Dislocations of the Foot and Ankle
Graham A. Hamilton
Lawrence A. Ford
Johanna-Marie Richey
Joint dislocations represent an injury that results in the loss of integrity of the anatomic structures that maintain joint alignment and congruity. With the insult, severe soft tissue injury results, and the normal position of the joint or other body part is disturbed. In the absence of fracture, dislocations of the foot and ankle are relatively rare. The anatomy of the foot and ankle is the principal reason for this. Strong ligaments that control motion in the foot and ankle also impart tremendous stability.
The type of dislocation injury can often be inferred from a complete history. The diagnostic approach should begin by establishing the magnitude and direction of the forces applied to the anatomic structures in question. Such forces may vary from high-velocity motor vehicle accidents to simple trivial twisting mechanisms. Application of the force can be direct, such as contact of the joint with the dashboard of a motor vehicle, or indirect, such as rotational forces applied through the foot and dissipation through ankle ligaments. Accurate knowledge of the magnitude and direction of forces applied and the position of the joint in question at impact, coupled with the understanding of anatomy, often leads to a suspected diagnosis or pattern of injury before the physical examination is started.
Adequate assessment and treatment of the entire patient and the entire injured limb generally have a higher priority than assessment and treatment of the foot or ankle injury. However, significant permanent morbidity, including amputation, can be prevented by adequate care of a foot or ankle injury. After lifesaving and limb-saving measures have been initiated, the foot or ankle injury must not be neglected. Marked deformity, usually resulting from a dislocation or fracture dislocation, poses a threat to the local perfusion of skin and soft tissues stretched over the deformed bony prominences. It promotes local swelling and may also interfere with distal blood circulation. In addition, it is often quite painful and may injure articular cartilage impinged on by a sharp bony edge. Therefore, provisional reduction to improve local perfusion and prevent further injury is urgent. Application of a molded splint should follow.
Priorities for the dislocated joint include prompt diagnosis, assessment, and treatment. A logical treatment protocol should be implemented. Adequate blood flow to the injured limb must be ensured. This is usually achieved with provisional reduction of marked deformity or dislocation. Any soft tissue compromise needs to be aggressively treated. Precise reduction of skeletal deformity then needs to be established, which must be maintained throughout healing. If the injury has affected tendons or nerves, restoration and repair are required. The final two phases of treatment include aggressive rehabilitation and identification and treatment of any complications that develop.
ANKLE DISLOCATIONS
HISTORY
The ankle is one of the most frequently injured joints. Most of these injuries are ligament sprains, fractures, or fracture dislocations. Pure dislocations of the ankle are uncommon. The literature is filled with case presentations and small series of dislocations of the ankle joint. There are however, no articles on randomized treatment protocols or prospective analysis. Most of our experience from the literature is anecdotal and retrospective (1,2,3,4,5,6,7,8,9,10,11 and 12). Ankle dislocations like all dislocations can be divided into dislocation with fracture and dislocation without fracture.
ANATOMY
An understanding of the anatomical restraints of the ankle joint provides insight into how pure dislocations can occur. Inman (11) described ankle anatomy and motion in a classic work. The talus is not flat but rather has a dual-dome upper surface. These two shallow convex curves articulate with a matching tibial distal joint surface. The talus is asymmetric; it is wider anteriorly and has different articulating surfaces with the tibia and the fibula. The distal tibia also narrows posteriorly, and the posterior malleolus does articulate with the talus and its trigonal process. The lateral and medial malleoli provide stability, which is important for both tilt and rotation, and their articulation with the talus constitutes an important amount of joint surface area.
The ankle ligaments form complex checkreins. Laterally, the ligamentous complex consists of three distinct thickenings of the joint capsule. The anterior talofibular (ATF) ligament travels from the anterior aspect of the fibula to the lateral aspect of the talar neck. This band restrains anterior displacement, inversion, and internal rotation of the joint (14,15). The calcaneofibular (CF) ligament lies deep to the peroneal tendons from the distal pole of the fibula to the calcaneus; this extracapsular ligament restrains the ankle and subtalar joint from eversion stresses. The posterior talofibular ligament is a short, stout ligament connecting the posterior process of the talus to the fibula. This ligament binds so tightly that it often causes a posterior malleolus avulsion fracture with enough strain.
Medially, the deltoid ligament is composed of two layers. The superficial deltoid originates on the anterior colliculus of the medial malleolus and inserts on the calcaneus, navicular, and talus and helps to resist hindfoot eversion (16). Laboratory studies demonstrated that sectioning the superficial deltoid ligament leads to a 43% decrease in contact area under the joint (17). The deep deltoid is thought to be the primary medial stabilizer of the ankle. Originating from the deep surface of the
posterior colliculus and inserting on the talus just posterior to the medial facet, the deep deltoid ligament accounts for up to 57% of the restraint to external rotation of the talus within the mortise (18).
posterior colliculus and inserting on the talus just posterior to the medial facet, the deep deltoid ligament accounts for up to 57% of the restraint to external rotation of the talus within the mortise (18).
Recent studies further delineate the deltoid ligament complex, finding the tibiocalcaneal ligament the longest and thickest of these structures (19). The deltoid, when it ruptures, often does so with a complex tear that often defies easy surgical reapproximation. Most sources have documented that acute repair of the deltoid is not necessary but that the ligament will heal with appropriate bony stability (5).
Superior to the ankle joint, but intimately involved with many ankle injuries, the syndesmotic ligament complex holds the fibula in close proximity to the tibia. The syndesmosis consists of four parts: the anterior inferior tibiofibular ligament, the posterior inferior tibiofibular ligament, the interosseous tibiofibular ligament, and the transverse inferior tibiofibular ligament. The distal syndesmosis acts as a bridge in the weight transfer from the tibia to the fibula (20). The tibial and fibular orientation provides a constrained “box-like” receptacle for the relatively square talus. This creates a very stable “box within a box” alignment as the ankle is load bearing and in neutral position. However, because the talus is narrower posteriorly than anteriorly, as the ankle rotates into more plantarflexion, the bony stability is less constraining. With this plantarflexion, the talus translates slightly anterior, “unlocking” itself from the mortise. So with the ankle in a neutral position, ankle dislocation is extremely rare. However, with progressive plantarflexion or extreme dorsiflexion, dislocation can occur.
MECHANISM OF INJURY
Pure ankle dislocations are usually a result of a high-energy mechanism. Review of the literature shows pure dislocation without fracture is most prevalent in road accidents (40%), particularly motorcycle accidents (33%). The second most common cause is sports trauma (35%), particularly in sports in which jumping is a fundamental component such as volleyball (13%) and basketball (8%) (21). Motor vehicle accidents often involve a severe axial load with the foot and ankle usually in maximal plantarflexed and inverted position. This can result in a posteromedial dislocation as a result of the force through the foot at impact. Alternately if an axial load is applied with the foot and ankle externally rotated and in maximal dorsiflexion, an anterolateral dislocation would most likely occur. Given the high-energy nature of these injury patterns, open injuries are also common (33%). Neurovascular compromise is frequently associated with posteromedial dislocation. Posteromedial dislocation can also be seen in sports injuries, in which an axial load is applied to the foot while it is plantarflexed and inverted and about to launch an athlete in a jump.
Most ankle dislocations with fracture are part of a malleolar injury. (Figs. 102.1 and 102.2). Pure dislocation of the ankle joint without fracture is rare. The ankle does not have discrete stabilizing structures anteriorly and posteriorly. Medially and laterally, there are strong stabilizing structures: malleoli and collateral ligaments. As a consequence, the talus dislocates either posteriorly or anteriorly in pure dislocation without fracture (Fig. 102.3).
CLINICAL EVALUATION
Evaluation begins once the patient is hemodynamically stable by assessing the skin envelope for any compromise. According to Kelly et al, about one-third of these injures are open and should be managed according to open injury protocol. Open dislocation carries with it the associated risk of infection and avascular necrosis (AVN). Skin lacerations are lateral with
posteromedial dislocations and medial with anterolateral dislocations (20). In the case of a closed injury, there is usually gross deformity of the lower extremity. The skin is likely to be tented and blanched over the malleoli. In posteromedial dislocations, the foot is plantarflexed and supinated and positioned medial and posterior to the leg. Neurovascular injury is an obvious concern and needs to be assessed expeditiously. The same is true for anterolateral ankle dislocation, as the foot is dislocated everted and lateral to the leg. Prompt relocation is of paramount importance. In the posteromedial dislocation, fragment pressure from within, usually the anterior aspect of the distal tibia, can cause tissue infarction and necrosis. Relocation is necessary to remove the “inside-out” pressure on the tissue envelope (Fig. 102.4).
posteromedial dislocations and medial with anterolateral dislocations (20). In the case of a closed injury, there is usually gross deformity of the lower extremity. The skin is likely to be tented and blanched over the malleoli. In posteromedial dislocations, the foot is plantarflexed and supinated and positioned medial and posterior to the leg. Neurovascular injury is an obvious concern and needs to be assessed expeditiously. The same is true for anterolateral ankle dislocation, as the foot is dislocated everted and lateral to the leg. Prompt relocation is of paramount importance. In the posteromedial dislocation, fragment pressure from within, usually the anterior aspect of the distal tibia, can cause tissue infarction and necrosis. Relocation is necessary to remove the “inside-out” pressure on the tissue envelope (Fig. 102.4).
![]() Figure 102.1 A,B: Lateral and AP radiographs of a bimalleolar ankle fracture with posterior dislocation. C: Lateral postreduction radiograph. (Continues on next page) |
The skin also needs to be evaluated for blisters. They are common with these injuries and represent a cleavage between the dermis and epidermis. Two types can develop: blood filled and fluid filled. Blood-filled blisters represent a complete
separation of the epidermis from dermis, and the fluid-filled blisters, partial separation (Fig. 102.5). Severe blistering of the skin develops from an ankle fracture dislocation. Validated by the work of Giordano et al, if internal fixation is planned and exposure requires passage through a blister, it is prudent to decompress the blister and wait until re-epithelialization occurs (21). Incision through a blood-filled blister carries with it the increased risk of infection.
separation of the epidermis from dermis, and the fluid-filled blisters, partial separation (Fig. 102.5). Severe blistering of the skin develops from an ankle fracture dislocation. Validated by the work of Giordano et al, if internal fixation is planned and exposure requires passage through a blister, it is prudent to decompress the blister and wait until re-epithelialization occurs (21). Incision through a blood-filled blister carries with it the increased risk of infection.
![]() Figure 102.3 A: Lateral radiograph of a posterior ankle dislocation without fracture. B: Pure anterior dislocation of the ankle. |
Clinical evaluation should cover palpation of the malleoli and collateral ligaments. The entire length of the fibula should be examined to rule out a Maisonneuve fracture. The syndesmosis should be checked with a medial, lateral squeeze about 5 cm superior to the joint to assess syndesmotic injury. Compartments of the foot and leg should be soft, and a muscle inventory should be performed, including a Thompson test to assess the integrity of the Achilles and extrinsic tendons. The foot should also be visually inspected and palpated. In the polytrauma patient who may be unresponsive, extra diligence must be taken to avoid overlooking pertinent findings on examination.
RADIOGRAPHIC EVALUATION
Standard anteroposterior (AP), lateral, and mortise views of the ankle should be obtained prior to reduction, but not at the expense of a timely reduction. If there is gross deformity, reduction of the foot under the leg in critical. High fibular tenderness warrants an x-ray up to the knee joint, and foot radiographs should be obtained if a positive clinical exam was noted. Postreduction views are also necessary to document concentric reduction (see Fig. 102.1). Avulsion and impaction fractures should be identified on injury and postreduction radiographs, and, if identified, computed tomography (CT) could help determine whether the fragments require fixation or removal.
TREATMENT
Treatment focuses on concentric reduction of the ankle joint, surgical management of open wounds, and repair of any injured tendons, nerves, and arteries as needed. Closed reduction is usually successful and generally affords a satisfactory result with a relatively low risk of posttraumatic arthritis and functional disability. This is usually accomplished in the emergency department under conscious sedation. The following initial sequence helps facilitate this process: (a) adequate anesthesia is induced with conscious sedation of the patient, (b) the hip and knee are flexed to relax the pull of the gastrocnemius and held for countertraction (lying the patient supine on a gurney with the leg hanging off the end usually facilitates this), and (c) using a Quigley maneuver, the foot is translated anteriorly or posteriorly thereby relocating the talus beneath the tibia. It has been the author’s experience that in patients that have sustained an anterolateral dislocation, the propensity for redislocation or subluxation is high. This is due to the rather thin anterior joint capsule that has been disrupted. If instability is noted after reduction, then percutaneous pinning of the tibiotalar joint with smooth Steinmann pins is recommended (22). Since most wounds are lacerations, they can usually be loosely reapproximated. If tension-free closure cannot be obtained or wound edges have questionable viability, then delayed closure is recommended, in approximately 3 to 5 days from the primary relocation. As a rule, definitive wound closure, either primarily or with a flap, should be obtained within the first week. Definitive treatment for ligamentous injury requires immobilization. Closed ankle dislocations without fracture can usually be treated without surgical repair. Immobilization is recommended for 4 weeks in a short leg non-weight-bearing cast. At this point, the patient can be progressed to a walking boot and rehabilitation begun with physical therapy. In the case of the open dislocation, after appropriate débridement, primary ligament repair can be performed if grossly unstable. The ankle is immobilized in a short leg cast until the wound is healed. Once this has occurred, the patient can be progressed to a rehabilitation program.
Open Dislocation (Extruded Talus)
Occasionally, there can be a complete dislocation of the talus from the ankle joint, subtalar, and talonavicular joints. These extruded talus injuries are usually open, and given the open nature of these injuries, carry all the risks associated with them, including sepsis, osteonecrosis, and potentially amputation (Fig. 102.6).
![]() Figure 102.6 Clinical pictures of an extruded talus of a 55-yearold male who fell 10 feet off a ladder. A-C: The talus is still partially attached to the tibia by the deltoid ligament. |
![]() Figure 102.6 (Continued) D: Immediate reimplantation and relocation. E-G: Stabilization of the talonavicular joint and ankle joint with percutaneous smooth Steinmann pins. |
The options for the treating foot and ankle surgeon are (a) talus reimplantation, (b) reimplantation and arthrodesis, (c) talectomy, and (d) talectomy and tibiocalcaneal arthrodesis. Historically, Coltart performed a talectomy on seven out of nine patients with these injuries (23). More recently, Marsh and Saltzman reported on their findings of 18 open talar fracture dislocations or total dislocations of the talus. They reported an infection rate of 38% (7/18 patients) and given this high infection rate, advocated talar excision and early tibiocalcaneal fusion (24). Smith et al reported in 2006 on 19 extruded tali, some completely devoid of any soft tissue attachments. In all cases, the extruded tali were reimplanted and salvaged after lavage. Protocol included, if still partially attached, lavage with 2 to 3 L of isotonic normal saline and then reimplantation.
A completely detached talus was transported with the patient to the operating room with the talus in bacitracin solution. Once in the operating room, it was placed in two or three bacitracin solutions and then gently scrubbed before reimplantation. With this protocol, an infection rate of 10% was reported and no patients underwent delayed amputation (25).
A completely detached talus was transported with the patient to the operating room with the talus in bacitracin solution. Once in the operating room, it was placed in two or three bacitracin solutions and then gently scrubbed before reimplantation. With this protocol, an infection rate of 10% was reported and no patients underwent delayed amputation (25).
Open fracture parenteral antibiotic protocol is recommended for these injuries and is based on size of the open wound and wound contamination (26). This usually involved a first-generation cephalosporin, coupled with an aminoglycoside for larger open injuries and penicillin for gross or farm contamination. Broad-spectrum antibiotics are usually given for 1 to 5 days (27). Antibiotics are started on arrival of the patient and continued usually for 48 hours after wound closure. It must, however, be remembered that in the presence of more powerful antibiotics, removal of all unhealthy tissue is the single most important factor in the attainment of a good result after an open dislocation with or without fracture (26).
Given the evidence, reimplantation and salvage of an extruded talus seems to be the most acceptable option, and this has been the author’s experience. Each scenario, however, should be taken on a case-by-case basis and the patient counseled on the severity of the injury and possibility of rapid removal if infection arises and the possibility of amputation (25).
PROGNOSIS AND LONG-TERM FOLLOW-UP
Prognosis after ankle dislocation without neurovascular injury is good. Stiffness is the most common complaint. Complications include wound problems, infection, recurrent instability, AVN, posttraumatic arthrosis, and neurovascular compromise.
Wound problems and skin slough or infarction in the closed dislocation are generally rare and preventable. Prompt reduction to decrease tenting on the skin can avoid inward outward pressure and potential skin infarction. If skin slough and potential necrosis does occur, then débridement of the affected area followed by appropriate wound care is indicated. Negative pressure wound therapy can also be utilized and highly effective (see Fig. 102.4C-I). Open injuries offer a whole new set of challenges. If closure does not seem feasible or if the soft tissue on initial inspection is clearly nonviable, early involvement of a plastic surgeon is advisable. Infection is a potentially serious complication. Serial débridement and culture-specific intravenous (IV) antibiotics are necessary, and foot reconstruction or amputation may eventually be required. A recent series on total talar extrusion showed a lower infection rate (10%) with no patients requiring delayed amputation (25).
Instability is an infrequent problem with roughly 10% of patients complaining of this problem. In the chronic scenario, orthotics or bracing should be a first-line therapy to stabilize the ankle. Refractory cases will require ligament repair or tenodesis augmentation.
AVN is a rare complication of ankle dislocation (7). AVN has also been shown to increase in open injuries and with talus fracture. Smith et al commented on collapse due to osteonecrosis on 13 extruded tali that had greater than 1-year follow-up. In the presence of major fracture, all showed some collapse (25).
TIPS, PEARLS, AND PITFALLS
Pure dislocation of the ankle joint without fracture is rare.
Most ankle dislocations are part of a malleolar injury. The ankle does not have discrete stabilizing structures anteriorly and posteriorly, which makes it vulnerable to dislocation in these directions.
Prompt relocation is of paramount importance.
Remember to flex the knee to remove the deforming force of the gastrocnemius.
Quigley maneuver can be very helpful for rotational mechanisms.
Anterior and posterior dislocations with large posterior malleolar fractures can be very unstable and may require percutaneous pinning with Steinmann pins
Treatment focuses on concentric reduction of the ankle joint, surgical management of open wounds, and repair of any injured tendons, nerves, and arteries as needed.
Respect the soft tissue envelope.
Definitive wound/laceration closure, either primarily or with a flap, should be obtained within the first week.
Removal of all unhealthy tissue is the single most important factor in the attainment of a good result after an open dislocation with or without fracture.
SUBTALAR DISLOCATIONS
The simultaneous dislocation of the subtalar and talonavicular joints defines subtalar dislocations. The calcaneocuboid and ankle joints remain congruent (28,29). As many as half the cases contain small avulsion or impaction fractures of the talar head, rearfoot tarsals, malleoli, and the fifth metatarsal styloid with these injuries (29). Subtalar dislocations either open or closed are rare injuries. About 1% to 2% of all dislocations involve the subtalar joint (30). Men are three to ten times more likely than women to sustain this injury (29,31,32 and 33), which typically occurs in the fourth decade (32,34).
HISTORY
DuFaurest and Judey first reported subtalar dislocation in 1811 (35,36). In 1853, Broca was the first to classify these injuries as medial, lateral, and posterior (37). This classification was modified by Malgaigne to include the rare anterior dislocation of the subtalar joint (38). This classification currently remains the most recognized and utilized.
Other terms for these injuries have been used historically. These include subastragaloid (33), subastragalar (39), peritalar (40), and talocalcaneonavicular (41).
CLASSIFICATION
Broca’s classification modified by Malgaigne remains the most widely used today (37). The classification scheme is purely anatomic, describing the foot position in relation to the leg. The subtalar joint can dislocate in any direction, most commonly in a medial (80%) and, to a lesser extent, lateral (15%) direction. These injuries can have a posterior (2.5%) and anterior
(1%) component to them with a much lower frequency (34) (Figs. 102.7 and 102.8; see Fig. 102.6A-C as an example of an open lateral subtalar dislocation).
(1%) component to them with a much lower frequency (34) (Figs. 102.7 and 102.8; see Fig. 102.6A-C as an example of an open lateral subtalar dislocation).
ANATOMY
The subtalar joint or “false ankle joint” combines three articular facets: anterior, middle, and posterior. It is through these articular facets that the talus inverts and everts on the calcaneus. Supporting soft tissue structures stabilizes the joint. The main ligamentous support involves the interosseous talocalcaneal ligament within the sinus tarsi and the joint capsule around each facet. These are further strengthened medially by the superficial deltoid ligament and medial talocalcaneal ligament and laterally by the CF ligament, the ATF ligament, the cervical ligament, and the lateral talocalcaneal ligament.
Cadaveric studies have shown that tearing of all three ligaments (CF, superficial deltoid, and talocalcaneal ligaments) has to occur to allow for the typical coronal plane dislocation either medially or laterally. The calcaneonavicular ligament (spring ligament) remains uninjured because of its increased tensile strength (45).
Cadaveric studies have shown that tearing of all three ligaments (CF, superficial deltoid, and talocalcaneal ligaments) has to occur to allow for the typical coronal plane dislocation either medially or laterally. The calcaneonavicular ligament (spring ligament) remains uninjured because of its increased tensile strength (45).
MECHANISM OF INJURY
Subtalar dislocation can occur as a result of both high- and lowenergy mechanisms. The majority (between 50% and 80%) are caused by high-energy mechanisms such as a motor vehicle accident or a fall from a height (46). The remainder occurs interestingly through rather trivial means, such as an inversion foot injury. As a general rule, poorer outcomes result from higher-energy injuries and lateral or open dislocations (31).
Medial subtalar dislocations result from a plantarflexion inversion moment around the sustentaculum that initially disrupts the talonavicular joint before the talocalcaneal joint. Given fewer anatomic constraints against this direction, these injuries can result from the simple low-energy inversion sprain. In this case, the foot clinically looks like an acquired clubfoot and is inverted, adducted, and plantarflexed. There may be a lateral foot wound or tenting to the skin (Fig. 102.7A and B) (47).
Lateral subtalar dislocations have the opposite deformity. Dislocation occurs with forceful eversion of the plantarflexed foot. In this scenario, the anterolateral talus acts as a fulcrum about which the anterior process of the talus pivots, resulting in sequential lateral dislocation of the talonavicular joint and subtalar joints (44,47). Given the anatomy of the subtalar joint, lateral dislocations require considerably more force than medial dislocations, therefore increasing the severity of soft tissue damage, and more than half are associated with open wounds (Fig. 102.6A-C) (28,31,32). The foot in this case clinically looks much like a flatfoot.
Finally, severe plantarflexion forces have caused the few documented true posterior subtalar dislocations (48,49 and 50). Pure anterior dislocations occur when anterior traction on the foot first tears the talocalcaneal interosseous ligament, followed by the medial and lateral ligaments. The low probability of exposure to this distractive type of force makes anterior dislocation very rare (48).
CLINICAL EVALUATION
Medial subtalar dislocations occur with the greatest frequency (80%) (34). Typically, these injuries cause marked deformity of the foot and can mimic an ankle dislocation at initial evaluation or before x-rays establish its true nature (51). In this case, the foot looks like an acquired clubfoot (Fig. 102.7A). In most cases, the skin envelope is intact, but tented over the lateral malleolus and prominent talar head laterally. Forty percent of these dislocations are associated with an open injury (52).
Lateral subtalar dislocations occur less frequently (17%) (34). Given the added soft tissue constraints stabilizing the joint, these injuries are usually the result of high-energy mechanisms. With the added force, greater than 50% are open (29,53,54). Clinically, the foot is abducted and pronated and looks much like a severe flatfoot. The skin if still intact is tented medially over the bulging talar head and medial malleolus.
Regardless of dislocation type, a precise neurovascular examination should be performed to document circulatory status of the foot as well as a thorough sensory examination. The skin envelope needs to also be carefully examined for openings.
RADIOGRAPHIC EVALUATION
Standard AP lateral and oblique radiographs of the foot and ankle should be obtained before attempts at reduction to assess for any associated fracture and aid in diagnosis and reduction. The key element to accurate radiographic diagnosis is the relationship of the talar head to the navicular, which is normally congruent on all views.
Lateral subtalar dislocations are identified by lateral displacement of the navicular relative to the talar head and of the calcaneus beneath the talus. The calcaneocuboid joint remains intact. Associated fractures commonly involve the cuboid, anterior calcaneal process, lateral talar process, and the lateral malleolus (45,41,55). Medial subtalar dislocation results in plantar and medial dislocation of the navicular relative to the talar head and medial dislocation of the calcaneus relative to the talus. As with the lateral dislocations, the calcaneocuboid articulation remains intact. Associated fractures include impaction of the dorsomedial talar head and lateral navicular and fracture of the posterior tubercles of the talus ((41,57,58,59 and 60)(Fig. 102.9).
In certain cases, these can be obvious on the prereduction radiographs. However, fractures may be more obvious after relocation has occurred (Fig. 102.9F). As mentioned, the key to relocation is the talonavicular joint. Fracture impaction here can block closed reduction and should therefore be recognized (28,30,45,60).
After relocation, which can usually be seen, felt, and heard (29,45), AP and lateral radiographs of the foot and ankle are essential to confirm a successful reduction and to demonstrate restoration of foot alignment and reduction of the talonavicular and subtalar joints. Another view that can be helpful in assessing the reduction of the subtalar joint is a Brodén view (Fig. 102.10). Once relocation has been assessed, the films should be scrutinized for impaction or avulsion fractures. Computed tomography (CT) after reduction is strongly recommended to identify these fractures more accurately and treat them appropriately either with removal or internal fixation. Osteochondral fractures (OCFs) are a common occurrence after subtalar dislocation. Reports have shown that OCFs occur in 50% to 71% of all subtalar dislocations and nearly all involve the subtalar joint. OCFs are less common in medial dislocation, occurring in 12% to 38% of injuries. A 100% incidence has been reported with lateral dislocations (28,29,32,34,61). A poorer prognosis is to be expected with the identification of an OCF, and patients should be counseled accordingly about the need for future surgery (29,33,40).
TREATMENT
Early reduction is essential in avoiding skin necrosis and circulatory compromise to the injured limb (40,23,62,63).
Closed Reduction
The important point in understanding the nature of subtalar dislocations and thus how best to treat it lies in the talonavicular relationship and the direction of its dislocation. It is the talonavicular joint relationship that guides relocation. The relocation
maneuver depends on the direction of dislocation. The following initial sequence helps facilitate this process: (a) adequate anesthesia is induced with conscious sedation of the patient, (b) the hip and knee are flexed to relax the pull of the gastrocnemius and held for countertraction (lying the patient supine on a gurney with the leg hanging off the end usually facilitates this), (c) the heel is manually distracted longitudinally, and (d) the deformity is initially exaggerated to “unlock” the foot (41,42).
maneuver depends on the direction of dislocation. The following initial sequence helps facilitate this process: (a) adequate anesthesia is induced with conscious sedation of the patient, (b) the hip and knee are flexed to relax the pull of the gastrocnemius and held for countertraction (lying the patient supine on a gurney with the leg hanging off the end usually facilitates this), (c) the heel is manually distracted longitudinally, and (d) the deformity is initially exaggerated to “unlock” the foot (41,42).
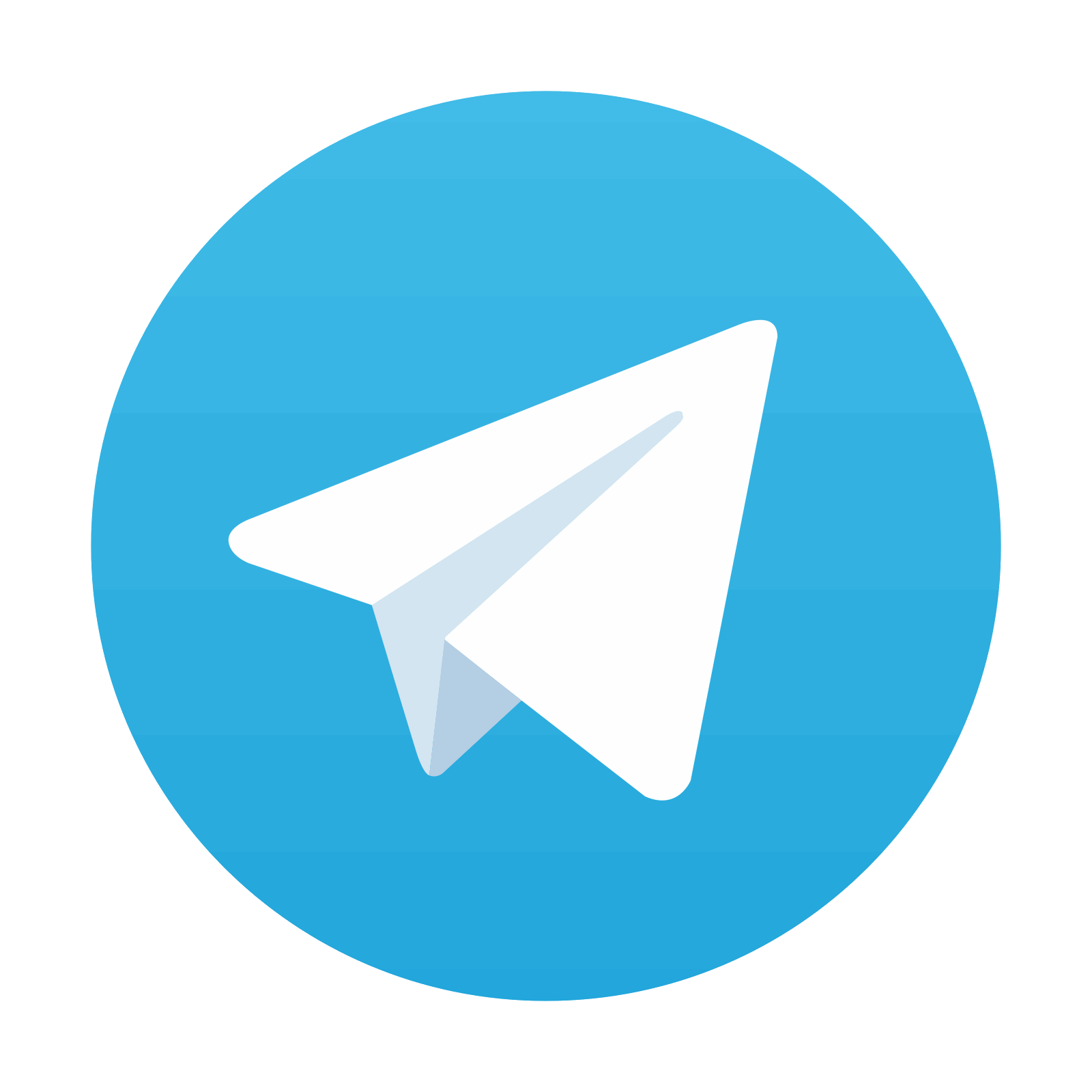
Stay updated, free articles. Join our Telegram channel

Full access? Get Clinical Tree
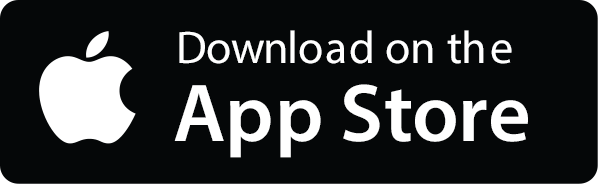
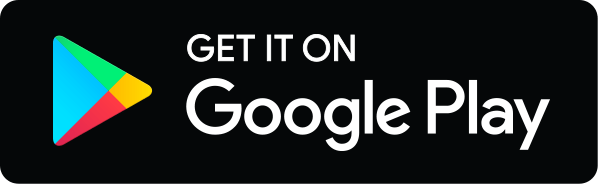
