Representations of the muscles of the vertebral column as per Spigelius (1685). Note the attention to detail in the presentation

Modeling of the vertebral column by Stenonis (1685) with integration of the globality of the load . In this representation, note the mechanical continuity between the column and the lower limb
In 1892, Trolard, describing the spinal muscles, noted that the transversospinalis was most often reduced to a few fascicles. “Despite its numerous dissections, it refused to allow a classification and adopted a topographic description from superficial to deep” [12].
The papers on the strength of the muscle were carried by the German anatomists Fick [13], von Lanz, and Wachsmuth [14] at the end of the nineteenth and twentieth centuries. Fick [13] noted the relations between size and power and characterized the importance of the notion of all fascicles in relation to their maximum strength (“Cross Section Area”).
The conventional descriptions were based on the complex muscle-tendon with 2 parts, a red central muscle and a tendinous terminal. The histological and physiological evaluation must be modified and has not been developed in anatomy textbooks. Dissections that we have done since 1985 have led to a macroscopic dissection on the disposition of the fascicles with the pennation, the aponeurosis, and the fascia to support innovative therapies [15].
Organogenesis
Embryologically, the skeletal muscles derive from the mesoderm, whose cells are organized into regular groups with anteroposterior distribution with the somites. There are several species of myoblasts during ontogenesis, those who remain in the somitic territory, at the origin of the trunk muscles, and those who migrate to the limb bud. The differentiation of mesoderm into myoblastic cells is dependent on four regulatory genes : myoD, myogenin, myf5, myf6, plus a fifth less well identified: myd [16]. They are organized into 42–44 somites.

Histological sections of a fetus with positioning of somites and neural tube

At the 60th day of intrauterine life , all muscles are in their final position

During the same period, the neural tube will protect the spinal cord. Coronal section of the cervical spine: topographic relationships with the spinal cord
By the fifth week, the myotubes appear; at 8 weeks (30 mm vc), all the muscles have their mature form and change their orientation with the exception of the axial longitudinal muscles.
General and Semantic Organization of the Muscle
Curvatures: Anatomical and Biomechanical Fundamentals
The human column has undergone a double evolution with adaptation for bipedal stance and recumbency. Throughout its height, it is unstable in the three planes of space, the regulation of which is ensured by all the structures constituting two essential mechanical principles with variable curvatures and the articular tripod. The notion of curvature is old, Fick [13] integrated the hip and pelvis entirely and the functional consequences of other types of curvature. At the end of growth, we consider that its morphology is stable. If it were straight and rigid, we would have difficulty maintaining our balance and in the face of mechanical stress, the deformation would be unpredictable (buckling).
To adapt, each segment has a curvature that ensures a prestressed state of defined shape, organized to oppose precise predictive forces so as to avoid breakage.

Sagittal sections of 2 fetuses (a, b) and adult subjects (c) to show the configuration of curvatures of the spine. Progressive placement of column curvatures from the fetal period, from the newborn to the adult

Sagittal section of a vertebral column with its curvatures (a). Varieties of curvatures considered physiological according to Fick [13] (b)
The essential role in the maintenance of this attitude is devolved to the muscular tone of posture, in particular to small permanent contractions of the muscles of the paravertebral gutters and the pelvic girdle. The first biomechanical experiment on stability with incorporated muscles was presented by Bergmark [17] with an elastic function. In a histological study, Nitz [18] determined the number of neuromuscular spindles in the muscles of the lumbar portion with, in the interlamellar and interspinous muscles, where a much larger percentage of neuromuscular spindles exist than in multifidus and semispinalis.
Musculo-Aponeurotic Static and Dynamic Mechanical Imperatives

Organization of the muscles according to the principle of the stays (akin to a guy rope or cable tethers) from the shortest to the longest

Three-dimensional functional continuity of the pelvis, thoraco-lumbar and cervico-thoracic muscles
Comparative Anatomy: Curves and Muscles

Principle of anticlinism : arrangement of the spinous processes (a: side view) and transverse processes (b: dorsal view) in the dog (Anatomy museum, Montpellier)

Results of the arrangement of the vertebral column muscles by Winkler (1936) after dissection of several animal species (Sciurus vulgaris, Lupus cuniculus, Scrofa domesticus), the insertions are related to the principle of thoracolumbar vertebral anticlinism
Numbers
The number of muscles presumed to be 400 varies: for Chaussier [19] 368, Theile [20] 346, Sappey [21] 501 distributed into 190 truncal, 63 head-neck, 98 upper limbs, 104 lower limbs, and 46 of the abdomino-pelvic system.
Old Nomenclature
Their denominations were based on a single principle. Before Sylvius [22], they were designated by the numerical names of first, the second, following the order of their superposition or their use. The order of Galen [23] was entirely topographical. Vésale [24] substituted a physiological order according to their use, true or supposed, and corrected many errors.
Modern Nomenclature
For Cruveilhier [25], their shape was determined: from their resemblance either with geometric forms, with generally known objects , according to their symmetry or their lack of symmetry. Under this last report, there was a very great difference between the osseous and muscular systems. “The topographical order was preferable in many respects, in that it was essentially anatomical and offered the advantage of appreciating the relations of the muscles between them and the various regions between them; it had, from the point of view of the economy of the subjects and the facility of the preparations, an incontestable advantage on the physiological order, with which, moreover, could be reconciled for a few regions. He adopted the topographical order with some modifications which made it possible to study all myology on the same subject.”
Since the twentieth century, anatomists had wanted to use a vocabulary that is understood by everyone with a universal language with Latin, which was that of Nomina Anatomica. Since the adoption in principle of Nomina Anatomica at Oxford in 1950, they were recognized and legitimized, then recognized by the International Federation of Associations of Anatomists (IFAA).
Muscle Insertions

Diagram of digitations of the levator scapulae muscle (a, b) and dissection with its four strands (c)

Ilio-costal muscle with its lumbar and thoracic portions , schematic aspect according to Winkler (a), after dissection of an anatomical specimen (b) and representation of the emergence of the posterior branches of the spinal nerves (c). The muscular strands are very short in reference to the thoraco-lumbar aponeurosis. The thoraco-lumbar aponeurosis represents 90% of its total length, the principle of an antigravity muscle that stores energy to restore it in any circumstance

Dissection and removal of the thoraco-lumbar portion of the ilio-costal mm (a) with, in close-up (b), the muscular strands which continue with a aponeurosis for each digitation. Note the reduced length of the strands (fascicles) compared to that of aponeuroses (aponeurosis)
Another group is distinguished by the presence of the single or double origin tendon with two or more muscular bodies, with all of these musculo-aponeurotic complexes grouping together on a single tendon at its termination. The longissimus dorsi in its thoracic portion is detached from the sacral crest by a fascial layer which extends with the ilio-costal and ends with two chevrons: costal on the last five ribs and transverse on the transverse processes of T12–T7. Conversely, it may have insertions of osteo-periosteal origin as after constitution of the muscular body; it divides into several distinct tendons such as the two bundles of the dorsal part of the ilio-costal strand of the longissimus dorsi which consists of 6 tense strands of the posterior arch of the 1st and 2nd ribs towards the posterior arches of the ribs of the 5th to the 11th.
Intrinsic and Semantic Morphology
It is worth distinguishing the “anatomical muscle fascicles of the functional muscular fibers.” The new anatomical approach benefits from details of anatomical architectural parameters with overall muscular length, length of anatomical fascicles, length of muscle fiber, and angle of pennation. Its actual length is defined as the distance between the proximal insertion of the muscle fiber and its distal insertion on the fascia . This measurement is variable according to the torsion of the fibers. Their length consisting of 500–10,000 parallel myofibrils can only be determined by micro-dissection after fixation. The fascicle is the sum of several muscle fibers, difficult to isolate by microdissection and which can contain from 5 to 50 fibers. Fundamentally, the anatomical fascicle is not necessarily representative of the real function of the muscle, which can only be identified histologically [26]. The determination of long or short fibers depends on the method of fixation, the measurement of the length of the sarcomere being difficult to interpret.
Sarcomere and Myofibrils
The sarcomere forms the histological functional unit and the fascicle the macroscopic functional unit. The universal organization consists of sarcomeres, which group together in the form of myofibrils , which in turn constitute the fascicles. The sarcomere consists of actin and myosin interconnected contractile proteins arranged to slide relative to each other. The energy allowing their movement comes from the hydrolysis of the adenosine triphosphate fixed on the head of the myosin which can then tilt. This tilting pulls the action on the M band and causes shortening of up to 1 μm which can then create shortening of up to 10 cm [27].
Each myofibril is composed of 1000 to 2 million sarcomeres in series, 2–3 μm in length and 1 μm in diameter.

Schematic organization of a sarcomere which is the fundamental motor of all muscles

Organization from the sarcomeres of the constitution of a muscular fiber
Titin: The Primary Elastic Protection of the Sarcomere
Titin is the most abundant “connective” protein in striated muscle, representing 10% of the myofibrillar mass, playing a role in the organization of sarcomere components essential for their growth.
It is involved in the control of the assembly of sarcomeric proteins regulating the elasticity of sarcomere related to thick myosin filaments and extends from the Z disc to the M band (almost half a sarcomere, i.e., more than a micron in length). With a length of 1 μm, about 30,000 amino acids and a molecular weight of 4000 kDa, it is the largest known polypeptide chain controlling integrity and ensuring the mechanical stability of the sarcomere. During traction, it generates a force sufficient to oppose the stretching tension of the sarcomere and plays a role in myofibrillogenesis , thus its degradation weakens the muscle. At its termination, it constitutes an element of information at the level of the Z and M bands as a factor of stabilization of passive stretching, a true spring of protection of the sarcomere during extension. Friden and Lieber [29] showed that passive stretching imposed strains equivalent to painful maximal muscle tension that degraded titin. By force or equivalent elongation, the extensibility of the elastic component of a rat soleus was about half that of a rat’s rectus femoris. Such a difference would come from the existence of the different isoforms of titin and from its length of the extensible part. According to the type of muscle, it has different mechanical properties.
Its role is fundamental in the protection of sarcomeres during excessive traction, mainly in the vertebral column where its constraints are central to its stability.

Schematic representation of titin which is the protection factor of the sarcomere during excessive lengthening. During extension movements, titin, by its extension properties, protects the sarcomeres
When stretching the sarcomere, it generates a force capable of opposing the physiological tension of stretching. Exceeding this causes a rupture of the myosin actin bridge with trauma to the sarcomere [29]. The length of the sarcomeres varies according to the position of the joint.
Muscles and Aponeurosis
When these models join together, they result in a complex muscular organization of effective functionality. The length of fascicles of a long muscle should not be judged by that of the fleshy aspect but should encompass its associated fascial elements.
The generic term of fascicle is a functional set of sarcomeres as discussed by Huxley [30] and Alexander [31]. The particular fusiform shape of many muscles is a false image of the true length of the fascicles. The observation before dissection gives the impression that a fascicle extends from one end of the muscle to the other [32]. After a specific dissection, the particular disposition of each muscular fascicle whose muscular constitution is based on fundamental models adapted to their function with parallel fascicles, convergent with three-dimensional arrangements [13, 33–35]. In man, the length of fascicles is on average 4–5 cm, the longest being 10–15 cm. The axis of the muscle being not the same as that of the fascicles which compose it, we must study, for each, their direction with respect to the aponeuroses and the tendon.
Muscle Fasciculi and Aponeurosis
Muscle Fasciculi and Pennation : Topographical Economics
Muscles requiring considerable efforts have a large cross section with parallel fascicles, which would require an extensive and non-punctiform bone insertion. But there being no room for a muscle too large and thick, a solution has been found by an oblique arrangement of the fascicles (pennate) over the whole length of the bone and the fascia, which continues with a tendon, an organ of transmission.

Arrangement of the fascicles in a bipennate fashion with their insertion on a central fascia (white fibers) (a), and diagram of different modes of insertion of the fascicles with respect to the fascia (b)

Insertions of fascicles of the trapezius muscle at the level of the spinous processes of the cervico-thoracic vertebrae, lamellar aspect
The fascicles are implanted directly or obliquely on the aponeurosis, like the barbs of a feather on their common stem: (penna: feather), as per Borelli [11] and Stenonis [10].
Aponeurosis: Fundamental Structure
The aponeurosis is the indissociable complementary element of the muscle. The fascicles are inserted either along the axis of the fascia or obliquely to distribute the mechanical stresses avoiding rupture during muscular contraction, all along its length.

Disposition of aponeuroses (aponeurosis) (whitish fibers) of superior serratus posterior intended to distribute the constraints of muscular fascicles. It is a unipennate muscle type, observe the disposition of these aponeuroses (aponeurosis) of the superficial (a) and deep (b) aspects. Most anatomical depictions represent only one aspect that does not make it possible to understand the essential role of the aponeuroses (aponeurosis)

Dissection of serratus posterior superior and inferior (a) joined by a continuous aponeurosis (b), in this case, muscular fascicles persist along its length with the aponeurosis

Common aponeurosis (green arrow) for longissimus dorsi and ilio-costal : posterior view (a). Sagittal section (b) at the insertion on the sacrum, contrary to the overall appearance of the muscle, the muscular fascicles are very short, responding to the mechanical principle of maximum yield during contraction
A Historical Conceptual Error
It is along the faces and edges of this aponeurosis that the fascicles are born (insert). It is again on a membranous surface or aponeurosis that they terminate. This aponeurosis, gathering on itself, constitutes a terminating tendon which the fleshy fibers (fascicles) leave at a more or less considerable distance from its extremity. It results from this provision: (1) a considerable development of surface for the insertion of the fascicles which the tendon collects, so to speak, in order to concentrate their efforts on the same point; (2) the obliquity of insertion or incidence of the fasciculi in relation to the tendon which represents the axis of the muscle, that is, the direction. It is conceivable that this obliquity is of the greatest relevance in the dynamic relation of the force of action of the muscles and leads necessarily to a great loss of forces. There is a great variety in these angles of insertion or incidence of fascicles on the aponeurosis. It is conceivable that the facility of multiplying the fascicles by arranging them in this way obliquely outweighs the disadvantage of their direction.
The original tendon extends as a form of membrane or aponeurosis in the thickness or surface of the muscle.
Intrinsic Structure of the Aponeurosis: Endomysium and Perimysium Aponeurosis

Organization of the aponeurosis in the muscle which promotes the transmission of the stresses. Histological section at high magnification
The different muscular fascicles are not randomly grouped, but constitute regular bundles surrounded by an outer layer of dense connective tissue, limiting the muscles as a whole called epimysium.
In 1989, Ettema and Huijing [2], on the physiological level, led to an interpretation and a comprehensive mechanical synthesis of the internal structures of the fascicle and aponeurosis. For the sarcolemma of isolated fibers, the endomysium contributes to the elastic static function as it can be stretched to 140–150% and contributes to the elasticity and viscosity of the muscular complex. The sum of the connective tissue increases the compliance of the muscle in addition to that of the fascicles and the tendon.
Due to the tension that is transmitted through the aponeurosis to the endomysium, the mechanical properties of this connective tissue are a factor contributing to improve the mechanical properties of the muscle, transferring the stresses between the discontinuous fascicles through transendomysial bundles. In contrast to the high tensile forces of the collagenous fibers on which the fascicles attach themselves, the translaminar leaflets undergo shear forces of the endomysium . Each muscle fiber is surrounded by a fibrous tissue (perimysium ) which is organized into cells which, by their adhesion, undergo progressive elastic transmission of mechanical shear stresses.

Posterior view of the large posterior cervical complex according to Winkler (a) and after dissection with its fascial intersection and its two bundles (b). During the movements of anterior flexion of the head (c), it is essential that the muscles consist of an aponeurosis (c) which protects the tension of the sarcomeres

Disposition of variable aponeuroses (aponeurosis) in the continuity of the muscles according to the law of association and commutativity intended to balance the transmission of stresses with identical contraction of all muscular fascicles
Mechanism of the Aponeurosis

Modeling of the muscle–aponeurosis–tendon complex according to the Hill principle with the contractile muscle component (C) and the elastic aponeurotic component (PE) and that of the tendon (SE) in series and in parallel

Representation of sporting activities based on the restitution of energy (archery, pole vault)
When the force decreases from the maximum force (optimum length) to a force equal to its passive resistance (initial state), the aponeurosis decreases in length by 9%, resulting in work of 4.8 μJ. Dissection of the lumbar portion of the longissimus dorsi (antigravitary) shows a aponeurosis that occupies 95% of its length. The aponeurosis mass occupies the lateral part of the lumbar curvature which inserts on the deep medial aspect of the rough surface of the iliac bone between the articular surface and the medial lip of the iliac crest. Each bundle ends with a lateral chevron on the costiform processes and a medial chevron on the accessory tubercles of the lumbar vertebrae.
When standing, the fascia behaves like a passive rope that maintains joint balance without involving muscular contraction resulting in a significant saving of energy expenditure.
During one movement, its length variation is considered an important factor in explaining the difference in muscle length changes. For Lieber [27], during passive movements in the frog, the elasticity of the aponeurosis was 8% and for the tendon of the semi-tendinosis it was 2%. Huijing [28] showed a greater extension of the aponeurosis of gastrocnemius in the rat when the muscle was in contraction. For the same mechanical effect, the tendon had a tension of 3 to 4%, which indicated that the compliance of the aponeurosis was different from that of the muscle. The lengthening of the muscle depends on the properties of the aponeurosis which is an important element as a factor of storage of energy and its release during muscle contraction. The elastic energy stored during the passive elongation is very low (about 0.04 μJ) because of the small levels of force, if the muscle is activated to a certain length, the aponeurosis is stretched by about 2% resulting in 0.56 μJ elastic energy storage. For an active muscle, if the muscular force decreases, the force exerted on the aponeurosis is also reduced; this results in a release of energy of 3.15 μJ. The consequence is that the relaunched energy of 3.5 μJ can be obtained if the fascia follows the force/length displacement curve during the isometric contraction of a muscle. The properties of the fascia appear to be an important field for architectural modeling applications of a muscle. For muscular modeling, therefore, the stiffness of the fascia must be incorporated for elongations of less than 10%. The origin of the restitution of energy is still obscure, but may be dependent on the aponeurosis-tendon junction. All these fundamental notions find their application in the structure of the posterior muscles of the vertebral column, where the aponeuroses occupy a prominent place.
Muscle with Parallel Fascicles: Pseudo-Penniform

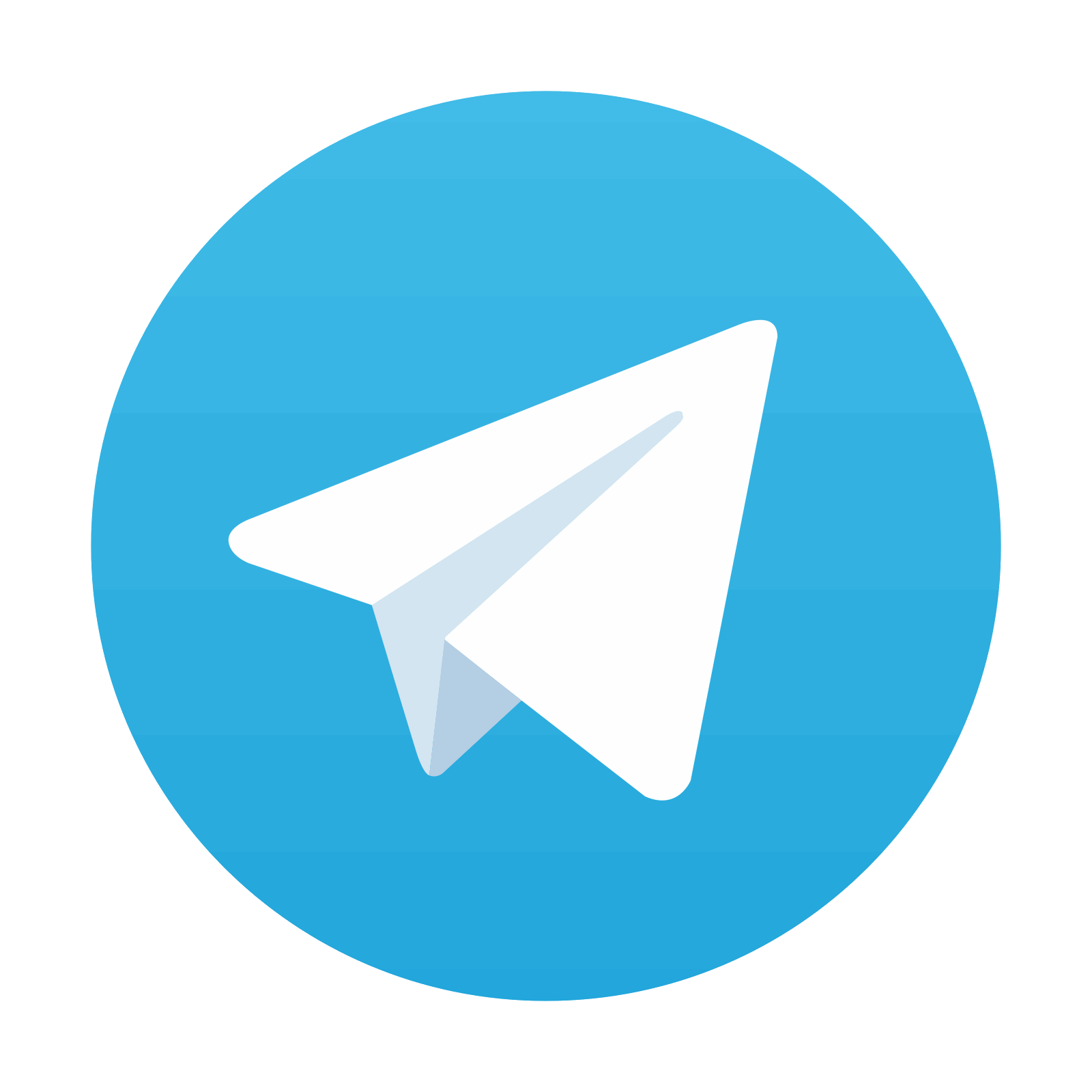
Stay updated, free articles. Join our Telegram channel

Full access? Get Clinical Tree
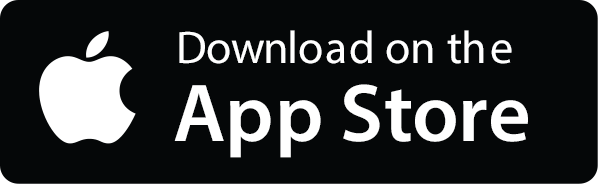
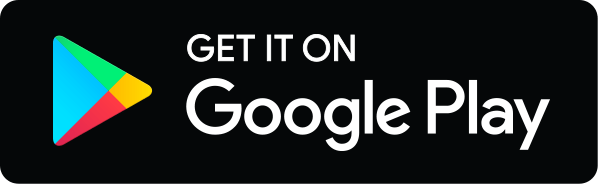