and Peter J. Millett2
(1)
Steadman Philippon Research Institute, Vail, CO, USA
(2)
The Steadman Clinic, Steadman Philippon Research Institute, Vail, CO, USA
Keywords
ShoulderBicepsLong headLabrumSLAP tearPhysical examClinical exam5.1 Introduction
Although its precise function remains relatively unknown, the long head of the biceps (LHB) tendon is likely a significant pain generator in a variety of shoulder conditions. Therefore, pathology related to the LHB tendon should be assessed in any patient with a condition related to the glenohumeral joint. Unfortunately, physical examination is often difficult due to confounding results and the lack of definitive research. However, an in-depth knowledge of relevant anatomy, biomechanics, and pathoanatomy can typically overcome these confounders and is instrumental in making any diagnosis or formulating a treatment plan related to the LHB tendon.
5.2 Anatomy and Biomechanics
5.2.1 Anatomy
The biceps brachii, innervated by the musculocutaneous nerve, has two heads that originate from different points on the scapula. The short head arises from the coracoid process as a single tendon combined with the coracobrachialis muscle (the conjoined tendon) and the long head arises from within the glenohumeral joint from both the supraglenoid tubercle and the adjacent glenoid labrum (Fig. 5.1). The tendon travels anterolaterally in the rotator interval and exits the joint through the bicipital groove which lies between the greater and lesser tuberosities of the proximal humerus. Travelling distally, the muscle bellies of each head coalesce, cross the cubital fossa and spiral towards their primary insertion on the bicipital tuberosity on the proximal radius (Fig. 5.2). This chapter will focus on the intra-articular portion of the LHB tendon since it is often implicated in the development of acute and chronic shoulder pain.
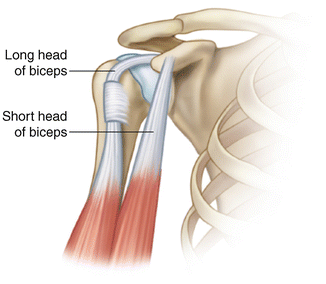
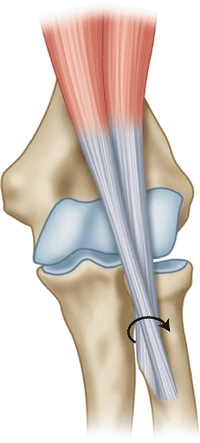
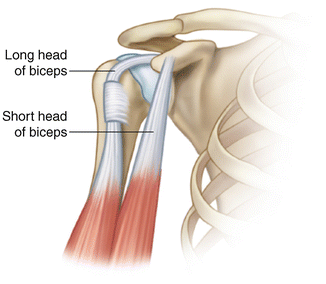
Fig. 5.1
Illustration depicting the basic anatomy of the long and short heads of the proximal biceps muscle.
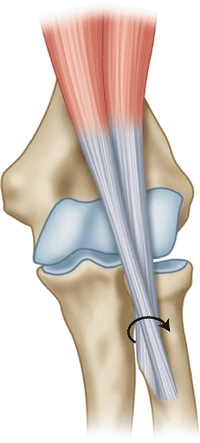
Fig. 5.2
Illustration demonstrating the rotation of the distal biceps tendon just before its insertion on the bicipital tuberosity.
The supraglenoid tubercle of the scapula has historically been described as the origin of the LHB tendon. However, several cadaveric studies have found that the superior labrum contributes significantly to the LHB origin [1–5]. In a series of 105 cadaveric shoulders, Vangsness Jr et al. [5] found that 40–60 % of the LHB tendon originated from the supraglenoid tubercle while the remaining fibers originated from the superior labrum (Fig. 5.3). Tuoheti et al. [4] dissected 101 cadaveric shoulders and found that the LHB tendon originated from both the supraglenoid tubercle and the glenoid in every case. In addition, Gigis et al. [2] noted that the LHB not only arises from both the supraglenoid tubercle and the labrum, but it also forms a portion of the posterosuperior labrum itself. An anatomic study of 16 cadaveric shoulders by Arai et al. [1] found that the fibers of the labrum became stiffer and stronger as the LHB tendon origin was approached. These studies support other claims that the torsional strain placed upon the superior labrum by the LHB tendon in positions of hyperabduction and external rotation is at least one factor involved in the development of superior labral anterior to posterior (SLAP) tears in throwing athletes (discussed later in this chapter) [6].
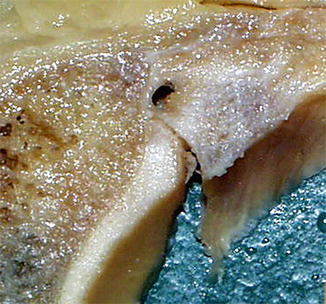
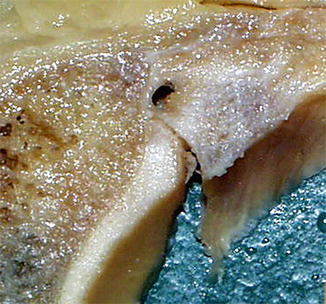
Fig. 5.3
Cadaveric photograph showing the insertion of the proximal biceps tendon on both the superior glenoid labrum and the supraglenoid tubercle.
One of the difficulties inherent to the diagnosis of SLAP tears, especially with regard to imaging studies, is the significant anatomic variability of the biceps anchor-superior labrum complex. According to Rao et al. [7], there are three predominant variations in superior labral anatomy that may be present in up to 10 % of the general population: the sublabral recess, the sublabral foramen, and the Buford complex (Figs. 5.4 and 5.5). The sublabral recess represents a potential space beneath the biceps anchor and the anterosuperior aspect of the glenoid labrum. The sublabral foramen is a small orifice located between the anterosuperior labrum and the articular cartilage of the anterior glenoid. The Buford complex is characterized by an absence of the anterosuperior labrum with a cord-like middle glenohumeral ligament that attaches directly to the superior labrum. It is crucial for the clinician to identify these findings as normal anatomic variants rather than pathologic lesions since inappropriate “repair” may lead to significant pain and external rotation loss as a result of stiffness [8, 9].
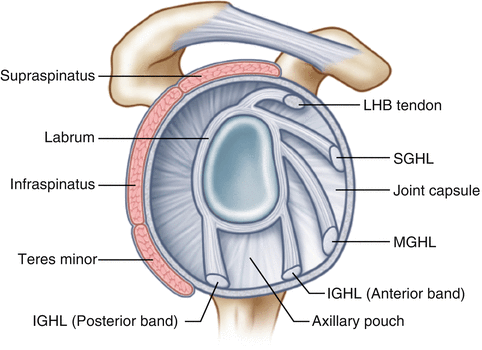
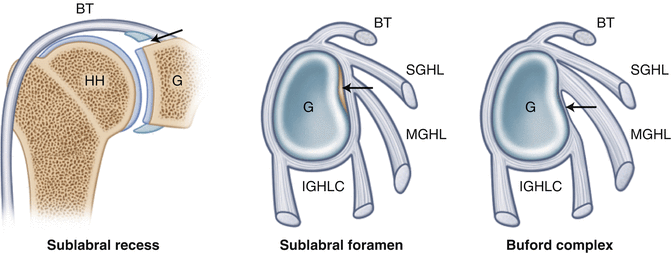
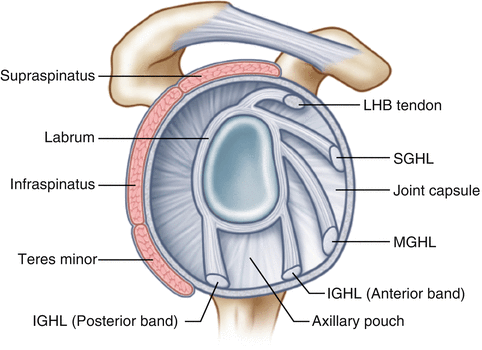
Fig. 5.4
Illustration depicting the normal anatomy of the glenohumeral capsuloligamentous structures.
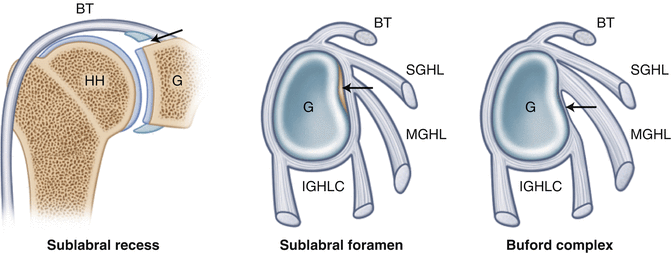
Fig. 5.5
Illustrations showing the most common glenolabral anatomic variations. The sublabral recess, sublabral foramen, and Buford complex are shown.
As the LHB tendon travels obliquely through the joint in an anterolateral direction, the tendon is encased in an outward-facing synovial membrane that is continuous with the joint capsule and renders the tendon extra-synovial [10]. As the tendon travels distally towards the bicipital groove of the proximal humerus, its position is maintained by the capsuloligamentous restraints within the rotator interval. The rotator interval is a triangular area in the anterosuperior aspect of the glenohumeral joint (Fig. 5.6). The medial base of the triangle is located at the coracoid process. The anterior margin of the supraspinatus and the superior margin of the subscapularis make up the superior and inferior borders of the rotator interval triangle, respectively. The lateral apex of the rotator interval is composed of the transverse humeral ligament which covers the bicipital groove and contributes to the bicipital sheath (discussed below). The contents of the interval include the LHB tendon, the superior glenohumeral ligament (SGHL), the coracohumeral ligament (CHL), and the glenohumeral joint capsule [11]. A more detailed description of the structure, function, and pathologies associated with the rotator interval can be found in Chap. 6.
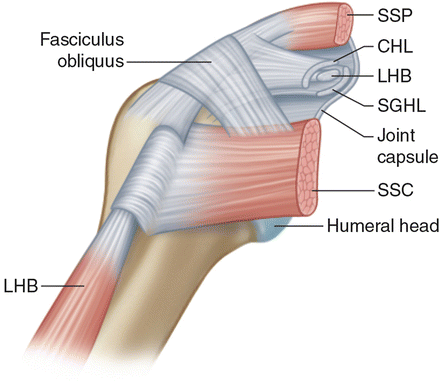
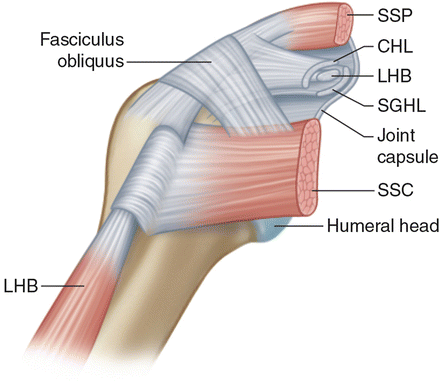
Fig. 5.6
Illustration highlighting the components of the rotator interval.
As the LHB tendon courses towards the bicipital groove, the SGHL and the CHL form a sling around the LHB tendon, primarily preventing its medial subluxation. This sling extends to the most anterior portion of the rotator cable and the biceps reflection pulley (BRP) at the proximal end of the bicipital groove [12]. The BRP, derived from the coalition of the SGHL, CHL, and the upper 1/3 of the subscapularis tendon, redirects the anterolateral course of the LHB tendon such that the tendon travels directly inferiorly along the anterior humeral shaft (Fig. 5.7). Habermeyer et al. [14] described a 30–40° inferior turn of the LHB tendon as it exited the joint via the BRP. Tearing of the subscapularis in this region, often known as a “pulley lesion,” can allow medial subluxation of the LHB tendon producing a painful “popping” or “snapping” sensation as the arm is moved (Fig. 5.8). In addition, a biomechanical study by Braun et al. [16] found that the LHB tendon slides up to 18 mm in and out of the joint with forward flexion and internal rotation, respectively. Therefore, the LHB tendon itself is subjected to significant mechanical stresses in the area of the BRP which can lead to tendonitis, tearing or rupture of the LHB.
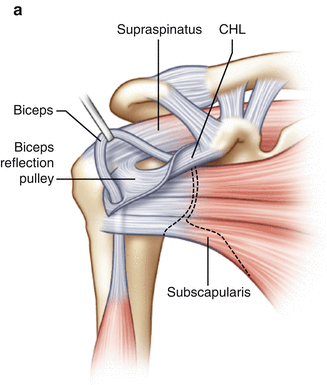
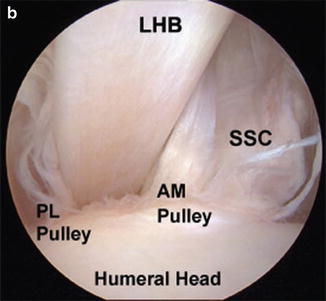
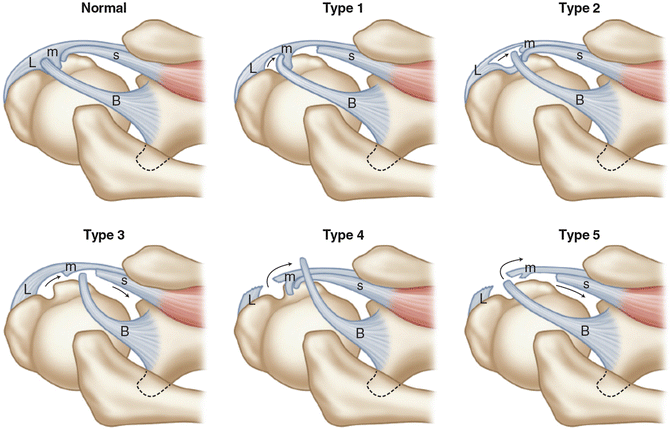
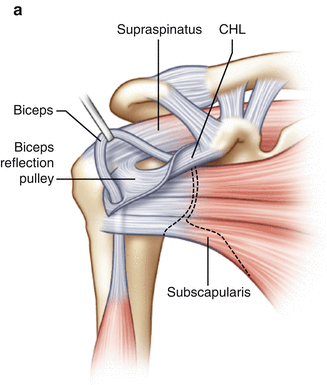
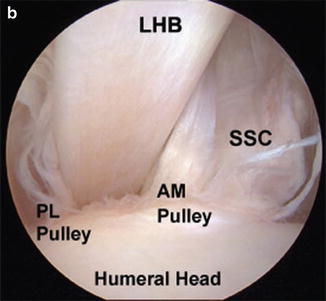
Fig. 5.7
(a) Illustration showing the structure of the bicipital sheath and biceps reflection pulley as the LHB tendon travels away from the glenohumeral joint. (b) Arthroscopic image showing anteromedial (AM) and posterolateral (PL) BRPs. (From Elser et al. [13]; with permission).
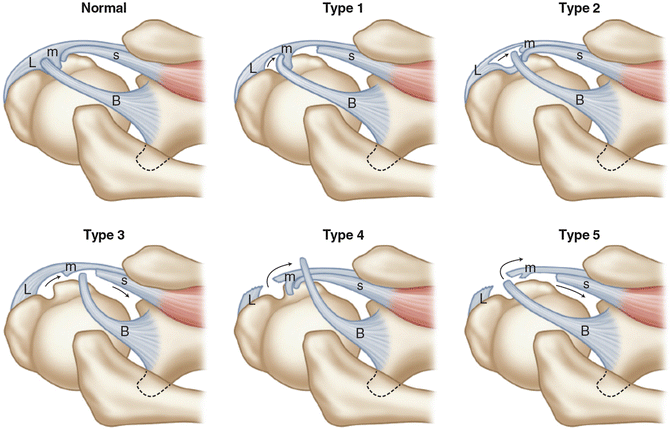
Fig. 5.8
Classification of pulley lesions as proposed by Bennett [15]. Note that medial subluxation of the LHB tendon is much more common than lateral subluxation.
The bicipital sheath is another complex structure through which the LHB tendon traverses as it passes through the bicipital groove (see Fig. 5.7). The floor of this sheath is formed from the coalescence of the SGHL and the subscapularis tendons at the superior aspect of the lesser tuberosity. These fibers then travel laterally, forming the floor of the bicipital sheath. The roof of the sheath is mostly composed of fibers from both the supraspinatus and CHL ligaments. All of these fibers (the floor and the roof of the sheath) combine to form a continuous ring around the LHB tendon as it passes through the bicipital groove thus providing tendon stability (Fig. 5.9). A recent biomechanical study by Kwon et al. [17] found that the subscapularis tendon was the most important stabilizer of the LHB tendon within the bicipital groove since tears of the subscapularis in this area almost always resulted in medial subluxation of the LHB tendon.
The bony structure of the bicipital groove can also play a role in pathologies of the LHB tendon. In a radiographic study by Pfahler et al. [18], the opening angle of the groove in patients without LHB tendon pathology was between 101° and 120° with the medial wall having a greater height than the lateral wall. Patients with a shallow groove or a lower medial wall may also be susceptible to subluxation of the LHB tendon.
The vascular supply to the LHB tendon near the biceps-labral complex is variably derived from the suprascapular, circumflex scapular and posterior circumflex humeral arteries [10]. Vascularity of the tendon is richest near its origin and dissipates prior to entering the bicipital groove where the tendon is avascular and fibrocartilaginous. This infrastructure helps prevent tendon injury from the sliding action of the LHB within the sheath of the groove. Similarly, innervation of the LHB tendon is concentrated near its anchor and dissipates as the tendon travels distally [19]. This arrangement was described as a “net-like” pattern of sympathetic fibers by Alpantaki et al. [19] in a cadaveric study using neurofilament antibodies (Fig. 5.10). In addition, Tosounidis et al. [20] demonstrated the presence of sympathetic α1-adrenergic receptors along the LHB tendon in cadaveric specimens with known acute and chronic shoulder conditions. These studies demonstrate that sympathetic innervation of the proximal LHB tendon may play a role in the pathogenesis of shoulder pain.
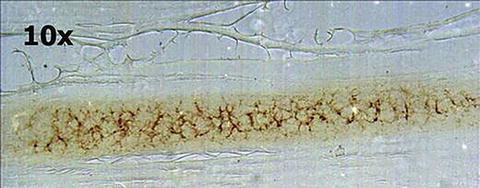
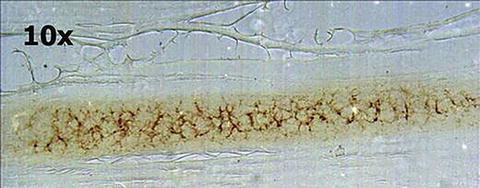
Fig. 5.10
Photomicrograph showing the attachment of neurofilament antibodies to the proximal LHB tendon in a “net-like” pattern (from Alpantaki et al. [19]; with permission).
5.2.2 Biomechanics
Although the anatomy of the proximal LHB tendon has been well described, its precise function has been debated for many years. Most studies that have aimed to describe its function are based on cadaveric models that focus on glenohumeral stability.
Pagnani et al. [21] used ten cadaveric shoulders to show that decreased anterior, superior, and inferior humeral head translation occurred when a simulated load of 55 N was applied to the LHB tendon in lower angles of elevation. Rodosky et al. [22] used a dynamic cadaveric shoulder model to simulate the forces typically applied to both the rotator cuff and the LHB tendons. They found that the LHB tendon contributed to glenohumeral stability by resisting torsional forces in the abducted and externally rotated position. The authors also noted a significantly increased strain applied to the anterior band of the inferior glenohumeral ligament (IGHL) when the biceps-labral complex was detached from its anchor at the superior aspect of the glenoid. This study provides some evidence that SLAP tears may contribute to increased anterior humeral head translation that is often found during physical examination (see Chap. 6 for more details regarding glenohumeral laxity and instability). More recently, Youm et al. [23] showed that the LHB tendon contributed significantly to anterior, posterior, superior, and inferior translation of the humeral head when a 22 N load was applied. Rotational range of motion and scapulohumeral kinematics were also affected when a load was applied to the LHB tendon. Alexander et al. [102] also noted a decrease in humeral head translation in all directions when a 20 N load was applied to the LHB tendon. Su et al. [24] studied the effects of the LHB tendon in cadaveric shoulders with variably sized rotator cuff tears. In their study, a significant decrease in anterosuperior and superior humeral head translation occurred when a 55 N load was applied to the LHB tendon.
Itoi et al. [25] contested that both the LHB and the short head of the biceps contribute significantly to glenohumeral joint stability, particularly in positions of abduction and external rotation when a simulated load of 1.5 and 3.0 kg were applied. This contribution to stability was particularly robust after attenuation of anterior stabilizing structures had occurred. In another biomechanical study, Kumar et al. [26] showed that loading of the short head of the biceps alone caused superior migration of the humeral head whereas tensioning of both the short head and the LHB simultaneously did not result in humeral head translation in any direction.
Although these studies suggest the role of the LHB tendon may be associated with glenohumeral stability, interpretation of dynamic shoulder models is difficult since replication of the in vivo environment, including complex force couples and resting tension, is quite difficult to achieve. In addition, the variability of simulated loads (11–55 N) makes their results difficult to compare, especially when the precise physiologic loads applied to the LHB tendon in different angles of abduction and rotation are currently unknown. Thus, it is possible that some studies may have obtained statistically significant results due to the application of non-physiologically high loads, making the results of these studies difficult to interpret.
To help answer these questions, electromyographic (EMG) studies have been performed to evaluate the effect of the LHB tendon on glenohumeral kinematics. However, their findings have been inconsistent to date. Levy et al. [27] found that the LHB tendon aided in glenohumeral stability both passively and in association with forearm supination or flexion. In contrast, Sakurai et al. [28] determined that the LHB tendon dynamically stabilized the humeral head, regardless of elbow activity. Other studies on pitching biomechanics found that the biceps may primarily function as a stabilizer of the elbow during flexion and supination with little effect on shoulder stability [29, 30]. Thus, the effect of the LHB tendon on glenohumeral kinematics remains controversial with respect to the most current EMG literature.
Both cadaveric and EMG studies have produced an incomplete picture of how the LHB tendon actually functions with regard to glenohumeral kinematics. Therefore, in vivo studies have also been conducted to help solve the mystery. Warner and MacMahon [31] studied a group of seven patients with rupture of the proximal LHB tendon and compared humeral head translation to their unaffected shoulders by true anteroposterior radiographs. In their study, radiographs were obtained in 0°, 45°, 90°, and 120° of abduction in the scapular plane. They found that superior migration of the humeral head was significantly increased in the shoulders with a ruptured LHB tendon compared to their contralateral, unaffected shoulders at all angles of abduction. Another radiographic study by Kido et al. [32] found similar results, noting that the humeral head was depressed as the LHB tendon was stimulated. However, the accuracy of radiographic studies has been called into question. Therefore, three-dimensional biplane fluoroscopy, a modality which has sub-millimeter accuracy, has been used to study in vivo kinematics with improved accuracy during full muscle activation. A study by Giphart et al. [33] found that, in five patients who underwent unilateral open subpectoral biceps tenodesis, humeral head translations of approximately 3 mm occurred in both the affected and unaffected shoulders during active elevation. The authors also studied various loading conditions such as forward flexion with maximal biceps activity on EMG, abduction to assess superior translation and a simulated throw (hyperabduction and external rotation) to evaluate anterior translation. Despite these loading conditions, the differences in translation between tenodesed and normal shoulders was always less than 1.0 mm, suggesting that the proximal LHB tendon may actually play a minimal role in glenohumeral kinematics in a shoulder that otherwise has an intact rotator cuff (Fig. 5.11).
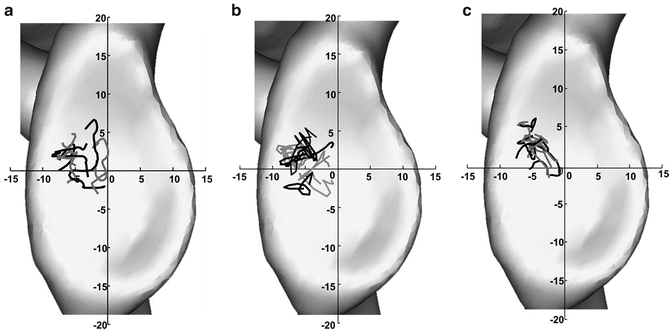
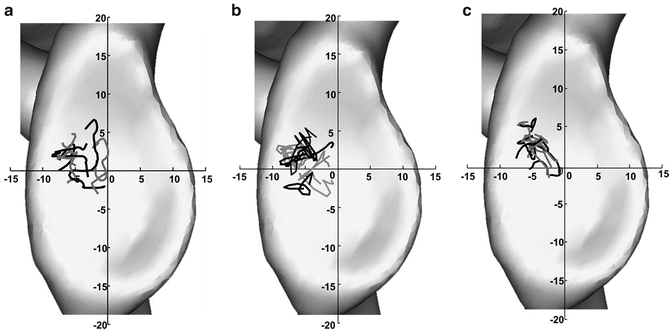
Fig. 5.11
Depiction of humeral head translation with (a) foward flexion, (b) abduction and (c) a simulated throwing motion. For each testing condition, biceps tenodesis resulted in minimal differences in humeral head translation when compared to the contralateral shoulder with various loading conditions.
The inconsistent and often contradictory findings of cadaveric, EMG, and in vivo studies have made it difficult to define the precise function of the proximal LHB tendon. While most of these studies have found that the LHB tendon may be involved with glenohumeral stability, further in vivo kinematic studies are necessary to completely elucidate the biomechanical role of the LHB tendon in shoulder motion and stability.
5.3 LHB Tendonitis, Tearing and Rupture
LHB tendonitis can occur as a result of impingement under the coracoacromial arch, subluxation out of the bicipital groove, or attrition as a result of degeneration [34]. Due to the many concurrent pathologies that are typically present in patients with LHB tendonitis, physical diagnosis is often difficult. However, a precise knowledge of the pathogenesis of LHB tendonitis will help the clinician synthesize a complete and accurate differential diagnosis with an understanding that overlapping conditions are often present.
5.3.1 Pathogenesis
Inflammation of the LHB tendon most often occurs secondarily as a result of surrounding pathologies such as impingement syndrome, pulley lesions, and/or degenerative rotator cuff tears (Fig. 5.12). Primary LHB tendonitis, in which there is isolated inflammation with no apparent cause, is not uncommon in clinical practice. Although isolated inflammation of the proximal LHB tendon can occur in overhead athletes, this may be the result of repetitive microtrauma as the tendon glides back and forth in a “sawing” motion within the bicipital groove and across the BRP. It is important to remember that because the LHB tendon is encased with synovium, inflammation within the shoulder may track proximally into the biceps-labral complex or distally into the bicipital groove.
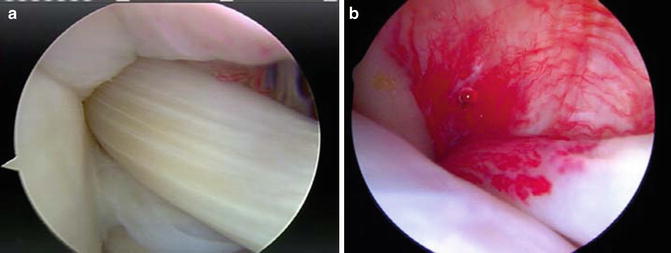
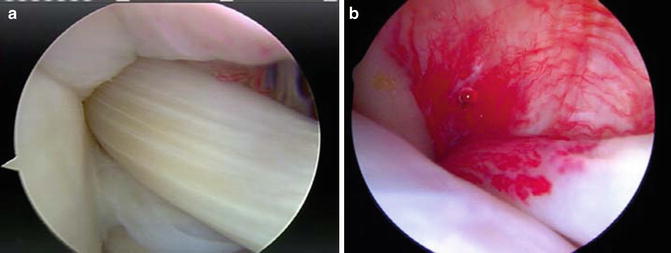
Fig. 5.12
Arthroscopic images demonstrating (a) a healthy LHB tendon and (b) an inflamed LHB tendon.
Subacromial impingement is the most common mechanism by which LHB tendonitis occurs, especially in older patients. Subcoracoid impingement can also lead to injury involving the LHB tendon and the BRP [35]. Weak rotator cuff and periscapular musculature potentially allow increased translation of the humeral head, narrowing the space available for the subacromial or subcoracoid contents to pass thus allowing impingement of these structures between the greater tuberosity and the undersurface of the acromion or between the lesser tuberosity and the coracoid (further details on subacromial impingement and subcoracoid impingement are provided in Chap. 4). In patients with subacromial impingement, LHB tendonitis almost always occurs simultaneously since the LHB is subject to the same mechanical wear from the coracoacromial arch. In addition, because the LHB tendon is encased in an outward-facing synovial membrane extending from the glenohumeral joint, any inflammatory process within the joint can thus involve the LHB tendon, producing painful inflammation and tenosynovitis (Fig. 5.12).
The acute stage of LHB tendonitis is characterized by significant anterior shoulder pain localized within the bicipital groove. The LHB tendon will swell and sometimes develop partial-thickness tearing at points of maximal wear. Later, the tendon further degenerates and may form adhesions with surrounding structures such as the bicipital sheath and rotator interval structures. In this stage, microscopic examination reveals fibrinoid necrosis and atrophy of collagen fibers [36]. As the tendon degenerates, it can either become hypertrophic or atrophic with a deterioration of its organization and infrastructure. If, or when, rupture occurs, symptoms will often resolve immediately with the formation of a “Popeye” deformity (discussed later). On the contrary, attritional tendon degeneration may occur asymptomatically and painless rupture may occur.
5.3.2 Physical Examination
Evaluation of patients with bicipital tendonitis is often difficult due to vague symptoms and inconsistent physical examination findings. It is therefore critically important to obtain a detailed history to help guide the use of appropriate provocative testing. Some patients may describe a “popping” or “snapping” sensation, especially when internally and externally rotating the humerus in 90° of abduction. Although the most common finding in patients with bicipital tendonitis is bicipital groove tenderness, this type of pain is difficult to differentiate from pain related to the anterosuperior cuff which inserts in the area of the bicipital sheath. Patients who describe a history of anterior shoulder pain that suddenly resolved after a specific incident likely had spontaneous rupture of their LHB tendon.
Both upper extremities of every patient should be inspected for any evidence of asymmetry. Spontaneous rupture of the proximal LHB tendon can result in the classic “Popeye” deformity in which the muscle belly is distally retracted, appearing as distinct bulge in the distal aspect of the humerus just above the elbow (Fig. 5.13). Strength and range of motion should also be recorded for each extremity, particularly noting any discrepancies. Range of motion loss in patients with bicipital groove tenderness is most often associated with concomitant rotator cuff disease (see Chap. 4 for information regarding physical examination of the rotator cuff). Because some biomechanical data suggests that the LHB tendon functions to promote glenohumeral stability, it may also important to perform stability testing in these patients (see Chap. 6). The most important provocative tests traditionally used for the detection of bicipital tendonitis and tearing are presented below.
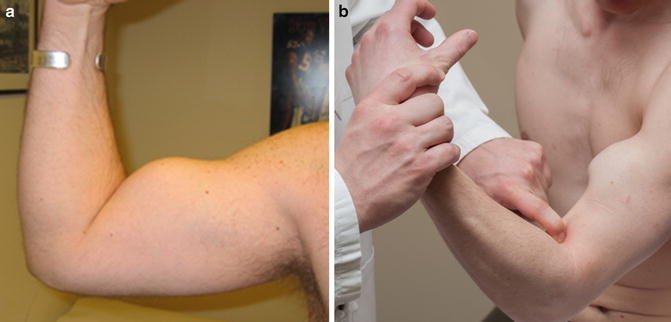
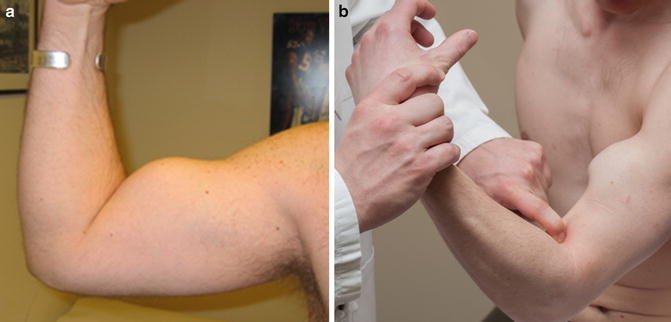
Fig. 5.13
Clinical photograph of Popeye deformity in (a) proximal LHB tendon rupture and (b) distal biceps tendon rupture. Note that proximal LHB tendon rupture results in distal retraction of the muscle belly whereas distal biceps tendon rupture results in proximal retraction of the muscle belly.
5.3.2.1 Palpation
There are several physical examination tests that involve palpation of the LHB tendon on the anterior aspect of the shoulder to detect peri-tendonitis or tearing. However, rather than delving into each individual palpation technique, it is most important to realize that the bicipital groove faces directly anteriorly when the humerus is slightly internally rotated and tenderness with palpation of the groove will typically move laterally as the humerus is externally rotated (Fig. 5.14). Although testing for bicipital tenderness is nonspecific and examiner dependent, when present, it is sometimes helpful to rule out other pathologies within the differential diagnosis. A recent study by Chen et al. [37] found that bicipital tenderness was 57 % sensitive and 74 % specific for the presence of biceps tendonitis after confirmation with ultrasonographic evaluation. Gill et al. [38] reported the diagnostic accuracy of bicipital groove tenderness to detect partial-thickness tears of the LHB tendon; the authors calculated a sensitivity of only 53 % and a specificity of only 54 % using this diagnostic test. Thus, palpation of the bicipital groove should only be used to document the presence or absence of bicipital groove tenderness since the test is not sensitive or specific for the detection of partial-thickness tears.
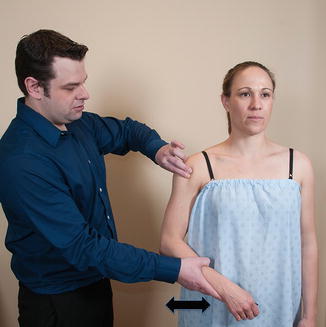
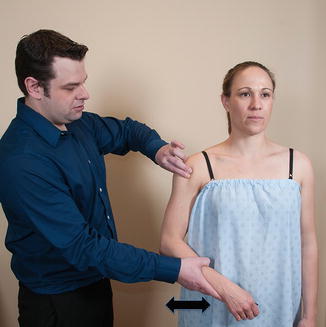
Fig. 5.14
Bicipital groove palpation. The LHB tendon is most easily palpated when the humerus is slightly internally rotated. The examiner can also simultaneously internally and externally rotate the humerus to detect any evidence of subluxation.
5.3.2.2 Speed Test
In the Speed test, the affected arm is placed in a position of 90° forward flexion with the elbow extended and the palm supinated. From this position, the examiner applies a downward force to the forearm while the patient resists (Fig. 5.15). Pain localized to the area of the bicipital groove is a positive test and may indicate the presence of bicipital tendonitis or partial tearing. The specificity for this test is much higher than its sensitivity, thus requiring a combination of historical and other physical findings to make the correct diagnosis (Table 5.1).
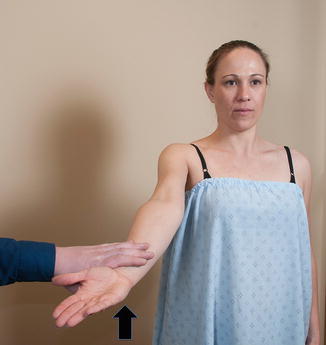
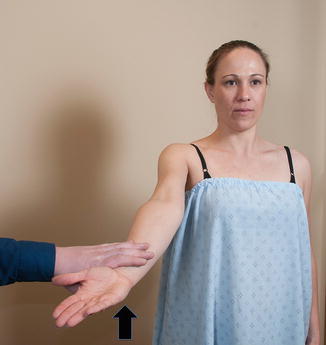
Fig. 5.15
Speed test. The patient is asked to forward flex the humerus to approximately 90° of elevation with the elbow extended and the forearm supinated (palm upward). The examiner then applies a downward pressure to the distal arm as the patient provides resistance.
Table 5.1
Reported diagnostic efficacies of clinical tests used for the detection of LHB tendonitis
Maneuver | Author(s) | Year | Pathology | Diagnostic standard | Sensitivity (%) | Specificity (%) | LR+ | LR− |
---|---|---|---|---|---|---|---|---|
Palpation | Chen et al. [37] | 2011 | Tendonitis | Ultrasound | 57 | 74 | 2.2 | 0.58 |
Gill et al. [38] | 2007 | Partial tear | Arthroscopy | 53 | 54 | 1.15 | 0.87 | |
Lift-off test | Gill et al. [38] | 2007 | Partial tear | Arthroscopy | 28 | 89 | 2.5 | 0.81 |
Jia et al. [39] | 2009 | Tendonitis | Arthroscopy | 28 | 89 | 2.5 | 0.81 | |
Speed test | Gill et al. [38] | 2007 | Partial tear | Arthroscopy | 50 | 67 | 1.51 | 0.75 |
Kibler et al. [40] | 2009 | Tendonitis | Arthroscopy | 54 | 81 | 2.77 | 0.58 | |
Jia et al. [39] | 2009 | Tendonitis | Arthroscopy | 50 | 67 | 1.52 | 0.75 | |
Goyal et al. [41] | 2010 | Tendonitis | Ultrasound | 71 | 85 | 4.6 | 0.34 | |
Salaffi et al. [42] | 2010 | Tendonitis | Ultrasound | 49 | 76 | 2.1 | 0.66 | |
Chen et al. [37] | 2011 | Tendonitis | Ultrasound | 63 | 60 | 1.55 | 0.63 | |
Yergason test | Oh et al. [43] | 2007 | Tendonitis | Ultrasound | 75 | 81 | 4.03 | 0.31 |
Kibler et al. [40] | 2009 | Tendonitis | Arthroscopy | 41 | 79 | 1.94 | 0.74 | |
Chen et al. [37] | 2011 | Tendonitis | Ultrasound | 32 | 78 | 1.47 | 0.87 |
5.3.2.3 Yergason Test
In the Yergason test, the affected arm is placed at the side with the elbow flexed 90°. In patients with bicipital tendonitis or partial tearing, resisted supination of the forearm should produce pain over the anterior aspect of the shoulder localized to the bicipital groove (Fig. 5.16). Similar to the Speed test, the specificity for the Yergason test is higher than its sensitivity thus requiring further information in order to make the correct diagnosis (see Table 5.1).
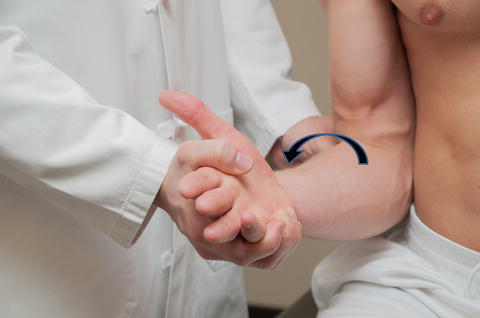
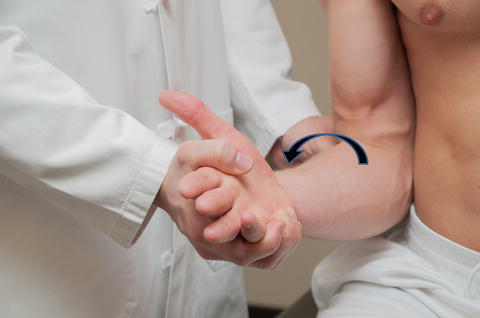
Fig. 5.16
Yergason test. With the arm at the side and the elbow flexed to 90°, the patient attempts to supinate the forearm against resistance provided by the examiner.
5.3.2.4 Lift-Off Test
Testing for rotator cuff function, especially that of the subscapularis, can also provide clues regarding the status of the proximal LHB tendon as it passes through the bicipital groove. Tearing of the subscapularis may also involve tearing of the bicipital sheath and the LHB tendon itself. Gill et al. [38] noted that the lift-off test, which tests the strength of the subscapularis, had a sensitivity of 28 % and a specificity of 89 % for the detection of partial-thickness tears of the LHB tendon. The bear-hug test can also be used to detect biceps tendinopathy with a reported sensitivity of 79 % and a specificity of 60 % [40]. See Chap. 4 for details regarding physical examination for the detection of subscapularis pathology.
5.3.2.5 Biceps Entrapment Sign
The hourglass biceps, first described by Boileau et al. [54] in 2004, occurs when the LHB tendon in the proximity of the bicipital sheath becomes inflamed and swells to a diameter that exceed that of the bicipital groove, thus preventing the LHB tendon from gliding within the sheath as shoulder motion is initiated (Fig. 5.17). Arthroscopically, the defect resembles an hourglass-shape that becomes entrapped proximal to the bicipital sheath which typically limits forward flexion capacity.
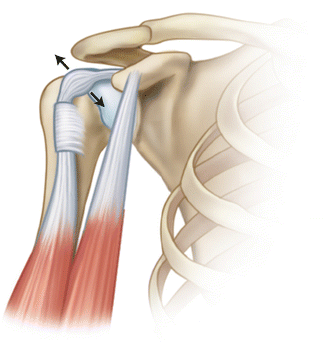
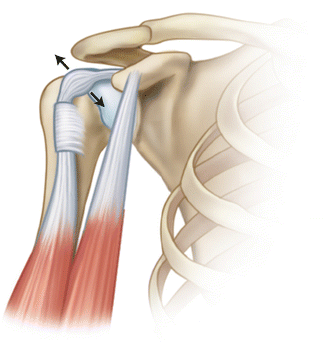
Fig. 5.17
Illustration depicting an hourglass biceps which cannot slide efficiently into and out of the bicipital sheath with arm elevation.
Boileau et al. [54] originally reported this entity in a series of 21 patients with a “hypertrophic intra-articular portion of the LHB tendon,” all of which were associated with rotator cuff tearing. The authors noted that a 10–20° loss of passive forward flexion in the presence of bicipital groove tenderness were the most common physical findings in this group of patients. Others have suggested that both active and passive motion should be restricted where an attempt to increase the forward flexion angle results in increased shoulder pain [55]. Because this examination finding has not been formally validated, imaging studies such as those including ultrasonic evaluation [56], remain important for identification of these lesions in the clinical setting.
5.4 LHB Tendon Instability
Instability of the LHB tendon is most often associated with tearing of the subscapularis tendon, coracohumeral ligament, and the SGHL, all of which are components of the BRP (Fig. 5.18) [15, 57, 58]. Although several authors have proposed different classification systems to describe LHB instability [14, 57, 59], most clinicians still categorize this pathology into one of two types primarily based on the system developed by Walch et al. [59] in 1998: (1) subluxation out of the groove, (2) dislocation out of the groove, and (3) intra-articular dislocation. In general, most episodes of instability occur with the LHB translating medially over the lesser tuberosity. Lateral instability of the LHB tendon is rare.
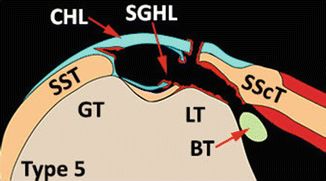
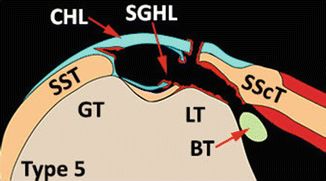
Fig. 5.18
Illustration showing medial biceps subluxation as a result of tearing of the subscapularis tendon and the SGHL. Tearing of the coracohumeral ligament can also be seen. Source: Stadnick ME. Pathology of the long head of the biceps tendon. Radsource Web Clinics. February 2014. http://radsource.us/pathology-of-the-long-head-of-the-biceps-tendon/.
5.4.1 Pathogenesis
The pathogenesis of pulley lesions that lead to medial subluxation or dislocation of the LHB tendon has not been completely elucidated. Some have suggested that asymmetric loading of the LHB in positions of abduction and external rotation may be responsible for lesions of the BRP. However, in a biplane fluoroscopic study in cadavers performed by Braun et al. [16], the investigators demonstrated high shear-force vectors across the BRP in both the neutral and in internal rotation positions with or without forward flexion. Currently, there is insufficient data to suggest a specific pathomechanism responsible for the development of pulley lesions.
5.4.2 Physical Examination
While there are some physical examination maneuvers that can be used to evaluate instability of the LHB tendon [25, 60, 61], none of these methods have been formally validated. Typically, the clinician will palpate the bicipital groove while simultaneously internally and externally rotating the humerus (see Fig. 5.14). Painful “clicking” or “popping” occurs as the LHB tendon translates over the lesser tuberosity. Complete dislocation of the LHB tendon out of the bicipital groove indicates a high probability of concomitant full- or partial-thickness tearing of the upper 1/3 of the subscapularis tendon and/or the anterior aspect of the supraspinatus tendon [59, 61]. Therefore, full examination of the anterosuperior rotator cuff is necessary in cases where instability of the LHB is suspected (see Chap. 4).
5.4.2.1 Arm Wrestle Test
Recently, we have begun using an arm wrestle test to detect medial or lateral subluxation of the LHB tendon. In this test, the clinician first grasps the patient’s hand in a standard “arm wrestle grip.” With the elbow flexed approximately 90° and the shoulder in neutral rotation, the patient is asked to flex the elbow and supinate the forearm against resistance with perturbations (Fig. 5.19). A positive test occurs when pain or other mechanical symptoms are reproduced as the LHB tendon subluxates out of the bicipital groove. Although we are unaware of any other previous studies that utilize this test, we have anecdotally found it quite useful for the detection of medial or lateral biceps subluxation.
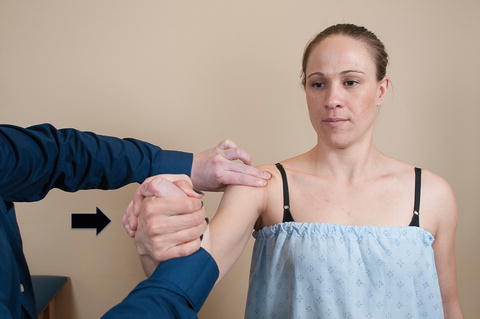
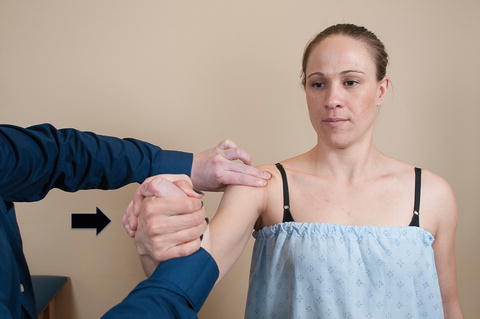
Fig. 5.19
Arm wrestle test. With the elbow flexed to 90° in neutral rotation, the examiner grasps the hand of the patient in an “arm wrestle grip.” The patient then attempts to flex and supinate the forearm against resistance (perturbations) provided by the examiner.
5.5 SLAP Tears
In 1985, Andrews et al. [62] were the first investigators to describe lesions to the proximal biceps tendon with involvement of the superior aspect of the glenoid labrum. Later, Snyder et al. [63] coined the term “SLAP” tear due to the anterior to posterior direction of labral tearing (Superior Labral Anterior to Posterior; Fig. 5.20). Snyder et al. [63] also classified SLAP tears into four groups which were later supplemented by three additional groups (types I–VII) (Fig. 5.21). The incidence of non-type I SLAP (non-degenerative) tears ranges from 3.4 to 26 % in patients who present with shoulder pain [47, 63–68]; however, these figures may be increased in high-level throwing athletes. SLAP tears can occur in isolation or in conjunction with other shoulder pathologies such as rotator cuff tears, biceps tendon pathology, and/or glenohumeral instability [64, 67, 69].
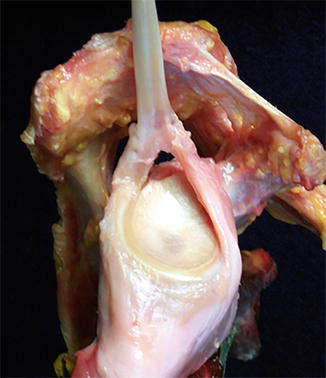
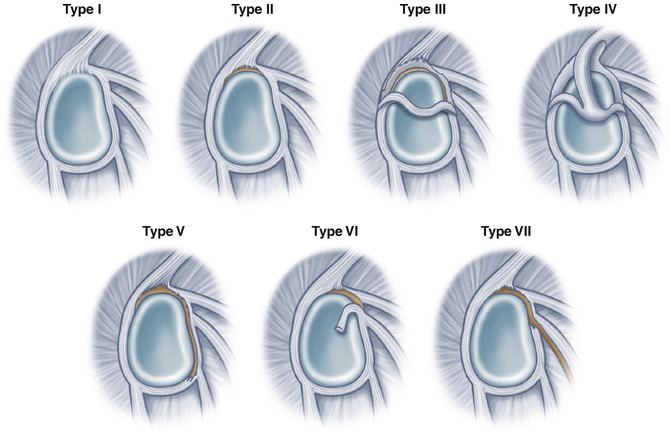
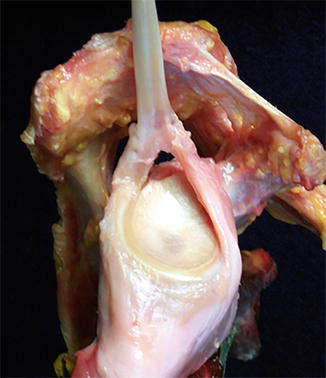
Fig. 5.20
Cadaveric photograph depicting a SLAP tear. Note that the biceps-labral complex is elevated away from the glenoid both anteriorly and posteriorly.
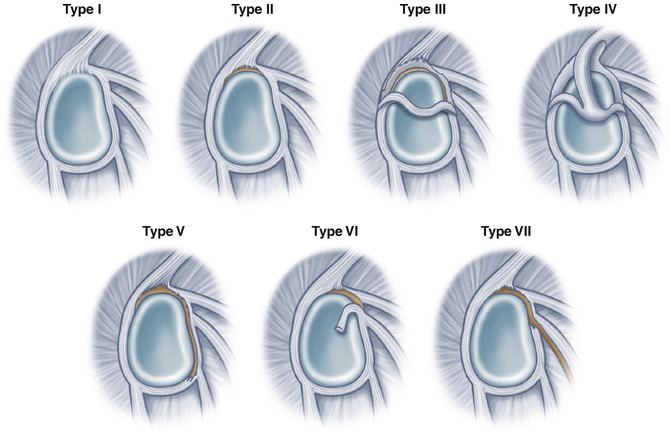
Fig. 5.21
SLAP tear classification system developed by Snyder et al. [63] and later modified by Maffett et al. [64] Type 1 = degenerative fraying; Type II = extension into biceps tendon; Type III = bucket-handle tear with intact biceps; Type IV = bucket-handle tear with biceps extension; Type V = SLAP tear combined with Bankart lesion; Type VI = unstable flap tear Type VII = extension into middle glenohumeral ligament (MGHL).
5.5.1 Pathogenesis
The precise pathomechanism behind the development of SLAP tears has been debated since its first description in 1985. Investigators most commonly cite forceful traction loads, forceful compression loads, and overhead sporting activities as the most common causes of SLAP tears. A forceful traction load to the biceps tendon may create a defect at the superior labrum resulting in pain and dysfunction [10, 70]. Clavert et al. [70] and Bey et al. [71] found that forward flexion and inferior traction, respectively, facilitated the development of SLAP lesions in separate cadaveric models. A forceful compression load may trap the biceps-labrum complex between the humeral head and the superior glenoid rim which may produce a mechanical shearing effect (or “grinding,” as suggested by Snyder et al. [63, 67]) resulting in a tear of the superior labrum. Repetitive traction from participation in overhead throwing sports such as baseball and softball also commonly result in tearing of the superior labrum.
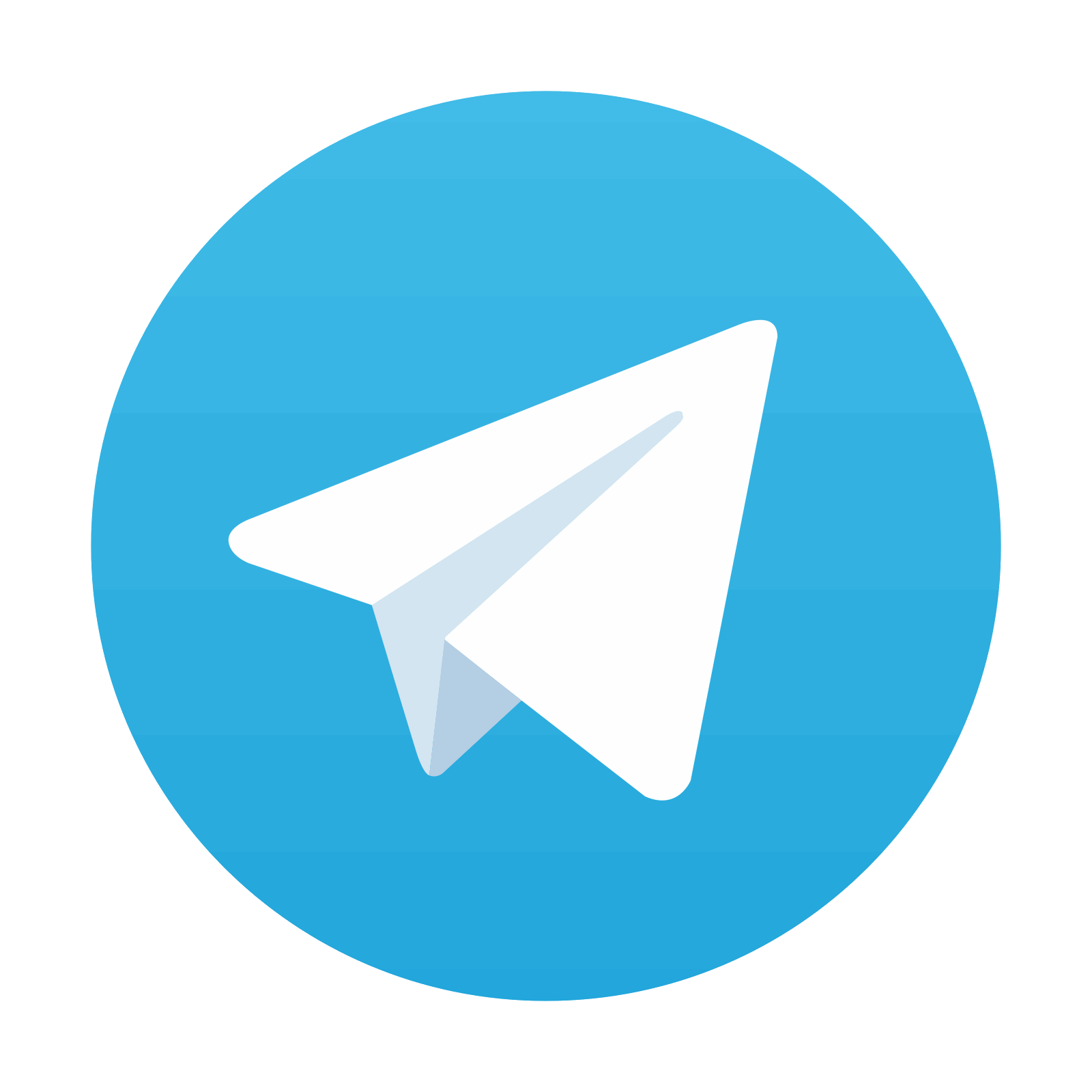
Stay updated, free articles. Join our Telegram channel

Full access? Get Clinical Tree
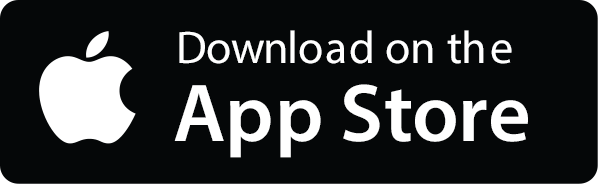
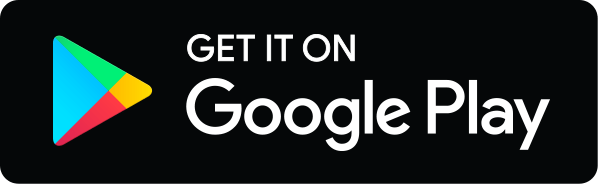