Chapter 1
Background information
The term ‘spinal cord injury’ is used to refer to neurological damage of the spinal cord following trauma. In most developed countries, the incidence of spinal cord injury is between 10 and 80 cases per million per year.1,2 Approximately half of all spinal cord injuries occur in people aged under 30 years.3–6 The typical person with spinal cord injury is male, aged between 15 and 25 years; only about 15% of spinal cord injuries affect females and only 18% affect people over 45 years.3 Obvious exceptions to these demographics occur in natural disasters. For example, in the Pakistan earthquakes of 2005 the majority of spinal cord injuries (estimated to be over 1500) were in young women and children.
The most common causes of spinal cord injury are motor vehicle and motor-bike accidents, followed by falls.3,7 Work-related injuries are also common, as are injuries from sport and water-based activities. In some countries the incidence of spinal cord injury from gun, stab or war-related injuries is high. Spinal cord lesions can also be due to disease, infection and congenital defect.
Over 55% of all spinal cord injuries are cervical; the remainder are approximately equally divided between thoracic, lumbar and sacral levels.8,9 The most common level of injury is C5, followed by C4, C6 and T12, in that order.10 A spinal cord injury in the cervical region affects all four limbs, resulting in tetraplegia (also called quadriplegia). Spinal cord injuries in the thoracic, lumbar or sacral region affect the lower limbs and result in paraplegia. Most spinal cord injuries do not involve transection or severing of the spinal cord.11,12 Rather, the cord remains intact and the neurological damage is due to secondary vascular and pathogenic events, including oedema, inflammation and changes to the blood–spinal cord barrier.13,14
The extent of damage to the spinal cord is highly variable and, consequently, a spinal cord injury can prevent the transmission of all or just some neural messages across the site of the lesion.9 In some patients the only sign that part of the spinal cord has been preserved is very slight movement or sensation below the level of the injury. For other patients there may be extensive preservation of motor and sensory pathways enabling them to walk almost normally. Partial preservation of the spinal cord is more common following cervical, lumbar and sacral injuries than thoracic injuries. It is also more common today than 20 years ago because of advances in retrieval, emergency and acute management reducing secondary neural damage (see Figure 1.1).15
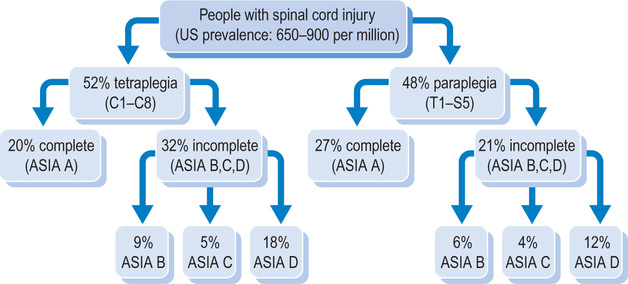
Motor, sensory and autonomic pathways
The vertebral column consists of seven cervical, 12 thoracic, five lumbar, five sacral and four coccygeal vertebrae, although the sacral and coccygeal vertebrae are fused. Emerging from the spinal cord are 31 pairs of anterior and posterior nerve roots: eight cervical, 12 thoracic, five lumbar, five sacral and one coccygeal. At each level an anterior (ventral) pair of nerve roots carries motor nerves and a posterior (dorsal) pair of nerve roots carries sensory nerves. The anterior and posterior roots join to form two spinal nerves, one on either side of the spine, which then exit the vertebral canal through the intervertebral foramina. Once outside the intervertebral foramina they form peripheral nerves.16
While there are eight pairs of cervical spinal nerves there are only seven cervical vertebrae. This disparity occurs because the first pair of cervical spinal nerves exits above the first cervical vertebra just below the skull. However, the eighth pair of cervical spinal nerves exits below the last cervical vertebra (see Figure 1.2).17
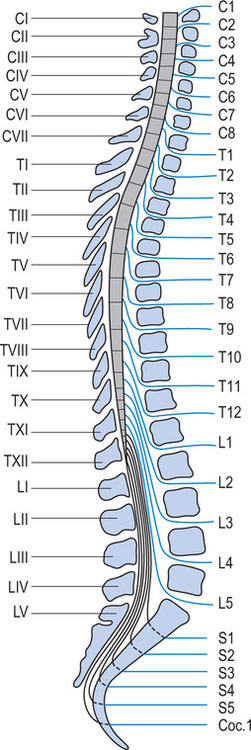
Motor pathways
Upper and lower motor neurons connect the motor cortex and muscles. The upper motor neurons originate within the motor cortex and then travel down the spinal cord within the corticospinal tracts. These tracts are also called pyramidal tracts. Approximately 85% of upper motor neurons cross over to the contralateral side in the brainstem and then travel within the lateral corticospinal tract. The other 15% cross within the spinal cord at the level they terminate and are carried within the medial corticospinal tract. The cervical upper motor neurons are centrally located within the corticospinal tract and the lumbar and sacral neurons are peripherally located (see Figure 1.3). This explains patterns of neurological loss seen with certain types of incomplete spinal cord injuries where the peripheral rim of the spinal cord is undamaged (see p. 11). There are also other complex motor pathways contained within the extrapyramidal system.
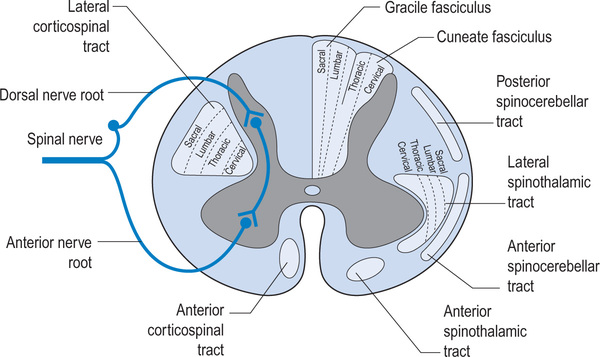
Autonomic pathways
The spinal cord not only carries motor and sensory nerves but also autonomic nerves (see Figure 1.4). Sympathetic nerves exit the vertebral canal via thoraco-lumbar spinal nerves, and parasympathetic nerves exit via sacral spinal nerves. Consequently, patients with cervical lesions lose supraspinal control of the entire sympathetic nervous system18 and of the sacral part of the parasympathetic nervous system. Patients with thoracic, lumbar or sacral lesions lose varying amounts of supraspinal control of the sympathetic and parasympathetic nervous system as determined by the level of the lesion. Some parasympathetic fibres are carried within cranial nerves and are unaffected by spinal cord injury.
The ASIA assessment of neurological deficit
Spinal cord injuries are classified according to The American Spinal Injury Association (ASIA) classification system.19 The classification is based on a standardized motor and sensory assessment (see Figure 1.5). It is used to define two motor, two sensory and one neurological level. It is also used to classify injuries as either complete (ASIA A) or incomplete (ASIA B, C, D or E).
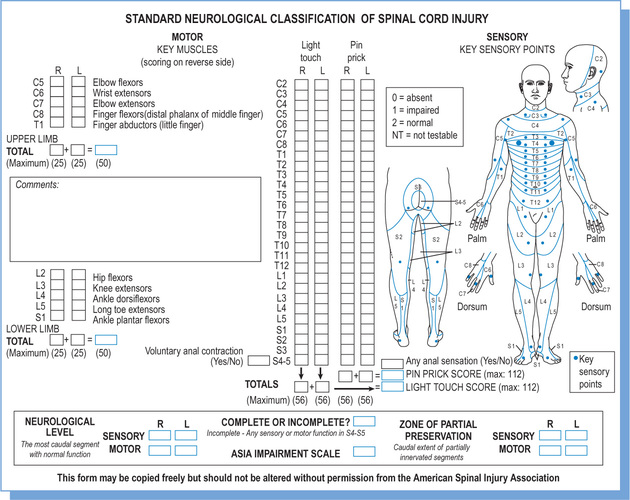
The ASIA motor level
A motor assessment is used to define two motor levels: one for the right and one for the left side of the body. An ASIA motor assessment involves testing the strength of ten key muscles. Each key muscle group represents one myotome between C5 and T1, and between L2 and S1 (see Table 1.1).
Table 1.1
C5 | Elbow flexors | L2 | Hip flexors |
C6 | Wrist extensors | L3 | Knee extensors |
C7 | Elbow extensors | L4 | Ankle dorsiflexors |
C8 | Finger flexors (middle finger) | L5 | Long toe extensors |
T1 | Little finger abductors | S1 | Ankle plantarflexors |
Each muscle is tested for strength on the original six-point manual muscle testing scale where:
1 = a flicker of muscle contraction
2 = full range of motion with gravity eliminated
3 = full range of motion against gravity
4 = full range of motion with added resistance
The main difference between an ASIA motor assessment and a standard manual muscle test20 (see Chapter 8) is that the ASIA test is performed with patients in the supine position. This position is used because it is important to standardize the testing position and often recently injured patients cannot be moved from the supine position. Limb positions are manipulated to vary the effects of gravity (e.g. testing the iliopsoas muscle for a grade 2/5 involves asking the patient to flex the hip from an externally rotated position; see Table 1.2 for some general rules about ASIA motor testing). In addition, there are a few complexities in the ASIA motor assessment. For example, grade 1/5 strength in the upper limbs is tested in the gravity-eliminated position whereas in the lower limbs it is tested in the anti-gravity position, except in the case of the plantarflexor muscles (see Table 1.2).
Table 1.2
Ten rules for ASIA motor testing
1 | Test for a grade 3/5 first, then test up or down depending on findings |
2 | Only downgrade strength if due to neurological deficit. Patients unable to fully cooperate due to pain should not be downgraded |
3 | Do not test if the patient has a serious contracture (50% or more loss of joint mobility), severe pain or severe spasticity (mark as not testable) |
4 | Test for a grade 1/5 in the upper limbs with the body segment in its gravity-eliminated position |
5 | Test for a grade 1/5 in the lower limbs with the body segment in its anti-gravity position (except when testing the plantarflexor muscles) |
6 | Test for a grade 4/5 in the upper and lower limbs with the body segment in the anti-gravity position (except when testing T1 and SI muscles) |
7 | Do not use half marks, pluses or minuses |
8 | Test for grade 2/5, 3/5, 4/5 or 5/5 by asking the patient to move all the way through range. Grade 4/5 and 5/5 should be tested with resistance applied throughout range |
9 | Ensure that the patient is not performing trick movements (e.g. ensure grade 3/5 elbow extension occurs without the shoulder dropping into extension) |
10 | Modify testing positions if the patient has loss of extensibility in underlying muscles that span two or more joints (e.g. finger flexion can be tested with the wrist flexed if the patient has shortening of the extrinsic finger flexor muscles) |
It is possible to sum the motor scores of the five key ASIA upper limb muscles on both sides of the body and express the total with respect to a maximum possible score of 50. The same can be done for the lower limbs.21
The ASIA sensory level
A sensory assessment is used to define two sensory levels: one for the right and one for the left side of the body. An ASIA sensory assessment involves testing light touch and pinprick sensation in 28 key points on each side of the body. Each point represents one dermatome (see Figure 1.5). For example, a precise spot over the back of the hand and just distal to the metacarpophalangeal (MCP) joint of the third digit represents the C7 dermatome. A three-point scale is used for light touch and pinprick where normal sensation is given a score of 2, abnormal (i.e. heightened or reduced) sensation is given a score of 1, and absent sensation is given a score of 0 (see Table 1.3).
Table 1.3
Definitions of ASIA sensory scores for pinprick and light touch
Grade 0 | |
Light touch | the patient cannot consistently distinguish between being touched and not touched with a light cotton bud |
Pinprick | the patient cannot consistently distinguish between being touched with the sharp end of a safety pin and touched with the blunt end of a safety pin |
Grade 1 | |
Light touch | the patient can consistently distinguish between being touched and not touched with a light cotton bud but light touch feels different from light touch on the face (this comparison is tested at each dermatome) |
Pinprick | the patient can consistently distinguish between being touched with the sharp end of a safety pin and touched with the blunt end of a safety pin BUT the sharp side of the pin feels different from the sharp side of the pin on the face (this comparison is tested at each dermatome) |
Grade 2 | |
Light touch | the patient can consistently distinguish between being touched and not touched with a light cotton bud AND light touch feels the same as light touch on the face (this comparison is tested at each dermatome) |
Pinprick | the patient can consistently distinguish between being touched with the sharp end of a safety pin and touched with the blunt end of a safety pin and the sharp side of the pin feels the same as the sharp side of the pin on the face (this comparison is tested at each dermatome) |
The ASIA neurological level
The ASIA motor and sensory assessment is also used to depict one overall neurological level.19 This is relatively straightforward in patients who have the same motor and sensory levels on both sides of the body. In this situation, the neurological level corresponds with the motor and sensory levels. However, in patients who have asymmetrical lesions, the highest motor or sensory level on either side of the body is used to define the neurological level of the lesion. For instance, a patient with a right sensory level of C5 but bilateral motor and left sensory levels of C6 has an overall neurological level of C5.
The ASIA Impairment Scale
1. Motor function in S4–S5. This is reflected by the ability to voluntarily contract the anal sphincter.
2. Sensory function in S4–S5. This is reflected by appreciation of deep anal pressure or preservation of either light touch or pinprick sensation in the perianal area.
3. Strength in muscles below the motor and neurological level.
The importance of the S4–S5 segments is linked to prognosis. Its preservation is a strong predictor of neurological recovery.22 Likewise preservation of pinprick sensation anywhere on the body helps predict motor recovery (this is thought to be due to the proximity of the spinothalamic and corticospinal tracts).23
The definition of each ASIA impairment is:
ASIA A: no motor or sensory function in S4–S5.
ASIA B: preservation of sensory function in S4–S5.
ASIA C: preservation of sensory function in S4–S5 provided there is also motor function more than three levels below the motor level OR just preservation of motor function in S4–S5. In addition, less than grade 3/5 strength (i.e. grades 0/5–2/5) in more than half the key muscles below the neurological level.
ASIA D: preservation of sensory function in S4–S5 provided there is also motor function more than three levels below the motor level OR preservation of motor function in S4–S5. In addition, grade 3/5 or more strength (i.e. grades 3/5–5/5) in at least half the key muscles below the neurological level.
Common patterns of neurological loss with incomplete lesions
There are some common patterns of neurological loss with incomplete spinal cord injury. These are:
Sacral sparing. Sacral sparing occurs when the peripheral rim of the spinal cord is preserved. This can happen in vascular injuries when the small radicular arteries supplying the outer rim of the spinal cord are preserved. Consequently, motor and sensory pathways to the sacral segments remain intact and the patient retains sacral sensation, voluntary anal control and, possibly, toe movement (see Figure 1.3).
Brown-Sequard lesion. Brown-Sequard lesions occur when one side of the spinal cord is damaged (i.e. lateral hemi-section). They are usually due to penetrating injuries such as gunshot or knife injuries and account for only 2–4% of all spinal cord injuries. The consequence of damage to half the spinal cord is loss of proprioception and motor function on the same side as the injury and loss of pain and temperature sensation on the opposite side. The pattern of neurological loss is due to the crossing of different motor and sensory pathways within the spinal cord (see p. 4–6). For example, most fibres carrying pain and sensation cross at or near the level they enter the spinal cord. In contrast, fibres carrying motor and proprioception cross in the brainstem.
Central cord lesion. Central cord lesions commonly occur following hyperextension injuries of the cervical spine in older people with cervical spondylosis. The hyperextension injury causes compression, hypoxia and haemorrhage of the central grey matter of the cord, although the peripheral rim of the spinal cord remains intact. Typically, a patient with a cervical central cord lesion has more severe paralysis of the upper limbs than of the lower limbs. This is because the cervical motor tracts are centrally located while the lumbar and sacral tracts are more peripheral (see Figure 1.3). Quite often a mixed lesion occurs combining features of central cord and Brown-Sequard lesions.
Upper and lower motor neuron lesions
Injuries above the conus are predominantly upper motor neuron lesions. Spinal cord-mediated reflexes remain intact and consequently the lesion results in a spastic paralysis. The exceptions are spinal cord injuries which are associated with extensive ischaemic damage. In these types of injuries the anterior horn cells of lower motor neurons are damaged over many segments and sometimes down the entire length of the spinal cord. Similarly, it is common for the anterior horn cells of lower motor neurons to be damaged at the site of the injury.24,25 In this latter scenario, the patient has quite specific and isolated damage of the lower motor neurons associated with the level of the injury although the spinal cord injury is predominantly an upper motor neuron lesion. For example, a patient with a motor C6 level may have lower motor neuron damage of the C7 and/or C8 myotomes but upper motor neuron damage of all other myotomes below the level of the lesion. The lesion is largely an upper motor neuron lesion although the patient has a flaccid paralysis of one or two myotomes.
Prognosis
Most neurological recovery occurs within the first 2 months after injury although recovery may continue for up to 1 year and occasionally after this.26–29 In patients with complete lesions (i.e. ASIA A), the probability of extensive neurological recovery is low.30 One study indicated that only 6% of patients initially diagnosed with a complete lesion had a motor incomplete lesion 1 year later.31 However, patients with complete lesions often regain one neurological level in the months after injury. For example, an individual presenting with C5 tetraplegia at the time of injury may present with C6 tetraplegia 3 months later. Motor recovery following an incomplete lesion is more common. Approximately 50% of patients initially diagnosed with ASIA B or C lesions improve over the first few months by one ASIA level (i.e. from ASIA B to ASIA C, or from ASIA C to ASIA D). It is less common for patients with ASIA D to fully recover (i.e. to ASIA E).29,32
It is difficult to predict patients’ ability to walk at the time of injury but the best estimates indicate that very few patients with ASIA A lesions at the time of injury ultimately ambulate with or without assistance, 30–45% of patients with ASIA B lesion ambulate for at least short distances and most patients with ASIA C and D lesions become community ambulators.33–35 Patients with Brown-Sequard or cervical central cord syndrome have a reasonably good prognosis for walking but not if they are elderly (see Chapter 6).33,36
Impairments associated with spinal cord injury
Vertebral damage and instability
Instability of the vertebral column is generally managed in one of two ways. The first approach is conservative and involves immobilizing the spine for a period of 6–12 weeks. Sometimes this is done with extensive bracing such as can be provided with a halo-thoracic jacket and patients are mobilized in a wheelchair relatively soon after injury. More commonly, patients are confined to bed for 6–12 weeks. During this period the spine is immobilized with skeletal traction (for cervical lesions) or with some type of pillow wedge (for thoracic, lumbar and sacral lesions). There are tight restrictions placed on therapies which may cause movement at the injury site, and patients are turned and moved only under strict medical supervision. The precise limitations on therapies, such as passive movements and stretches, vary from hospital to hospital depending on medical protocols.37 For example, some hospitals limit hamstring stretches for 2 weeks, and others for 3 months. Similarly, some hospitals place tight restrictions on shoulder movements in people with cervical lesions and others encourage movement from the time of injury. The prolonged bedrest associated with conservative management can cause respiratory complications (see Chapter 11) and pressure ulcers, promote disuse weakness (see Chapter 8) and encourage contractures (see Chapter 9).
Once the spine is deemed stable, the patient is mobilized in a wheelchair, often with a spinal orthosis38 which is worn for a further few months (see Figure 1.6).
The second and more common approach to the management of vertebral damage and instability is surgical. Typically, vertebrae are realigned and fixated with or without spinal decompression. There are many different surgical options.39,40 Patients managed surgically are often permitted to mobilize much more rapidly than those managed conservatively, sometimes within a week or so of surgery. They may or may not require some type of bracing once mobilized (see Figure 1.7). The main implication of this approach for physiotherapy management is that patients are confined to bed for a shorter period, and so experience fewer complications associated with immobilization. On the other hand, anaesthesia depresses respiratory function, increasing risk of respiratory compromise in the days after surgery (see Chapter 11).
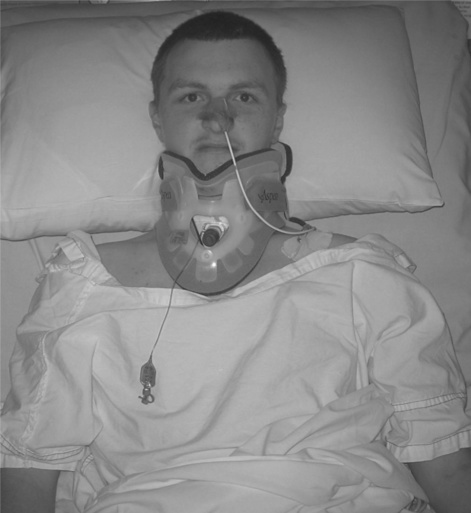
Spinal shock
Immediately after the onset of a spinal cord injury, patients develop a condition called spinal shock.13,41 As the name implies, the spinal cord has an acute reaction to the injury and there is a temporary loss of spinal cord-mediated reflexes below the level of the lesion.13 The extent of disruption to reflexes is variable. The precise definition and duration of spinal shock is debated because different reflexes are lost for varying lengths of time and there is not one reflex used to define spinal shock. For instance, reflexes around the ankle, such as the delayed plantar response, are often only lost for 1–6 hours after injury, while those associated with bladder and bowel function can be lost for many months.41 Some clinicians define spinal shock solely by the absence of deep tendon reflexes42 (typically lost for several weeks) while others define it by the loss of the bulbocavernosus reflex (a reflex associated with anal function which is typically lost for 1–3 days).43 For a long time it was believed that caudal reflexes returned before cephalad ones, with the bulbocavernosus reflex (S4 to S5) being one of the first to return.13 However, this has been disputed.44 It is now generally agreed that spinal shock gradually resolves in a series of stages lasting from a few days to a few months.41
As spinal shock resolves patients with upper motor neuron lesions gradually develop spasticity. The development of spasticity is not solely due to the resolution of spinal shock but may also be due to associated neurophysiological and physical changes.13,41 The development of spasticity has important implications for physiotherapy management and especially for the management of contractures (see Chapter 9).
Paralytic ileus
The development of a paralytic ileus is associated with spinal shock. Like spinal shock, this condition presents immediately after injury and can last from a few days to a few weeks. The main consequence of a paralytic ileus is that food cannot be digested and, if untreated, patients develop abdominal distension and may vomit. The distended abdomen increases the work of breathing, and vomiting heightens the risk of aspiration pneumonia (see Chapter 11). Typical management includes ‘nil by mouth’. A nasogastric tube is inserted to regularly aspirate stomach contents (the tube is not for feeding). Nutrition and fluids are provided intravenously.
Deep venous thrombosis and pulmonary embolus
Patients are particularly vulnerable to deep venous thromboses during the first 2 weeks after injury. During this period the dislodgement of deep venous thromboses is one of the leading causes of death.45–50 Deep venous thromboses are caused by stasis of blood within the venous system that results from paralysis and lack of movement. The stasis is exacerbated by bedrest and lack of vasomotor control in people with lesions involving the sympathetic nervous system.47,51 Deep venous thromboses are particularly common in the veins of the calf but potentially more dangerous in the veins of the thigh and groin. The signs of deep venous thromboses are low grade fever and localized swelling, warmth and discolouration.46 Pain may be present in patients with intact sensation. Definitive diagnoses are based on the results of impedance plethysmography, ultrasound or venography.46
The dislodgement of deep venous thromboses can result in pulmonary emboli, which are life-threatening. The presence of emboli is characterized by any number of the following symptoms: loss of consciousness, shortness of breath, hypoxia, sweating, haemoptysis, tachycardia, confusion or chest pain. Sometimes the first sign of an embolus is respiratory or cardiac arrest.46 Deep venous thromboses are particularly likely to be dislodged when patients are moved for routine care or when patients’ limbs are moved during passive movements. Therefore, if deep venous thromboses are suspected or diagnosed, movement of the patient should be kept to a minimum and passive movements of the legs should cease.52
To prevent deep venous thromboses during the period immediately after injury, patients are routinely placed on anticoagulation medication,47,53,54 provided with tight-fitting stockings, regularly screened for deep venous thromboses, and mobilized as soon as feasible. External pneumatic compression devices46,54–56 and electrical stimulation have also been advocated.47 For a long time it was believed that passive movements prevented deep venous thromboses, although this is now disputed46 (see Ref. 57 for clinical guidelines on prevention of thromboembolism). Deep venous thromboses and pulmonary emboli are treated with thrombolytic agents or low molecular weight heparin, e.g. enoxaparin.58
Spasticity
Spasticity is present in up to 80% of patients with spinal cord injury.59 It is only present in patients with intact lower motor neurons so is not present in patients with cauda equina injuries. Spasticity is more troublesome for patients with incomplete rather than complete lesions,60 and tends to gradually increase over the first year before it plateaus. The increase may be due to neural sprouting or changes in the sensitivity of neural receptors. Spasticity can be elicited with many stimuli but stretch and touch are the most common.61 Sudden increases in spasticity are usually indicative of illness or injury, or an over-distended bladder or bowel.59 Many tests62,63 are used to quantify spasticity but the two most widely used are the Tardieu Scale64 and the Modified Ashworth Scale65–67 (see Table 1.4). The usefulness of these and other tests of spasticity is widely debated.68,69
Table 1.4
0 | No increase in muscle tone |
1 | Slight increase in muscle tone, manifested by a catch and release or by minimal resistance at the end of range of motion when the affected part(s) is moved in flexion or extension |
1 + | Slight increase in muscle tone, manifested by a catch, followed by minimal resistance throughout the remainder (less than half) of the range of motion |
2 | More marked increase in muscle tone through most of the range of motion but affected part(s) easily moved |
3 | Considerable increase in muscle tone, passive movement difficult |
4 | Affected part(s) rigid in flexion or extension |
Reproduced from Bohannon RW, Andrews AW: Interrater reliability of hand-held dynamometry. Phys Ther 1987; 67:931–933, with permission of the American Physical Therapy Association.
The neurophysiology of spasticity is complex and not fully understood (see Refs 59, 68, 70, 71 for comprehensive overviews). It can have many different features but the two key ones are an abnormal and velocity-dependent increase in resistance to stretch.70 Spinal cord injury changes the excitability of the tonic and phasic stretch reflexes, which are controlled by the balance of excitatory and inhibitory inputs onto alpha motor neurons. These inputs arise from a large number of segmental and descending neural circuits. There are many theories about precisely how and to what extent these circuits are disrupted, and which disruption is most important.72 Until recently it was believed that spasticity primarily resulted from unchecked hyperactivity of gamma motor neurons, driving intrafusal muscle fibres and increasing reflex facilitation of alpha motor neurons. However, the importance of gamma motor activity has been disputed,61 and spasticity is now believed to be due primarily to a direct increase in the excitability of the alpha motor neurons. This may be related to enhanced sensitivity of the alpha motor neurons.13,61 The increased excitability of the alpha motor neurons is also thought to be due to a loss in the normal dampening effects of the descending fibres of the corticospinal tract. With less dampening, the alpha motor neurons are more excitable and responsive to sensory inputs. The alpha motor neurons of antagonistic muscles are also easily excited because there is a loss of activity in the cells which are responsible for reciprocal inhibition (i.e. Renshaw cells).
Spasticity is primarily managed with pharmacological agents.73 There are two main categories of drugs, some acting predominantly within the central nervous system (e.g. baclofen, diazepam, gabapentin, clonidine, tizanidine) and others acting peripherally, either within the muscle or at the neuromuscular junction (e.g. dantrolene and Botulinum toxin). Severe spasticity is sometimes managed by administering drugs directly to the spinal cord (i.e. intrathecally).
The main implications of spasticity are that it predisposes patients to pain, contractures and pressure ulcers, and makes movement and hygiene difficult.59,61,68 For some patients, spasticity limits function and quality of life.74 Physiotherapy interventions such as hydrotherapy, stretch, heat, TENS, cold, electrical stimulation, therapeutic exercise techniques, passive movements, standing and vibration may provide transient relief from spasticity, but there is no evidence that any of these interventions produce lasting reductions in spasticity.73,75 In addition, the application of heat can cause burns and therefore should only be used with extreme caution in patients who lack sensation.
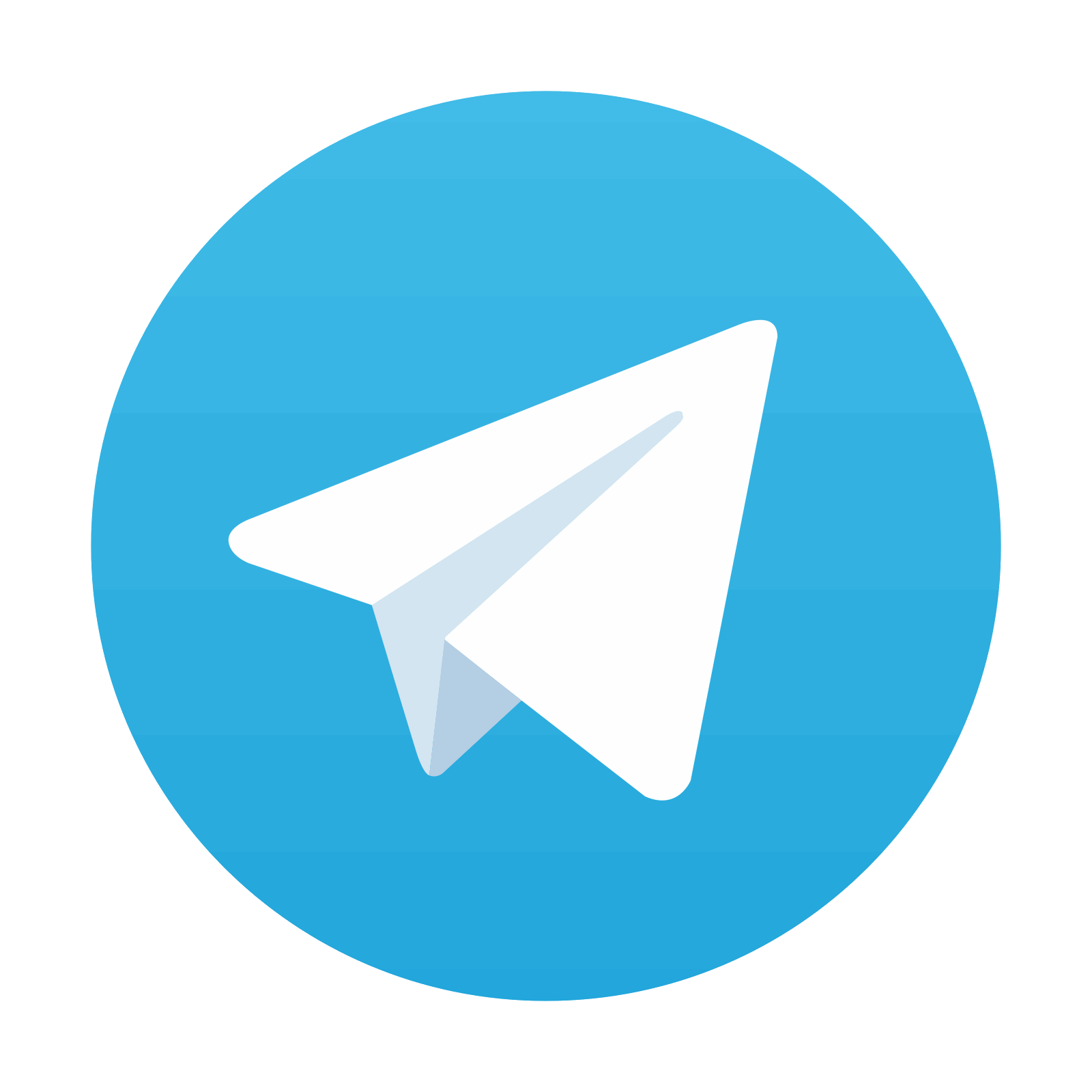
Stay updated, free articles. Join our Telegram channel

Full access? Get Clinical Tree
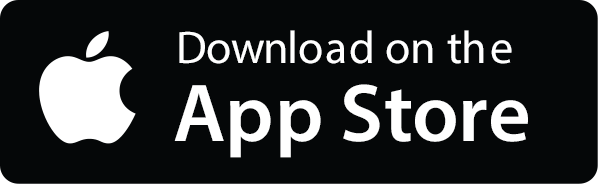
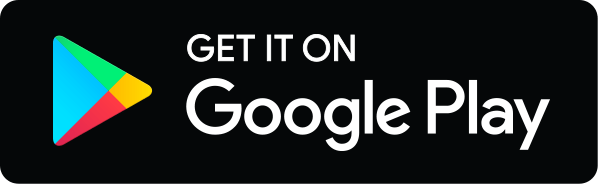