective for the majority of individuals with lateral epicondylalgia. Empirically, the number of insertions depends on the clinical presentation of the patient and the response of the tissue during the needling procedure, realising that there are no objective parameters to determine the optimum response pattern. Similar to fascial needling, needles can be rotated with tendon needling to lengthen fibroblasts (Langevin et al., 2005) and reduce nociceptive input (Chiquet et al., 2003). Loyeung and Cobbin (2013) proposed needle retention for 21 minutes with manipulation of the needle every 3 minutes. Empirically, it is recommended to rotate the needle until the patient’s symptoms are provoked. Tendon needling can be performed with or without ultrasound guidance. As most clinicians do not have access to sonography, tendon needling will have to be based on the clinical presentation of the patient. Tenderness and pain with palpation are common with tendinopathy (Ramos et al., 2009). Placing the needle directly into the most painful region or spot of the tendon is recommended with or without needle rotation (Fig. 3.2). An alternative procedure is to insert the needle throughout the tendon, that is, parallel to the painful area (Fig. 3.3). With this approach, the needle can be either rotated or inserted over the tendon fibres.
Dry Needling for Fascia, Scar, and Tendon
Introduction
Fascia and scar dry needling
Introduction to Fascia
Sensory Aspects of Fascia
Biomechanical Aspects of Fascia
Dry Needling of Fascia
Dry Needling of Scar Tissue Adhesions
Dry needling and tendon
Clinical Reasoning for Tendon Needling
Needling Therapies for Tendons
Clinical Guidelines for Tendon Dry Needling
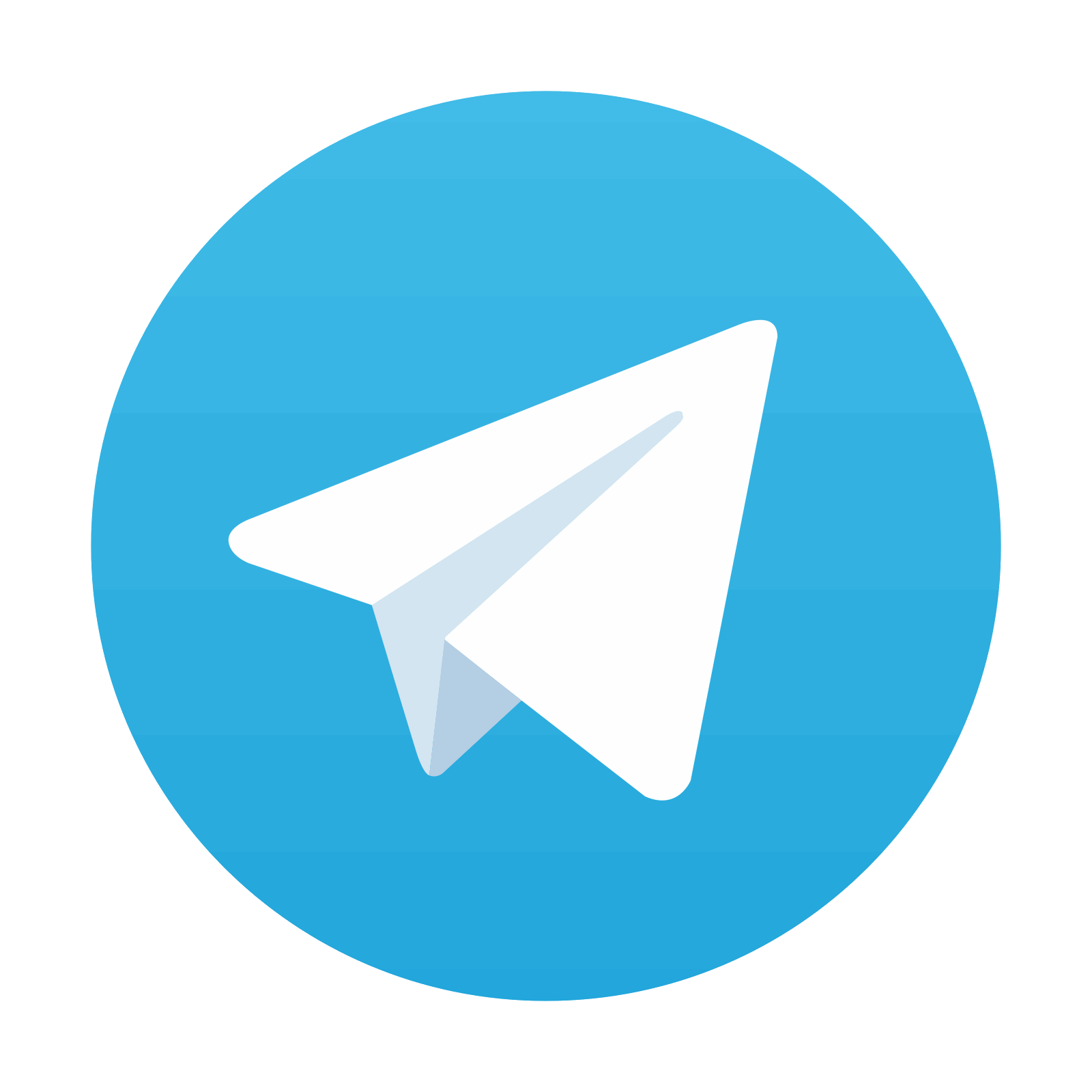
Stay updated, free articles. Join our Telegram channel

Full access? Get Clinical Tree
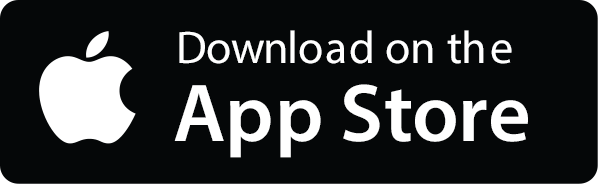
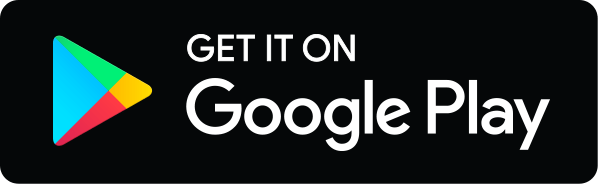