Cervical Spine Injuries in the Athlete
Rahul Banerjee MD
Michael P. Bradley MD
Mark A. Palumbo MD
Paul D. Fadale MD

Football, ice hockey, rugby, skiing, snowboarding, and equestrian athletes have been identified as being at an increased risk of spinal cord injury. Athletic injuries are the fourth most common cause of spinal cord injury. The risk is small, but such an injury can be devastating to a young player’s career and life.
Equipment and rule changes, as well as education of coaches, have helped to reduce the risk of injury.
If the space available for the spinal cord is reduced because of a narrow canal, an athlete is at greater risk. Cord compression can be anticipated when the diameter of the midsagittal cervical spinal canal is 10 mm or less.
Cervical spine injuries can be classified as either catastrophic or noncatastrophic.
Catastrophic injuries can be defined as a structural distortion of the cervical spinal column associated with actual or potential damage to the spinal cord. Such injuries include unstable fractures and dislocations, transient quadriplegia, and acute central disc herniation.
The vast majority of injuries are noncatastrophic. These injuries include neuropraxia of the cervical root or brachial plexus (known as a “stinger” or “burner”), paracentral intervertebral disc herniation, stable fractures, spinal ligament injury, and intervertebral disc injury.
In football and hockey, the injury vector most frequently associated with cervical spinal cord injury is compression (axial loading).
During competition, sports medicine staff should make efforts to monitor play. A visual image of the traumatic event can be useful in attempting to determine both the type and the severity of injury.
The initial evaluation follows the ABCDE sequence of trauma care: A, airway maintenance with cervical spine protection; B, breathing and ventilation; C, circulation; D, disability (i.e., neurological status); and E, exposure of the athlete.
Regardless of the etiology, the primary objective when respiratory compromise exists is to rapidly identify hypoxia and then intervene by providing proper ventilation for the injured player. This must be accomplished without causing any further injury to the spinal and neurological structures.
Protective equipment should be removed before transport only in select cases.
Unless an emergency exists that requires removal of the helmet and/or shoulder pads, initial screening radiographs can be obtained with the protective equipment in place. Lateral computed tomography (CT) scout films have been used effectively in this case.
Cervical spine injuries in the athlete continue to present a small but inherent risk and may devastate a young player’s career and life. The injured athlete must be handled cautiously until the extent of skeletal and neurological injury can be defined. Athletic injuries are the fourth most common cause of spinal cord injury (behind motor vehicle accidents, violence, and falls) and account for approximately 7.5% of the total injuries since 1990 (1). Sports-related spinal cord injuries occur at a much younger age (mean age, 24 years) compared with other causes of this injury (2). Furthermore, sports injuries are the second most common cause of spinal cord injury under the age of 30 years (3). Several sports, including football, ice hockey, rugby (4,5), skiing (6,7), snowboarding (6,7), and equestrian (8), have
been identified as carrying an increased risk for athletes to sustain spinal cord injury. American football and ice hockey, which are the most popular collision sports around the country, account for most these cervical spine injuries. In addition, these sports have been, perhaps, most influenced by changes in injury and prevention secondary to their popularity and high visibility worldwide.
been identified as carrying an increased risk for athletes to sustain spinal cord injury. American football and ice hockey, which are the most popular collision sports around the country, account for most these cervical spine injuries. In addition, these sports have been, perhaps, most influenced by changes in injury and prevention secondary to their popularity and high visibility worldwide.
Defining the spectrum of cervical spine injuries can range from temporary, fully recoverable injuries to permanent, catastrophic injuries. A catastrophic cervical spine injury can be defined as a structural distortion of the cervical spinal column associated with actual or potential damage to the spinal cord. As mentioned, these catastrophic injuries not only can instantly change an athlete’s life but also leave them with irreversible and devastating neurological consequences. Many advances in protective equipment, including helmet design and modifications in rules of play, have helped to decrease the rate of these injuries in collision sports. Recognizing and quantifying injury data on a national level have helped to drive several changes in these collision sports; however, this clinical problem challenges the sports medicine physician during early, on-field decision making. Furthermore, because of the overall low incidence of catastrophic cervical spine injuries, few physicians develop the extensive experience and essential skills that are necessary for the emergency care of cervical spine injuries. Perhaps the single most important primary decision deals with handling of the cervical spinal column on the field and during transport. Many authors now agree that improper handling of the spine during the early stabilization period can worsen spinal cord dysfunction. An athlete’s cardiac and respiratory status can be significantly compromised with improper management of the cervical spine. Furthermore, the initial management of a collision sport athlete versus that of a typical trauma victim with an injured cervical spine is very different. Specifically, the protective helmet and shoulder pads worn by the player complicate the medical evaluation and immobilization process during initial management; however, the consensus currently is to leave helmets and shoulder pads in place unless specific circumstances exist. Many clinical scenarios will be reviewed at the end of this chapter.
In this chapter, we will review the epidemiology, functional anatomy, and diagnostic considerations that are relevant to cervical spine trauma in the athlete. We will consider specific injury patterns and clinical syndromes. Although a number of different sports have been associated with spine injuries, we will focus primarily on the collision sports of American football and ice hockey. The unique aspects of the protective gear (i.e., helmet and shoulder pads) in these sports will be described. We also will provide the reader with a concise protocol for on-field diagnosis and management of the athlete with an injured spine. Finally, we will review initial management of the spine-injured athlete on transfer to the emergency room.

Equipment modifications, rule changes, and educational efforts directed at athletes, coaches, and parents have helped to decrease the incidence of cervical spine injuries. The potential for catastrophic injuries in collision sport athletes, however, continues to exist.
American Football
Roughly 1.8 million athletes per year participate at various levels in the collision sport of football. Approximately 1.5 million participate at the junior/senior high school level. Additionally, approximately 75,000 play in college, and approximately 2,000 are in professional football (9). Although spinal cord injury is uncommon in this sport, a significant burden of cervical trauma exists. Despite the fact that football has a lower rate of catastrophic cervical spine injuries (per 100,000 players) compared with ice hockey or gymnastics, the large number of participants has resulted in football being associated with the largest overall number of catastrophic cervical spine injuries in the United States (9,10).
In football, specific patterns of injury to the cervical spine have evolved over time. From 1959 to 1963, Schneider (11) documented 56 cases (1.36 per 100,000 participants) of cervical fracture/dislocation, of which 30 cases (0.73 per 100,000) were associated with permanent quadriplegia. From 1971 to 1975, the National Football Head and Neck Injury Registry compiled 259 cases (4.14 per 100,000) cervical fracture/dislocations and 99 cases (1.58 per 100,000) of quadriplegia (12). This disturbingly high rate of cervical spine injury was thought to be influenced by the introduction of modern football helmets. Techniques that incorporated the helmet top as the initial point of contact during tackling had been implemented, and it became apparent that using this initial point of contact for blocking or tackling placed the cervical spine at increased risk of injury.
This important realization helped to shape major rule modifications in American football. Head-first contact was banned by the National Collegiate Athletic Association (NCAA) Football Rules Committee and high school football governing bodies early in 1976. Penalties for “spearing” behavior were adapted by the National Federation of High Schools (9,13,14). These important rule changes forced players and coaches to adhere to techniques in tackling that helped to decrease the incidence of spinal cord injury in football for close to a decade. From 1976 to 1987, the rate of cervical injuries decreased by 70%, from 7.72 per 100,000 to 2.31 per 100,000, at the high school level (13). Additionally, traumatic quadriplegia decreased by approximately 82% over the same time period, from an annual rate of 2.24 per 100,000 to 0.38 per 100,000 in high school football and from 10.66 per 100,000 to 0 per 100,000 in college football (9). The yearly incidence of permanent cervical quadriplegia from 1975 to 1995 is shown in Figure 5-1.
Most recent data indicate a continued plateau in the incidence of traumatic quadriplegia from approximately 1991 to present. In 2002, the incidence of this injury was 0.33 per 100,000 in high school football and 1.33 per 100,000 in college football (9).
The incidence of catastrophic spine injuries in American football from 1977 to 2001 was reviewed by Cantu and Mueller (10). In their review of this 25-year period, the authors reported 223 football players sustained a catastrophic cervical spine injury with either no or incomplete recovery. In summary, 183 injuries occurred in high school athletes, 29 in college athletes, 7 in professional athletes, and 4 injuries in recreational players. The incidence rates, therefore, over the past 25 years are 0.52 in high school, 1.55 in college, and 14 in professional football. Interestingly, more defensive players were affected (71% versus 29%), and most of these injuries resulted from tackling (69%). Fracture/dislocations accounted for almost 80% of these catastrophic injuries.
Ice Hockey
Ice hockey continues to be increasingly popular throughout the United States and Canada. In contrast to American football, hockey experienced a marked increase in the occurrence of cervical spine injuries since 1980. The Canadian surveys performed by Tator et al. (15) from 1966 to 1993 reported a total of 241 spinal fracture and dislocations related to hockey. Almost 90% of these injuries were neck related and occurred between C1 and the cervicothoracic junction. An alarmingly increased rate had been documented from 1982 to 1993, with an average of 16.8 fractures/dislocations per year. Of the 207 athletes in the Canadian registry with adequate documentation of neurological status, 108 (52.2%) sustained a permanent spinal cord injury, and in 52 (25.1%), the cord lesion was complete. Eight players died as a result of complications of their spinal cord injury.
The annual incidence of spinal cord damage with paralysis is at least threefold greater in Canadian hockey than in American football (9,15). We have noted before that overall total catastrophic neck injuries are reportedly higher in football, but this is attributed to increased participation. Hockey seems to pose a much higher rate and total number of permanent paralysis and death because of spinal injury.
Checking an opponent from behind (“boarding”) has been identified as an important causative factor of cervical spine trauma in hockey. This playing tactic typically produces a head-first collision of the checked player with the boards (15,16). In an effort to prevent these injuries, rule changes have been adopted that prohibit both checking from behind and checking of an opponent no longer controlling the puck. Data from the Canadian registry suggest that fewer cases of major spinal column trauma and complete quadriplegia have been caused by illegal playing techniques since the institution of these rule changes (15). Furthermore, educational programs, such as the Safety Toward Other Players (STOP) program, have helped to decrease injury by providing a visual reminder (in the shape of a Stop sign) on the back of players’ jerseys to prevent checking from behind (17) (Fig 5-2).

Understanding the structure of the cervical spine and the pathoanatomy of characteristic patterns of injury is essential for initial, effective management. Simply stated, the cervical spine supports the head and protects the neural elements; but motion about the cervical spine can be quite complex. Many agree that distinct regional differences exist between the upper cervical spine and the lower cervical spine. In this section, we will briefly explore some of these important characteristics.
The occiput and the first two vertebrae make up the upper cervical spine(Fig 5-3). The atlas (C1) is a bony ring that articulates with the occipital condyles. The major function of the atlanto-occipital joint is motion in the sagittal plane. In fact, 40% of all cervical flexion and extension occurs above the axis (C2). In contrast, only 5° to 10° of lateral bending occurs at the atlanto-occipital joint. The axis (C2) has a true vertebral body, from which the odontoid process, or dens, projects. The major stabilizing force at this joint is the transverse atlantal ligament (TAL). The ligament crosses posterior to the dens and attaches to C1 on both sides; this prevents anterior translation of the atlas on the axis. This specialized osseoligamentous anatomy allows C1 to rotate on C2 in a highly unconstrained manner, providing 60% of all cervical rotation (18).
The lower cervical spine consists of the C3 through C7 vertebrae. These joints account for the remaining arc of neck motion, including flexion, extension, lateral bending, and rotation. Two contiguous vertebrae and supporting soft tissues make up a motion segment, and these motion segments can be separated into an anterior and a posterior column (Fig 5-4). Stability of a cervical motion segment is derived mainly from the anterior column elements. The vertebral bodies and intervertebral discs provide the majority of resistance to compression. In contrast, it is the surrounding paraspinal musculature and ligaments that resist shear forces.
Of course, the coronal orientation of the articular processes of the facet joints in the cervical spine provides additional resistance to anterior translation. Distraction and tensile forces are resisted by the annulus fibrosus and longitudinal ligaments. Secondary support structures include the supraspinous and interspinous ligaments, the ligamentum flavum, and the facet capsules (18).
The spinal cord lies within, and is protected by, the upper and lower spinal columns. From the foramen magnum to the cervicothoracic junction, the osseoligamentous structures of the cervical spine provide a protective space described as the cervical spinal canal. This spinal canal occupies a funnel shape from cephalad to caudal (19) (Fig 5-5). In fact, the cord occupies less than half the canal’s cross-sectional area at the level of the atlas, but this space reduces significantly in the lower cervical spine. Almost 75% of the cross-sectional area of the canal is occupied by the larger spinal cord between C4 and C7 (19).
The dimensions of the lower cervical spine remain relatively consistent among most patients (20), but some important, distinct differences exist. For instance, the diameter of the midsagittal cord averages between 8 and 9 mm; however, a range of 14 to 23 mm exists for the vertebral canal at the
corresponding level. Criteria for radiographic stenosis are anteroposterior dimensions measuring less than 13 mm on a lateral radiograph (21). Additionally, a Pavlov ratio of less than 0.8 has been used to describe stenosis (Fig 5-6). Even without radiographic criteria for a stenotic canal, the potential for increased risk at the time of injury exists secondary to anatomical variation.
corresponding level. Criteria for radiographic stenosis are anteroposterior dimensions measuring less than 13 mm on a lateral radiograph (21). Additionally, a Pavlov ratio of less than 0.8 has been used to describe stenosis (Fig 5-6). Even without radiographic criteria for a stenotic canal, the potential for increased risk at the time of injury exists secondary to anatomical variation.
![]() Fig 5-5. The diameter of the spinal canal progressively narrows in a cranial-to-caudal direction, with the space available for the spinal cord being at a minimum between C4 and C7. |
In summary, if the space available for the spinal cord is reduced because of a narrow canal, an important risk factor and predictor of acute neurological dysfunction is present (22). When the diameter of the midsagittal cervical spinal canal is 10 mm or less, cord compression can be anticipated (21). Cervical canal stenosis has been implicated as a risk factor for the burner phenomenon (see Fig 5-13) in college football players (23).

Cervical spine injuries can be classified, as described previously, as either catastrophic or noncatastrophic. The vast majority of injuries are noncatastrophic. Catastrophic cervical spine injuries include unstable fractures and dislocations, transient quadriplegia, and acute central disc herniation. Additionally, some congenital spinal anomalies can place an athlete at increased risk for injury. All these conditions typically produce neurological symptoms and signs that involve the extremities in a bilateral distribution.
As mentioned, only a very small percentage of football and hockey players sustain a catastrophic cervical spine injury. Most cervical spine injuries and injury patterns associated with collision sports do not involve spinal cord injury. In contrast to catastrophic injuries, these common syndromes usually do not affect the extremities in a bilateral fashion. Most neck-injured athletes display clinical findings in (a) a single upper extremity, (b) the neck and arm, or (c) the neck only. These syndromes include neuropraxia of the cervical root or brachial plexus (the “stinger” or “burner”), paracentral intervertebral disc herniation, stable fractures, spinal ligament injury, or intervertebral disc injury.

In this section, we will review the four most common catastrophic cervical spine injuries (unstable fractures and dislocations, transient quadriplegia, acute central disc herniation, and congenital spinal anomalies) experienced by the sports medicine physician and specific concerns in each scenario.
Unstable Fractures and Dislocations
As expected, unstable fractures and/or dislocations make up the majority of catastrophic spinal injuries in athletes. An osseous or ligament injury is considered to be unstable when it results in loss of the ability of the spine, under physiological loads, to maintain its premorbid patterns of motion, so there is no initial or additional damage to the spinal cord or nerve roots, no major deformity, and no incapacitating pain (24).
Spinal column damage occurs when the force and resistance balance of the normal motion of the spine is exceeded. Injury patterns often can help to illustrate the mechanism of cervical motion and damage. In football and hockey, the injury vector most frequently associated with cervical spinal cord injury is compression (axial loading) (13,15,25). A smaller percentage of injuries result from excessive flexion. Finally, extension, lateral stretch, and congenital instability also have been reported in cervical spine injury (26).
Initial loading most often occurs at the vertex of the collision athlete’s helmet. The cervical spine is then left to compress between the mass of the oncoming body, which represents the instantly decelerated head, and the mass of the remaining body. Perhaps most influential in determining specific injury patterns is the neck position at the time of impact. Neutral alignment leaves the cervical spinal column slightly extended because of the normal lordotic posture. Compressive forces in this position are dissipated by the
anterior paravertebral musculature and vertebral ligaments (anterior longitudinal ligament) (Fig 5-7A). Slight flexion will eliminate cervical lordosis and direct force along the spine’s longitudinal axis. This will result in large forces being transferred directly to the vertebrae as opposed to the surrounding soft tissues (13) (Fig 5-7B). Cadaveric studies have shown that the cervical spine, when straight and colinear with the applied load, responds to compression by buckling (27) (Fig 5-7C).
anterior paravertebral musculature and vertebral ligaments (anterior longitudinal ligament) (Fig 5-7A). Slight flexion will eliminate cervical lordosis and direct force along the spine’s longitudinal axis. This will result in large forces being transferred directly to the vertebrae as opposed to the surrounding soft tissues (13) (Fig 5-7B). Cadaveric studies have shown that the cervical spine, when straight and colinear with the applied load, responds to compression by buckling (27) (Fig 5-7C).
Most fractures and dislocations in injured athletes occur in the lower cervical spine. Two major patterns of compressive spinal column damage exist. Most often, evidence of a compressive–flexion injury is present as a result of a combination of axial force and a bending moment. The anterior column shortens under loading. We then see compressive failure of the vertebral body and tensile failure of the posterior spinal ligaments (Fig 5-8A). This pattern can be highly unstable both anteriorly and posteriorly, with displacement of the anterior fracture and widening of the posterior elements, and it often may be associated with spinal cord injury (28).
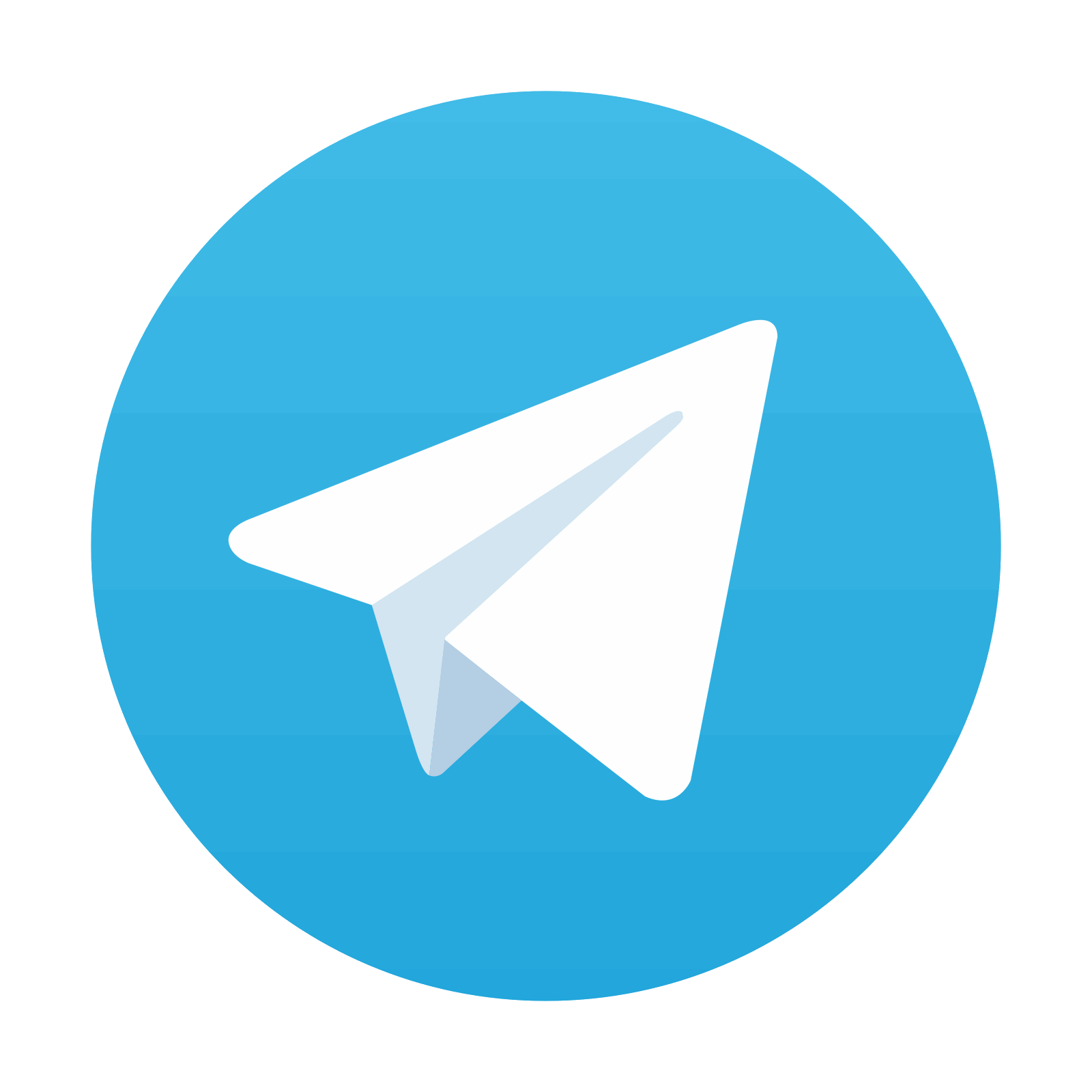
Stay updated, free articles. Join our Telegram channel

Full access? Get Clinical Tree
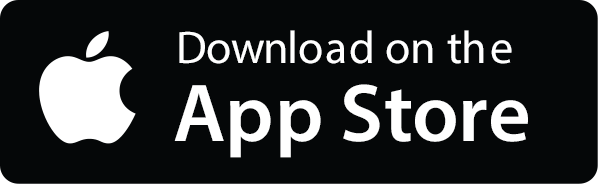
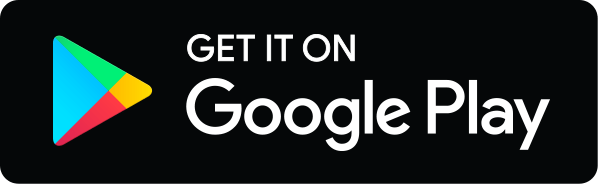