Chapter 120 Custom-Made Cutting Guides for Total Knee Arthroplasty
Custom-made cutting guides is a new concept of using computer-assisted preoperative planning to provide patient-specific instruments that could replace conventional instrumentation systems. Preoperative computed tomography (CT) or magnetic resonance imaging (MRI) scans are acquired and imported to a special software system that has three-dimensional data of the total knee arthroplasty (TKA) implant to be used. Planning and possibly virtual surgery is performed on the computer before it is done on real patients. This includes sizing, alignment, bone cutting, and verification of optimal implantation and positioning. As described later (“Summary of the Technique”), two virtual templates are designed and transformed into physical guides using rapid prototyping technology. Information built into the guides can be transferred to the patient’s knee, and surgeons can use these guides or conventional cutting blocks. TKA can then be done without using intra- or extramedullary guides (Fig. 120-1). This revolutionary technique is considered to be an important step in the development of TKA since the introduction of instrumentation systems in the 1970s.
Computer-Assisted Orthopedic Surgery
CAOS is defined as the use of computer-enabled technology at any stage (pre-, intra-, and postoperative) in the surgical management of orthopedic conditions using various systems (active, semiactive, passive or hybrid) and delivering several applications, including planning, simulation, guidance, robotic, telesurgery, and/or training.13 Also, previous classification of CAOS focused on robotics and navigation.34 There are several CAOS techniques that extend far beyond the narrow categories of robotics and navigation. Hafez and colleagues have described a more comprehensive classification for CAOS systems based on their functionality and clinical use.9 This includes six main categories, which are then subgrouped on a technical basis (Table 120-1). This classification could be extended because future devices can later be added under new categories or subcategories. It has made it easier for orthopedic surgeons to comprehend the complexity of surgical robots and the differences in their mechanisms of action and functionality. Currently, there has been an increasing number of developed robots, up to 159 surgical robots in the literature.36 The orthopedic robots are subcategorized here only as industrial, hand-held, or bone-mounted based on mechanism of action and ergonomics. Robots actively perform surgical actions, whereas navigation acts as a position tracking device and information system, subclassified based on the mechanism of action related to imaging requirements. Hybrids combine features of robotic and navigation, subclassified in a similar way as navigation.
Table 120-1 Classification of Computer-Assisted Orthopedic Surgery (CAOS) Systems
CATEGORY | Subcategory (Based on Mechanism of Action) | |
---|---|---|
No. | Name | |
1 | Robotics | Industrial |
Hand-held | ||
Bone-mounted | ||
2 | Navigation | Image-free |
Image-based (preoperative) | ||
Fluoroscopy-based (intraoperative) | ||
3 | Hybrid techniques | Image-free and image-based |
4 | Templating | Guide (pin positioning) |
Instrument (cutting block) or tool (implant) | ||
5 | Simulation | Planning simulators (templating software) |
Virtual reality and augmented reality | ||
6 | Telesurgery | Telepresence Telementoring |
Templating (category 4) provides guidance and/or tooling, subclassified based on the mechanism of action of the templates. Simulation (category 5) provides a rehearsal of surgical procedure that improves visualization for surgeons and trainees through intuitive user interfaces (with or without interactive animation). These are subclassified based on the mechanism of action and how the data are manipulated. The preoperative digital templating software used by surgeons to size and plan hip and knee arthroplasty is an example of a planning simulator; it is the most clinically used modality representing simulation.17 Telesurgery (category 6) allows the surgeon to operate or mentor other surgeons remotely but it is not yet developed to where it can be exploited and used in clinical practice.
Robotics, Navigation, and Early Templating Techniques
Navigation techniques for TKA are now the most popular CAOS system in surgical use. This modality is passive and does not perform any steps of the surgical procedure. Instead, it provides the surgeon with intraoperative measurements and feedback. The three different types of navigation systems have been used in TKA but image-free systems are the most commonly used. Several authors have reported the better accuracy of navigation systems in TKA.1,2,4,22 Robotic techniques have been less popular and the earlier, large industrial robots that were used for hip and knee arthroplasty have been withdrawn from clinical practice.14 Recently, a newer version of has been orthopedic robots are gaining popularity and been used in unicompartmental arthroplasty7 and then TKA.
Templating and custom-made guides is a type of CAOS in category 4 in Table 120-1. It involves image-based preoperative planning, followed by the production of templates that match the surface geometry of the patient’s bony structures. The templates are designed to transfer the preoperative planning to the intraoperative performance. The production machines range from computer numeric-controlled (CNC) to a more sophisticated technology of rapid prototyping (RP), which acts as a three-dimensional printer to produce physical objects from the three-dimensional computer-aided designs (virtual templates). RP acts by joining together liquid, powder, and sheet materials, producing complex templates in the form of plastic or metals. The medical applications of this technology started in the late 1970s, with modeling and computer-aided design and computer-aided manufacturing (CAD-CAM) producing custom prostheses.3 The earliest surgical reports of using RP technology came from the dentistry and maxillofacial fields.18 In orthopedics, this technology was used in pelvic, spinal, trauma, osteotomy, and knee surgery.32,35 These applications ranged from a simple printing of anatomic models using CT images to more sophisticated preoperative planning to produce surgical guides, such as those reported by Radermacher and associates.37 This developed further to the currently used custom-made cutting guides, with more specific software allowing a complete planning of TKA based on preoperative imaging and the CAD files of the TKA prosthesis.11–13 Thus, templates or cutting guides are produced that can completely replace TKA conventional instruments.
History of Custom-Made Guides
In 2004, Hafez and coworkers12 reported a new technique of using two-piece custom-made cutting guides instead of conventional instrumentation to perform TKA. Preoperative computer-assisted CT-based planning was used to design the templates (femoral and tibial cutting blocks) that were successfully used for 17 experimental cases (14 cadaveric and 3 plastic knee specimens) without resorting to conventional jigs. This work started in 2001 and the concept of this new technique was presented by these authors as a poster at a 2002 meeting of the International Society of Technology in Arthroplasty. A complete description of the technique, including the principles and experimental use for 45 TKAs, was presented by Hafez and associates in 2006,11 naming the technique patient-specific templating.10,13 Although the term custom-made cutting guides is very popular now, the term templating is more scientific and descriptive from technical and engineering aspects. This is because the technique involves preoperative planning with sizing, alignment, cutting, and verification of implant positioning and surgical simulation, and then the production of patient-specific templates. These templates can be used as guides, cutting blocks, instruments, or tools and the concept is applicable to other procedures in orthopedics (e.g., total hip arthroplasty [THA], osteotomy, spine surgery) or other specialties such as dental and maxillofacial surgery, in which template would be more relevant than cutting guides.
Although our work on custom-made cutting guides started in early 2001, the clinical application started toward the end of the decade. When the first successful cadaveric procedure was done in 2001, it was perceived that a new era of TKA was about to begin. The technique evolved over time and modified gradually until it reached its optimal accuracy and applicability in 2004. Although the success and the revolutionary nature of this technique were obvious, it was difficult at that time to apply the technique clinically because of several reasons. New techniques are not easily accepted by senior surgeons, reviewers, and health care systems, particularly when it competes with a time-honored technique such as conventional jig systems. I used to present the custom-made cutting guide as an alternative technique to conventional instrumentation (CI); the latter was the gold standard and the only technique available for most surgeons, and was the state of the art instrumentation for many implant companies. Conversely, there was no interest from implant companies to exploit the idea because of the unlucky coincidence with the emerging navigation systems at that time. Custom-made guides were considered by some implant companies as a competitor to navigational TKA, which was their marketing tool. The delay in clinical application allowed the laboratory work to continue until 2006, with the technique validated by testing its reliability and intraobserver and interobserver variation.10,15
In 2008, Howell and colleagues20 reported the first clinical application of custom-made cutting guides, named the Otis knee. They used MRI rather than CT scanning and based the position of the implants on kinematic rather than mechanical alignment. Then, the concept of custom-made cutting guides was exploited by all major manufacturers but with some modifications, such as using MRI instead of CT and using the templates as a guide to locate conventional cutting blocks (i.e., pin locator). MRI has the theoretical advantages of detecting cartilage and being a radiation-free imaging modality. However, CT scanning was found to be more practical because of the limitations of MRI, such as difficult segmentation, certain contraindications (e.g., the presence of pacemaker, implants), and obesity. The other limitations, of different degrees according to different health care systems, are cost, long waiting list, reimbursement, and other logistical considerations. In addition, most commercially available MRI-based systems have approximately a 6-week interval from the time of acquiring the MRI scans until the templates are delivered to the hospital. This may carry the risk of anatomic changes to the knee as a result of daily activities or any abnormal loading during this long delay, thus resulting in intraoperative malpositioning of the templates and subsequently implant malalignment. The same errors of malpositioning of the templates can occur because of errors in bone segmentation with MRI, which is less likely to occur with CT. These types of errors have already been reported.26 Our CT-based software system was easy to use. This allowed surgeons themselves to do the preoperative planning and designing of the templates, unlike MRI-based systems, which have to be done planned and designed by experienced technicians because of the need to do manual segmentation of the images. For CT-based systems, the segmentation is done automatically by the software.
Rationale for Use
There are several reasons why TKA techniques and their outcomes need to be improved. Several authors1,2,4,23 have outlined the rationale for using CAOS techniques in general, which is mainly to improve the accuracy of TKA. This may apply to computer-assisted, custom-made cutting guides without the drawbacks of navigation and robotics. Moreover, the cutting guide technique is an alternative to conventional instruments and can overcome the deficiencies in conventional techniques; these disadvantages are explained by the following.
Plain radiography is the routine preoperative imaging modality for conventional TKA and is usually done in the form of short leg x-ray. Plain radiographs have limited accuracy.25,29 Ten degrees of knee flexion and 20 degrees of external to 25 degrees of internal rotation can cause significant differences in knee alignment measurements. The knee has a complex three-dimensional anatomy, so planning, alignment measurement, and bone cutting have to be done in a three-dimensional fashion. The ability to understand the three-dimensional complexity of the knee is variable and depends on the surgeon’s experiences. Surgeons make intraoperative decisions based on what they visualize (eyeballing), which is usually focused on one plane. The accuracy of visual inspection by surgeons is limited, especially when using ill-defined or inconsistent operative landmarks, such as the center of the femoral head, center of the ankle, or epicondylar axis. These errors are even greater when dealing with obese patients or in the presence of bony abnormalities. Minor errors in bone cutting, such as posterior sloping of the tibial cut and posterior femoral cut, can affect the flexion gap and errors in tibial and distal femoral cuts can affect the joint line level. Intraoperatively, it is usually difficult to determine the normal level of the joint line accurately. The recent introduction of minimally invasive surgery (MIS) has made it more difficult to visualize the knee joint or even to visualize the bone completely during cutting.
CI systems are relatively complex tools with numerous jigs and fixtures and their use is time-consuming. They need setting up before surgery, assembling and dismantling during surgery, and washing and sterilization afterward for reuse in subsequent procedures. For example, a demonstration kit for a standard size 3 primary TKA (PFC Sigma, DePuy, Warsaw, Ind) has 84 different pieces. Most primary prostheses have various options—fixed-bearing, mobile-bearing, cruciate-substituting, cruciate-retaining. There are different sizes (average, six sizes) of implants for each of these options, with additional pieces of instruments to fit the different sizes and different options. It is more common to add extra pieces of instruments or more complex sets (e.g., modified sets, MIS or revision instruments). Every implant has its own instrumentation system and, in the same hospital, there could be several different systems. A report from England has revealed more than 30 TKA prostheses in use.28 This may overload hospital inventory, sterilization services and nurses’ learning curves, and increase operating room time. The conventional instrumentation set is packed in several trays (at least four) requiring one or two additional carrying tables, which need to be positioned as close to the surgeon and nurse as possible, but the environment is often not ergonomically efficient. In addition, the tables usually are in the way of the surgical assistant(s). There is generally a lack of space and these trays may cross the zone of the laminar flow. The complexity of CI systems may adversely affect the performance of nurses, trainees, and low-volume surgeons. In one report, 50% of TKA procedures were done in the United States by surgeons who perform about six procedures/year.27 Also, these drawbacks may affect high-volume surgeons, who may want to perform 10 joint replacement procedures or more in a single day, leading to exhaustion, with higher risk of errors because of unforeseen circumstances or the complexity of cases. Furthermore, in developing countries, in which TKA procedures are not common, most surgeons and hospitals have a low volume of arthroplasty procedures. Implant manufacturers cannot permanently store their instrumentation systems in such hospitals; rather, they provide the instruments on a loan basis and the cost is offset toward the cost of the implants.
CI systems can be invasive and hazardous. The use of alignment guides involves the violation of intramedullary (IM) canals. This can lead to a higher risk of bleeding,4,23 infection,30 and fat embolism.24 The numerous pieces of CI systems are metallic and some have sharp edges, spokes, or pins. They may require drilling to attach them to bone at different steps of the procedure. These metallic edges and pins require careful handling and can potentially cause sharp injuries to the surgeon or patient. There are risks related to the long operative time, such as longer tourniquet time, contamination, longer rehabilitation, and anesthetic complications. In a retrospective analysis of postoperative complications of 17,644 TKA procedures, it was found that extended surgery time increased the rate of hematoma and infection.6 The repeated use of conventional instruments carries a theoretical risk of contamination.
CI systems, including medullary guides, are used to guide several steps of a TKA, such as sizing, alignment measurement, and bone cutting. These steps are dependent on each other and this could lead to the accumulation of errors that might occasionally pass unnoticed. Most of these steps or actions (bone cuts) are irreversible. For example, the surgeon may correct the inaccurate bone cutting by making unplanned soft tissue release. Overzealous soft tissue release on one side will lead to imbalance and the surgeon may then have to release the other side, which might lead to laxity and instability. Femoral intramedullary guides are used by most surgeons, but this technique may not be suitable for patients with significant extra-articular deformities, marked bowing, and those with prior surgery or fractures. Several other authors have reported different sources of errors from using IM guides.42 It was noted that the anatomic axis exits the distal femur at an average of 6.6 mm medial to the center of the femoral notch, whereas most implant manufacturers recommend making the entry point at the center of the femoral notch. Other sources of errors include using short rods that do not reach the isthmus of the femur or tibia, using thin rods in large IM canals, or placing a straight rod in a deformed bone.
The average bone geometry of white patients has been used for designing most CI systems, which may vary even among patients of the same ethnic origin. Nagamine and associates31 have reported several anatomic variations in 133 Japanese patients with knee osteoarthritis (OA). They found proximal tibia vara, lateral offset of the tibial shaft with respect to the center of the tibial plateau, and external rotation of the femoral component of more than 3 degrees in 20% of patients. In 2000, Tang and coworkers43 reported that Chinese patients require 5 degrees of external rotation of their femoral component to obtain a rectangular flexion gap as compared with the commonly reported 3 degrees of external rotation. The accuracy of using conventional instrumentation for sizing is also questionable.21 It is not uncommon to have a mismatch between sizing of the femoral anteroposterior (AP) and mediolateral (ML) dimensions, leading to overhang or not enough coverage. Deformities and bone loss can make sizing somewhat difficult. Selecting a smaller size implant means cutting more bone and, once the bone is cut, it cannot be reattached. Bone cutting for a smaller size implant results in more bone cut posteriorly than distally, creating a wider flexion gap. The correction of this imbalance may lead to a series of compromises; more bone is removed distally with elevation of the joint line, there is excessive soft tissue release, or both can occur, requiring a thicker tibial insert. Undersizing may also result in femoral notching.
Alternative techniques, such as navigation and robotics, have been developed. Although conventional surgical instrumentation have been repeatedly modified, it appears that further refinements are unlikely to overcome their inherent drawbacks, such as the multiplicity of instruments and medullary canal perforation. Thus, a practical alternative to CI systems is required. The recent introduction of CAOS, particularly navigation, into surgical practice has allowed surgeons to evaluate its accuracy objectively; it has been proved to be superior to conventional instrumentation systems.1,2,4,22 However, navigation techniques still require the use of conventional instruments for making the various bone cuts and they even require additional instruments and the insertion of pins, onto which tracking instruments are attached. This doubling of instrumentation systems (navigational and conventional) is costly and could overload hospital inventory, sterilization services, and operating room time. They may also increase the number of decisions that have to be made by the surgeon. There is a growing need to introduce ergonomics in the surgical workplace,41 which is more difficult to achieve with bulky navigational or robotic devices. Furthermore, navigation and robotics require registration and tracking with a continuous line of sight. The overwhelming intraoperative information from navigation systems may confuse the surgeon and complicate the decision making process. These techniques can ultimately take senior surgeons away from their comfort zone and interrupt their learning curve. The rate of complications may increase during the early stages of learning the technique. All these drawbacks have limited the broad clinical application of robotics and navigation techniques. There is a need for alternative techniques that are simple, less invasive, less expensive, and accurate, with minimal drawbacks.
Surgical Procedures
Summary of the Technique
The technique involves the use of computer-assisted preoperative planning to provide patient-specific instruments that could replace CI systems. Preoperative CT or MRI scans are imported into a special software system that has three-dimensional data of the TKA implant to be used. Planning and possibly virtual surgery is performed on the computer before it is done on real patients. This includes sizing, alignment, bone cutting, and verification of optimal implantation and positioning. Two virtual templates (femoral and tibial cutting guides) are designed and then transformed into physical guides using rapid prototyping technology. The guides have built-in information about the preoperative planning, which can be transferred to the patient’s knee when the guides are positioned on the matching surfaces of the distal femur and proximal tibia. Then, surgeons can use these guides to make all necessary cuts or guide the position of conventional cutting blocks using locating pins. These patient-specific guides should be used once because they are designed based on the surfacing matching of the patient CT scan. TKA can then be done without using sizing or alignment jigs (intra- or extramedullary). The patient’s initials, hospital number, and sides of the knee should be engraved on the cutting guides (see Fig. 120-1).
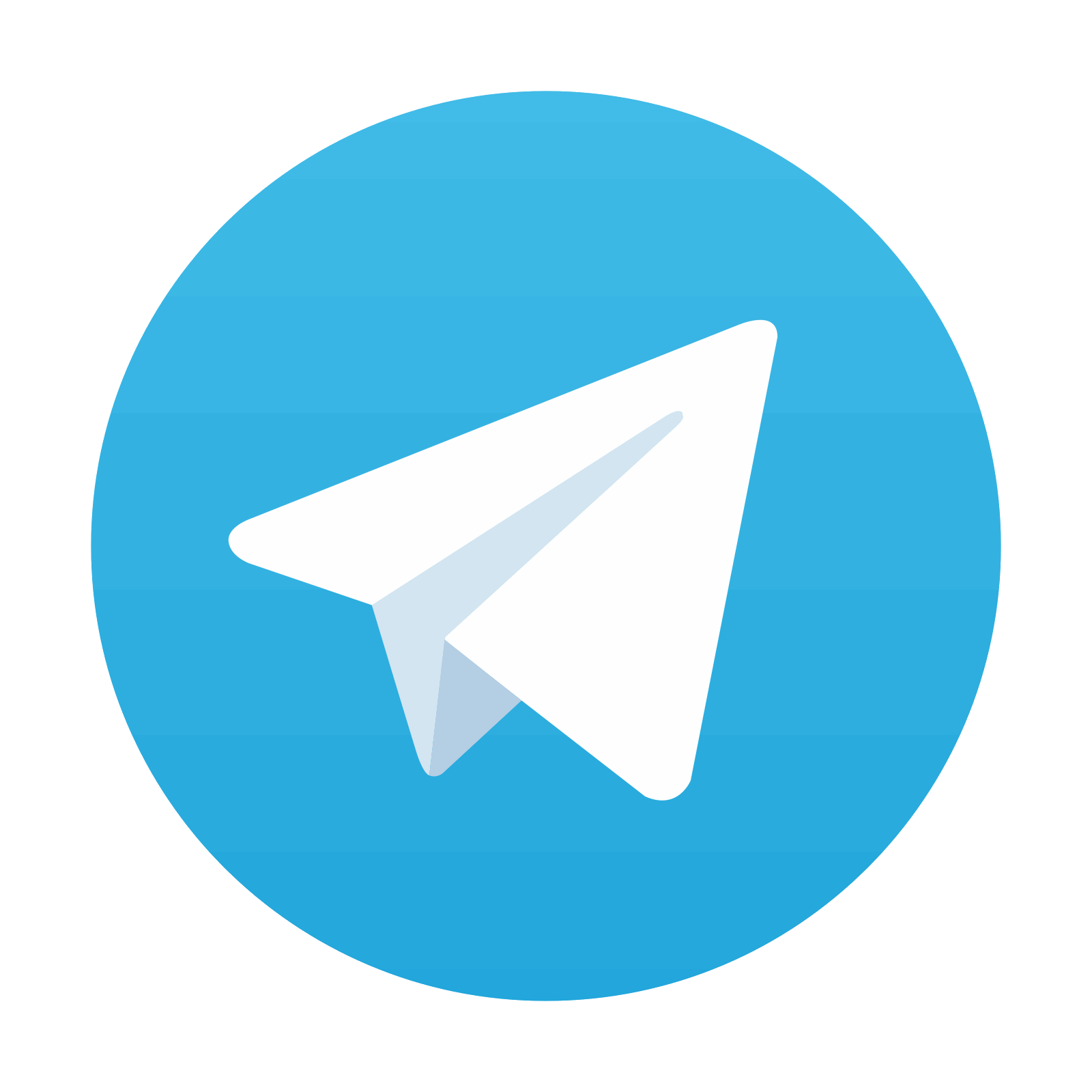
Stay updated, free articles. Join our Telegram channel

Full access? Get Clinical Tree
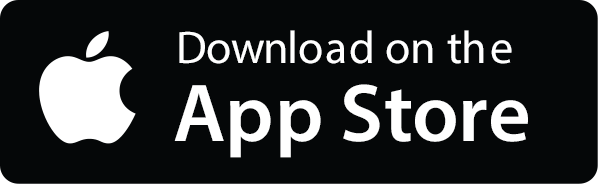
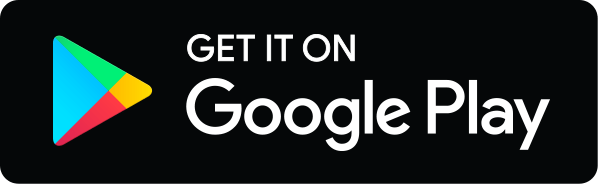