CHAPTER 51 Cervical Instability
INTRODUCTION
The definition of spinal instability has been a subject of considerable debate for decades, even among experts. According to White and Panjabi, ‘the loss of the ability of the spine under physiologic loads to maintain relationships between vertebrae in such a way that there is neither initial nor subsequent damage to the spinal cord or nerve root, and in addition, there is neither development of incapacitating deformity or severe pain.’1 In this definition, physiologic loads refer to those incurred during normal activity of the patients being examined. They preferred to emphasize the relationship between the mechanical derangement and pain or neurologic deficit. They also preferred a checklist to standardize the evaluation of cervical instability and show a systematic approach.
Earlier, Allen et al.2 presented a mechanistic classification system of injuries to the lower cervical spine. In their opinion the skeletal injury, the neurologic injury, associated injuries, medical disorders, and unique individual factors should be analyzed in each case to determine acute injury and risk for late instability.
Instability has been defined in anatomical, biomechanical, and clinical terms, but the variability of clinical presentations and the inability of sequential laboratory lesions to replicate clinical experiences indicate that instability should be defined in anatomical terms. Larson has emphasized that the stable spinal column is symmetrical in movement and configuration, whether normal or abnormal, and does not change with time.3,4 In considering spinal instability, it is necessary to generally review the column theory of vertebral stability. The two-column or three-column concepts of Holdsworth, White, Denis, and Louis are frequently used to conceptualize the mechanical integrity of the spinal column.1,5–7 The definition of the extent of injury to the soft tissues and bony components of the spinal column will assist the clinician in determining the risk to neural structures from alterations in stability or alignment. Although inclusion of a middle column has a theoretical advantage in thoracic and lumbar injuries, these considerations are not as anatomically important in the cervical spine and it is reasonable to use the two-column model in the cervical region (Fig. 51.1). As previously noted, laboratory studies of cervical instability determined through a sequential pattern of component section of a specific column are not usually representative of clinical injury which may include only selective elements of each column.8 The degree and character of a column compromise, however, are important indicators alerting the clinician to the potential risk of instability. Additionally, this information, especially the potential role of ligamentous injury, will assist in recognizing the possible development of delayed instability (subacute or chronic) which may be difficult to establish in the early post-trauma period. The degree of instability, irrespective of temporal occurrence, will be an important influence upon the threat or occurrence of neurologic injury. Therefore, clarification of the degree of injury and a more precise measurement of displacement will assist in determining the threat to neurologic integrity.
UPPER CERVICAL SPINE
Anatomic and biomechanical considerations
The anatomic stability of occipito-atlanto-axial joint complex is gained mainly by the ligament, capsule, and structural membrane (Fig. 51.2). The stability of occiput–C1 joint is provided by their capsule, along with the anterior and posterior atlanto-occipital membrane, enhanced by the additional structures such as the alar ligament, apical ligament, and the tectorial membrane. The transverse, dentate, and apical ligaments play an important role in structural stability of the atlantoaxial joint. The injury or dysfunction of the transverse ligament leads to the translation of C1 on C2 and widening of the atlantodens interval (ADI), which can be detected by lateral X-ray of the cervical spine. Functional loss of the alar ligaments indicates a potential for rotatory instability, which, however, must be determined in conjunction with other clinical findings, such as neurological dysfunction, pain, and deformity.9
Knowledge of the normal movements of the occipito-atlanto-axial joint complex is important for evaluating clinical cases that may be potentially unstable. The occiput and axis are sometimes described as two rotating squares with the atlas as a bearing between them. The joints between the atlas and axis are almost horizontal and therefore allow for a wide range of motion in rotation, whereas rotation between the occiput and atlas is completely prevented by the geocentric anatomy of the articulation. The investigations of measurement of range of motion of C0–C1–C2 are shown in Tables 51.1 and 51.2. Both joints, C0–C1 and C1–2, participate in flexion and extension. Extension at occiput–C1 is one of the largest single motions in the spine. Lateral bending occurs at occiput–C1, but is almost negligible at C1–2. The greatest intervertebral motion in the spine is axial rotation at the C1–2 joint.10,11
Table 51.1 Average Rotation in Degrees at the Atlanto-occipital Joint of C0–1 According to Different Investigators
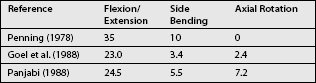
Table 51.2 Average Rotation in Degrees at the Atlanto-occipital Joint of C1–2 According to Different Investigators
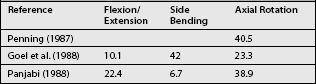
The mean occipitocervical angles were 24.2°, 44.0°, and 57.2° in flexion, neutral, and extension, respectively. The mean occipitocervical distances were 21.5 mm in neutral, 28.0 mm in flexion, and 14.8 mm in extension.10 These are simple to determine on routine lateral radiographs. The differences in the occipitocervical angle and occipitocervical distance in neutral, flexion, and extension are significant. These measurements should be a valuable intraoperative tool for achieving occipitocervical fusion in appropriate alignment.
The term coupled motion denotes that motion about one axis consistently occurs simultaneously with motion about another axis (rotation or translation).12 It is most dramatic in the cervical spine. Two kinds of coupled motion are especially well known in the cervical spine: axial rotation in the same direction as applied lateral bending, and lateral bending in the same direction as applied axial rotation. Posture affects motion coupling patterns of the upper cervical spine. The most dramatic change due to modification in posture is found in coupled sagittal plane rotation, which changes from extension at extended posture to flexion at flexed posture at both levels and in response to both load types. For the axial torque, the main axial rotation and coupled lateral bending changes little with posture.
Diagnosis
In daily clinical practice, one often deals with patients with neck pain or stiffness resulting from injury or the ongoing degenerative changes rather than those with no relevant complaint. Active motion in patients with complaints of neck pain or stiffness is usually restricted by the motion-induced pain. Due to the closely related trigeminus nuclei, segmental functional disorders in the suboccipital area cause, in addition to local neck pain, frontoparietal and retro-orbital pain that can also irradiate to the upper and lower jaw. In addition, tendomyoses with trigger points and difficulties in swallowing may occur in the anterior part of the neck.13 The historical information collected from the patient is important in making the diagnosis, even given the aid of radiologic technology.
A few recognized criteria of radiology for C0–C1–C2 instability have been established. For instance, when the ADI value, which may describe the range of C1–2 translation, is larger than 3 mm in lateral flexion and extension view, the atlantoaxis is considered unstable.14 Asymmetry of the odontoid lateral mass interspace on open-mouth view is also an important clue for diagnosis. One should also assess whether vertical migration of the odontoid process exists, especially in rheumatoid patients. For evaluation of the function of the upper cervical spine, especially assessment of the range of motion, functional X-rays films are useful in addition to the clinical examination (Fig. 51.3). For the diagnosis of segmental instability, passive motion should be induced in order to obtain the full range. If anterior instability of the upper cervical spine is suspected, flexion–extension X-rays in the lateral view are appropriate. If a lesion of the alar ligaments is suspected, then lateral flexion X-ray films should be taken. In the normal situation, the atlas glides in the direction of bending, coupled by forced rotation of the axis. In cases with rotatory instability of the upper cervical spine, functional computed tomography should be performed. Atlantoaxial rotation of more than 52° should be considered pathological as a result of a lesion of the alar ligaments. For examination of the relationship between the spinal cord and bony structures or inflammatory tissue in patients with rheumatoid arthritis, functional MRIs are helpful.
Functional magnetic resonance tomography is an important diagnostic method for assessing the cervical spine in patients with rheumatoid arthritis. In particular, fusion and instabilities as well as compressions of the spinal cord or medulla often can only be detected with the help of functional MRI. Compared with static MRI examinations in patients with rheumatoid arthritis, functional magnetic resonance imaging identifies the extension of pannus tissue cranial, ventral, and dorsal to the dens with possible displacing and impinging effects on the spinal cord during flexion and extension. In addition, it is suitable for demonstration of the degree of instability in the atlanto-occipital and atlantoaxial planes. In contrast to conventional X-rays, CT, and static MRI, basilary impression as well as compressions and angulations of the spinal cord are better visualized by cinematic magnetic resonance tomography.15 Cineradiography is a valuable continuous recording tool and has been used in motion analysis of the musculoskeletal system (Fig. 51.4).16 Cineradiography has advantages over other techniques for analyzing the details of cervical spinal motion and diagnosis of spinal instability.17–20 The outcome from the cineradiographic motion analysis of the cervical spine can be taken into account to determine the level of spinal fusion for cervical spondylosis. An instantaneous center of rotation deduced from cineradiographic films has also been used to clarify the quality of motion and to justify therapy for the neck region.
Hino et al. investigated the kinematics of the normal and pathologic atlantoaxial joints by cineradiography to determine the in vivo kinematic parameters for the quantification of atlantoaxial instability.21 The kinematics of the atlantoaxial joints were evaluated by cineradiography in healthy volunteers and in patients with atlantoaxial subluxation. The results revealed the different onset points of a rapid increase in motion between flexion-from-E and extension-from-F in atlantoaxial motion. They were defined, respectively, as points A and B (Fig. 51.5). The discrepancy between these points (i.e. zone A–B) was significantly more remarkable in patients with atlantoaxial subluxation than in the volunteers. Furthermore, in most of the cases with atlantoaxial instability, subluxation occurred when the cervical spine was in a more extended position, and it was reduced in a more flexed position.
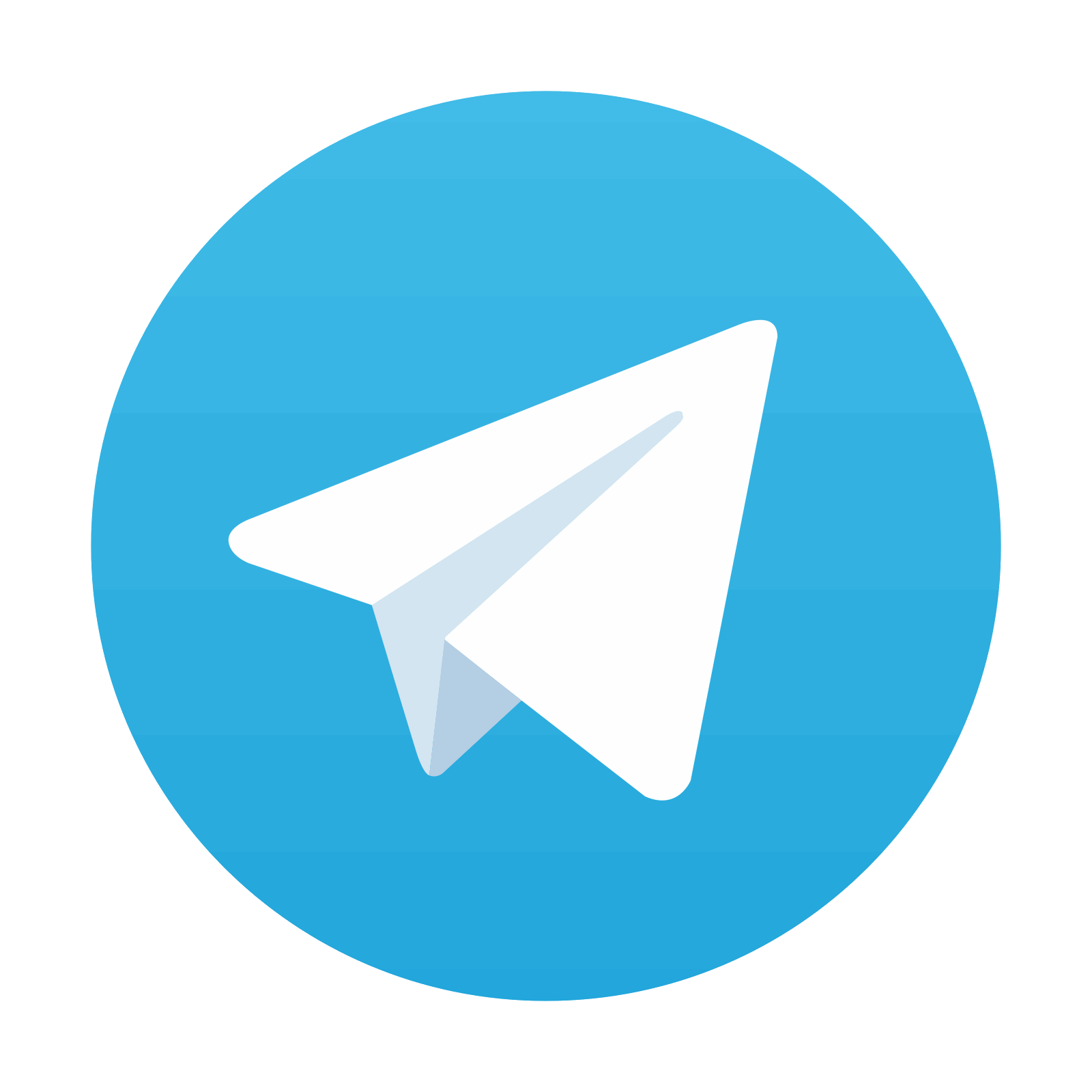
Stay updated, free articles. Join our Telegram channel

Full access? Get Clinical Tree
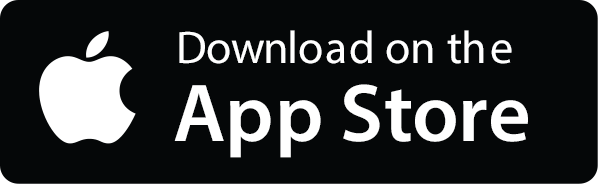
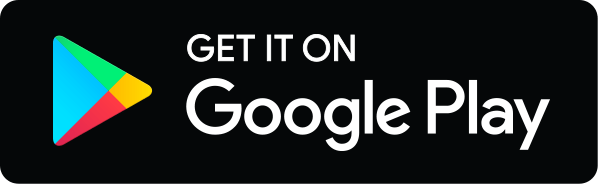