Chapter 37 Classification of Knee Ligament Injuries
Relationship of Capsular and Ligamentous Structures
Medial Structures
Hughston and colleagues36 Warren and Marshall,92 and LaPrade and colleagues50 have clearly described the supporting structures on the medial side of the knee (Fig. 37-1). The medial collateral ligament (MCL) and the posteromedial capsular ligament, termed the posterior oblique ligament, are augmented by the dynamic stabilizing effect of the capsular arm of the semimembranosus tendon and its aponeurosis, the oblique popliteal ligament. The medial head of the gastrocnemius provides dynamic support to the medial compartment. Brantigan and Voshell10 have described the MCL as having vertical and oblique portions that behave differently as the knee flexes. The parallel anterior fibers of the superficial medial ligament are arranged around the axis of flexion so that tension remains constant throughout the arc of motion. Posteriorly, the oblique fibers of the superficial ligament blend with the deeper layer within the posteromedial corner to form the posterior oblique ligament, which relaxes in flexion (Fig. 37-2).
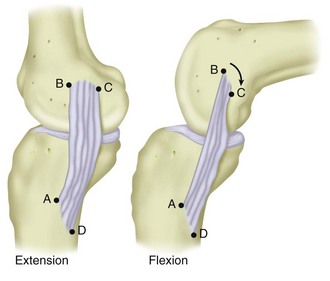
Figure 37-2 Medial collateral ligament in flexion and extension.
(From Scott WN [ed]: The knee, St Louis, 1994, CV Mosby.)
Hughston and associates35,36,41 have indicated that the posterior oblique ligament is the primary medial support against valgus stress to the knee. Although somewhat lax as the knee goes into flexion, the posterior oblique ligament is dynamized by the muscular attachment to the semimembranosus tendon, and it has a significant influence on stability throughout the first 60 degrees of flexion (Fig. 37-3). Muller61 has also stated that the posterior oblique ligament is paramount in abolishing any valgus laxity in the extended knee. Even though the posterior oblique ligament and tibial collateral ligament are functionally independent, they are both dynamized, the former by the semimembranosus tendon and the latter by the vastus medialis. This study also claims that the MCL has some function in preventing external rotation of the tibia on the femur with the knee flexed. However, the main deterrent to external rotation is the posterior oblique ligament.
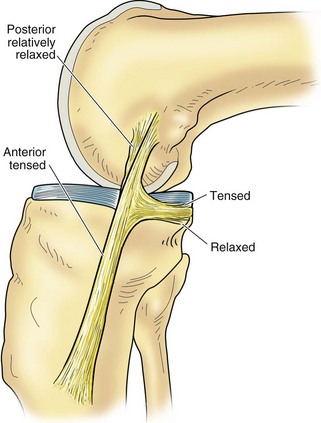
Figure 37-3 The posterior oblique fibers become more tense in flexion.
(From Palmer I: On the injuries to ligaments of the knee joint. A clinical study. Acta Chir Scand 81[Suppl 53]:3, 1938.)
In contrast to Hughston and Muller, Warren and coworkers92 have noted that most fibers of the MCL are the prime static stabilizers on the medial side of the knee. The anterior 5 mm of the MCL remains tight in flexion and thus resists valgus stress in external rotation in this position (Fig. 37-4). According to their study, the posterior two thirds of the superficial MCL is slightly lax with the knee in flexion and therefore serves as a backup restraint against valgus and external rotatory forces. In their reports, Hughston and colleagues35,36,41 have labeled the oblique fibers of the posterior aspect of the superficial MCL as the posterior oblique ligament. This is a thickening of the posteromedial capsule of the knee and it is firmly attached to and contiguous with the medial meniscus in this location.
Still another viewpoint, presented by Kennedy and Fowler,47 has identified the deep medial capsular ligament as a primary restraint against external rotatory instability. They concluded that external rotatory instability is caused by a tear in the medial capsular ligament, with or without a partial or a complete tear of the MCL. Grood and associates,33 in a biomechanical study of cadaveric knees, have concluded that the long parallel-oriented fibers of the tibial collateral ligament are the prime medial static stabilizers of the knee that resist medial opening to valgus stress. Although this ligament contributes to medial support with the knee close to full extension, as the knee flexes, the importance of this ligament increases from providing 57% of the restraining force to providing 78% of the restraining force. This increase is caused by the relaxation or laxity that develops with flexion in the other contributing structures, mainly the posteromedial capsule. The deep medial capsular ligament, although important in that it provides a firm attachment site for the medial meniscus, does not serve as a primary restraint against straight medial opening.42
Although these previous qualitative assessments are useful in developing an understanding of medial-sided knee injuries, LaPrade and coworkers50 have made a quantitative assessment of the medial structures of the knee. Claiming that the layered approach is not helpful for surgical exposure, because it often leads to an oversimplification of structures with frequent inaccuracies regarding ligamentous attachment sites, they sought to verify the relationships of medial knee structures to pertinent osseous anatomy through cadaveric dissection. They confirmed that the medial epicondyle lies anterior and distal to the adductor tubercle. They also consistently recognized a third, previously undescribed osseous prominence, which lies distal and posterior to the adductor tubercle, near the attachment depression of the medial gastrocnemius tendon. They labeled this new structure the gastrocnemius tubercle and noted that the posterior oblique ligament attachment was actually closer to this structure than to the adductor tubercle.
The superficial MCL is the largest medial knee structure, measuring between 10 and 12 cm in length, but it does not attach directly to the medial epicondyle, as previously described.10,41,91 Its femoral attachment lies slightly proximal and posterior.50 There are two distinct tibial attachments. Proximally, it attaches to the soft tissues directly over the anterior arm of the semimembranosus. Distally, it attaches to bone approximately 6 cm from the joint line.
The superficial, central, and capsular arms of the posterior oblique ligament are readily identifiable, with the central arm forming the largest component of this structure. The central arm contributes most fibers for femoral attachment and adheres to the medial meniscus as it merges distally with the posteromedial capsule.50
A subsequent study by Wijdicks and colleagues94 has concluded that the attachments of medial ligamentous structures could be correlated to the location of osseous landmarks seen on plain radiographs. This may aid in pre- and intraoperative assessment of surgical repairs and reconstructions of these structures.
Lateral Structures
The lateral supporting structures have been described by Hughston and associates37 and Seebacher and coworkers74 (Fig. 37-5). The lateral collateral ligament (LCL) is the major static support to varus stress, whereas the iliotibial tract provides both dynamic and static support. Terry and colleagues85 have investigated the role of the iliotibial tract, iliopatellar band, and iliotibial band as dynamic and static stabilizers of the lateral side of the knee.
The posterolateral corner is composed of the LCL, popliteus aponeurosis, popliteofibular ligament (PFL), and posterolateral capsule. Although these individual static structures have often been grouped together as the arcuate ligament complex, other studies have highlighted their individual importance. Together, their function is augmented by the dynamic effects of the biceps femoris, popliteus, and lateral head of the gastrocnemius.5
On the femoral side, the LCL attaches to a small depression between the lateral epicondyle and supracondylar process, and it attaches distally to the posterior aspect of the fibular head.12,51 The popliteus originates from the posteromedial aspect of the proximal tibia, courses intra-articularly, and inserts anterior and distal to the LCL attachment. Its function in providing dynamic stability to the lateral meniscus is controversial—countering views on its role in retraction and protection of the meniscus have been espoused.78,88,89 The PFL arises from the myotendinous junction of the popliteus and inserts on the fibular styloid process.
Anterior Cruciate Ligament
The anterior cruciate ligament (ACL; Fig. 37-6) is the primary structure that controls anterior displacement in the unloaded knee. The anatomic and functional aspects of the ACL have undergone extensive investigation.57–5984 For reconstruction purposes, the focus has often centered on the relationship of the ligament to osseous landmarks. On the femoral side, the anterior border of the ACL is a bony ridge on the medial wall of the lateral femoral condyle, commonly referred to as resident’s ridge.71 On the tibial side, the ACL posterior border lies at a ridge between the medial and lateral intercondylar tubercles at the base of the tibial eminence.
The ACL has been described as a single ligament, with different portions taut throughout the range of motion (ROM).6 In investigating the functional anatomy of the ACL, Odensten and Gillquist67 found no anatomic separation of the ligament into different bundles. However, they did confirm that the ligament is twisted through 90 degrees, and that both the length49,86,89,90 and tension27 of different fibers in the ligament change as knee flexion occurs. Therefore, they believe that there are different functional portions of the ACL.93 Based on this concept of different functional portions of the ACL, Girgis and associates29 have divided the ACL into anteromedial and posterolateral bands. Norwood and Cross62 further divided the ACL into three functional bundles and described their different actions in resisting rotatory instability. Amis and Dawkins3,4 have supported this multifascicular structure of the ACL; although not necessarily separate entities, the bundles interact as three functional bundles. They found that the fiber bundles were not isometric; the anteromedial bundle lengthens and the posterolateral bundle shortens during flexion (Fig. 37-7). These changes in fiber length correlate with their changing participation in total ACL action as the knee is flexed.
Tibial rotation is better resisted by a combination of capsular structures, collateral ligaments, the joint surface, and meniscal geometry, whereas the cruciates play only a secondary role.3,4,65 However, recent evidence suggests a larger role for the ACL in rotational stability if both bundles remain functionally intact.14 Despite this, the MCL is anatomically better suited than the ACL and has the mechanical advantage to control torsion or laxity because its attachments are further removed from the axis of tibial rotation.77 The MCL will provide significant resistance to the anterior drawer test only after the ACL is gone and when both ligaments are lost. In this scenario, the knee will exhibit large tibial excursions and response to anterior force if it is unchecked by muscle action. Injuries to the medial structures further compromise anterior stability when they accompany ACL injuries.81
Posterior Cruciate Ligament
The posterior cruciate ligament (PCL; Fig. 37-8) is believed to be the most important of the knee ligaments because of its cross-sectional area, tensile strength, and location in the central axis of the knee joint.* Its position provides 95% of the total resistance to posterior displacement of the tibia. Both James and associates44 and Kennedy and coworkers48 have shown that the tensile strength of the PCL is almost twice that of the ACL. Hughston and colleagues36 have described the PCL as the fundamental stabilizer of the knee because it is located in the center of the knee joint and functions as the axis about which the knee moves in flexion and extension, as well as in rotation.
The PCL prevents posterior translation at all angles of flexion.13,26,34 Patients who have an isolated injury of the PCL may maintain fairly good function of the knee.25 Gollehon and associates30 have found that isolated sectioning of the PCL produces increased posterior translation of the tibia at all degrees of flexion of the knee, with the greatest increase occurring from 75 to 90 degrees. Absence of the PCL has no effect on primary varus or external rotation of the tibia as long as the LCL and capsular structures are intact.
Like the ACL, the PCL is a continuum of fascicles, with different portions being taut throughout ROM. The anterior portion, which forms the bulk of the ligament, tightens in flexion, whereas the smaller posterior portion tightens in extension (Fig. 37-9).6 The PCL originates from the posterior part of the lateral aspect of the medial femoral condyle and inserts on the posterior surface of the tibia. The femoral footprint consists of a medial intercondylar ridge at its proximal border and a medial bifurcate ridge that occasionally divides the two functional bundles.24 The insertion reaches approximately 1 cm below the articular surface in a nonarticular area that Jacobsen43 has termed the area intercondylaris posterior. Tajima and coworkers82 have further investigated the tibial insertion site and noted that the insertions of its two bundles are located in different planes, with a change in slope beween them. Lying anterior to the PCL and connecting the posterior horn of the lateral meniscus to the medial femoral condyle is the ligament of Humphry (Fig. 37-10). The ligament of Wrisberg passes posterior to the PCL to attach on the PCL. The ligaments of Humphrey and Wrisberg (Fig. 37-11) are so intimately related that early authors described them as separate portions of a single ligament.55 Clancy and colleagues15 have noted that the meniscofemoral ligament may serve as a secondary stabilizer in a posterior cruciate-deficient knee. The presence of these structures may account for the absence of posterior drawer in isolated PCL tears.
Kinematics
In classifying knee ligamentous instabilities, it is important that the terms be clearly understood and used in a lucid and universally accepted manner. The terms in the literature should be specific to define positions of the knee, motions of the knee, and ligamentous injury. Noyes and associates66 have taken the time to review the literature and define terms that are in common orthopedic usage.
Position
Position refers to the orientation of the tibia with respect to the femur and determines the tension in each of the ligaments and supporting structures. Dislocation is a term indicating a complete noncontact position of both the tibia and femur or the patellofemoral joint. Dislocations of the knee are classified by the final tibial position-anterior, posterior, medial, lateral, or rotary.52 Subluxation is defined as an incomplete partial dislocation and does not have limits. It can be described in an anteroposterior, medial lateral, or rotary position.
Motion
Motion is the process of changing position and describes the displacement between the starting and ending points. Displacement is the change in position and is described according to 6 degrees of freedom, a combination of three translations and three rotations. Translation is the parallel displacement of a rigid body or, in the case of the knee joint, the tibia with respect to the femur. Translation of the tibia is composed of three independent components or translational degrees of freedom—medial lateral translation, anteroposterior translation, and proximal distal translation. Rotation describes motion or displacement about an axis and, in the knee, has 3 degrees of freedom—flexion-extension, internal-external rotation, and abduction-adduction. Range of motion is defined as the displacement that occurs between the two limits of movement for each degree of freedom. There is a ROM for each of the translational and rotational degrees of freedom. For motions other than flexion-extension, ROM generally depends on the angle of knee flexion. The limits of motion are defined as the extreme positions of movement that are possible in each of the 6 degrees of freedom. Injury to the ligamentous and osseous structures about the knee alters the limits of motion. By convention, the limits of flexion and extension are described relative to the neutral position or extension of 0 degrees, with flexion described in positive terms and hyperextension in negative terms. Coupled displacement concerns motion in 1 or more degrees of freedom that is caused by a load applied in another degree of freedom. The amount of coupled rotation depends on where the force is applied to the tibia or on whether the center of rotation is constrained or allowed to move freely. An example is the internal rotation that results when an anterior load is applied to the tibia.26 When assessing ligamentous stability, motion of the knee joint may occur freely or be constrained, based on the integrity of the ligamentous structures. The ligaments determine the constraint of the knee joint. Elongation or stretching of the ligament limits joint motion and is also supported by compressive joint contact forces that act in an opposite direction. Two ligaments are required to limit translation and rotation, one for each direction. A single ligament alone is unable to resist rotation. If the motion is unconstrained, the tibia displaces into its maximum position. Most clinical tests performed on the knee, however, are constrained.
Classification of Ligament Injuries
Several terms have been used to describe an injury to a ligament.
Sprain
A sprain is an injury to a joint ligament that stretches or tears ligamentous fibers but does not completely disrupt the ligament. In the handbook Standard Nomenclature of Athletic Injuries,1 sprains were characterized on the basis of indirect evidence of ligament injury, including the history, symptoms, and physical examination (Fig. 37-12). A first-degree sprain is a tear involving a minimal number of fibers of a ligament, with localized tenderness and no instability. A second-degree sprain tears more ligamentous fibers, with slight to moderate abnormal motion. In a third-degree sprain, there is a complete tear of the ligament, with disruption of fibers and demonstrable instability. Third-degree sprains are further subdivided as follows: grade I, less than a 0.5-cm opening of the joint surfaces; grade II, a 0.5- to 1-cm opening of the joint surfaces; and grade III, a rupture larger than a 1-cm opening. Rupture of a ligament implies complete tearing of the ligament, with concomitant loss of function. Because a ligament may undergo a complete tear but still retain continuity between displaced fibers, it is the loss of function (resistance to displacement) that defines a tear, not the property of continuity.66 Deficiency of a ligament implies that the ligament is absent or that there is loss of function, such as when the ligament still exists but is stretched and nonfunctional.60
Instability
The most elaborate classification system of knee ligament instability was developed by Hughston and colleagues36,37 and the American Orthopaedic Society of Sports Medicine Research and Education Committee in 1976.2 This classification system attempts to describe the instability by the direction of tibial displacement and, when possible, by structural deficits. The classification of knee ligament instability is based on rotation of the knee about the central axis of the PCL. All rotatory instabilities indicate subluxation about the intact PCL. Once the PCL is damaged, the instability is designated as straight instability, which indicates subluxation or translation without rotation around a central axis. The subluxation hinges on the intact MCL or LCL.
Rotatory Instability
Rotatory instability includes anteromedial, anterolateral, posterolateral, posteromedial, and combined (Fig. 37-13).72 Combined instability is not as clearly defined as rotatory or straight instability.
Anteromedial Rotatory Instability
External tibial rotation plus anterior translation is manifested as anteromedial rotatory instability, which causes the medial tibial plateau to subluxate anteromedially on the medial femoral condyle.38 This motion implies disruption of the medial capsular ligament, MCL, posterior oblique ligament, and ACL.41,46,47,80 The medial meniscus is considered an important stabilizing structure and may also be injured.70 On clinical examination, the abduction stress test result is positive, with abnormal excess opening of the medial joint space at 30 degrees, along with positive anterior drawer and Lachman test results.
Anterolateral Rotatory Instability
This instability results in excessive internal tibial rotation and anterior subluxation, which implies disruption of the lateral capsular ligament, the arcuate complex, and the ACL. The iliotibial band may be damaged to a varying degree, with most of the injury occurring to the deep fibers, which are attached to the posterior cortex of the lateral femoral condyle. Clinical examination reveals positive results for an adduction test at 30 degrees of flexion and for the anterior drawer, Lachman, and pivot-shift tests. The anterior drawer test result with the tibia rotated externally will be negative because the tibia will not be able to rotate internally. The radiographic presence of a Segond fracture implies an avulsion fracture of the attachment of the anterior oblique band of the lateral capsule from the tibia. This finding, associated with a tear of the ACL, is pathognomonic for anterolateral rotatory instability.73
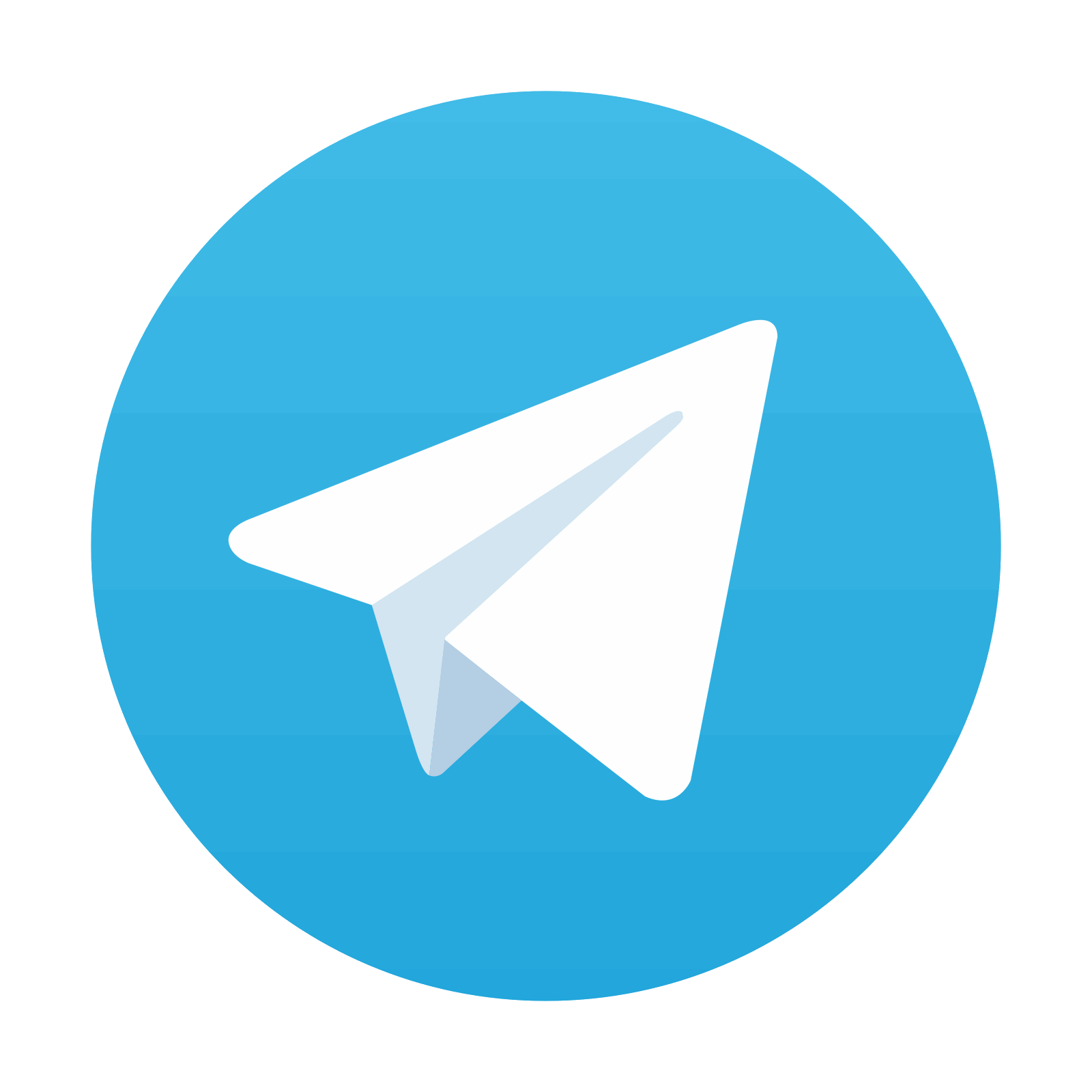
Stay updated, free articles. Join our Telegram channel

Full access? Get Clinical Tree
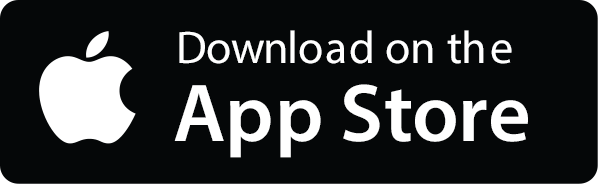
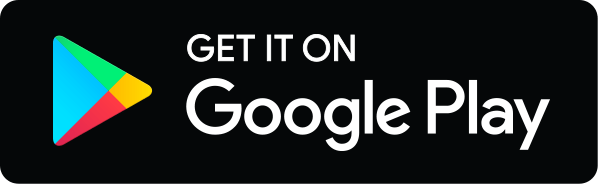