Fig. 16.1
Schematic representation of the innervation of normal (top) and degenerated (bottom) intervertebral discs (IVDs), as well as the origin of sensory nerve fibers that innervate them. In the normal IVD, innervation is restricted to the outer layers of the annulus fibrosus (AF) and consists of small nerve fibers (red and green) and some large fibers forming mechanoreceptors (brown). In the degenerated IVD, nerve fibers are increased in number, and they enter the inner layers of the AF and even the nucleus pulposus (NP). Furthermore, in these conditions, the density of mechanoreceptors in the superficial layers of IVDs is increased. Dorsal root ganglions (DRGs) contain different types of sensory neurons that project to the IVD and to the dorsal horn of the spinal cord (DH of SC). Thin myelinated Ad fibers and unmyelinated C fibers arise from small neurons (red and green), which, in the spinal cord, synapse in laminas I and II and mediate nociception. The myelinated Ab fibers (brown) arise from intermediate neurons; at the periphery, they form slowly and rapidly adapting low-threshold mechanoreceptors and synapse in laminas III and IV in the dorsal horn of the spinal cord; they mediate sensations of touch, pressure, and vibration. Most of the sensory nerve fibers innervating the IVD are Ad or C fibers. They originate from small peptidergic neurons expressing TrkA⁄TrkB (the receptor for nerve growth factor/brain-derived neurotrophic factor, red) or non-peptidergic neurons expressing the common signaling receptor for glial cell-derived neurotrophic factor family of neurotrophic factors (Ret) (red). Neurons in DRGs can be differentiated based on their pattern of expression of receptors for neurotrophic factors, pattern of expression of different ion channels primarily of the degenerin⁄epithelial sodium channels (DEG⁄ENaCs) (ENaCa, ENaCb, and ENaCc), acid-sensing ion channel (ASIC) (ASIC1, ASIC2, and ASIC3) and transient receptor potential (TRP) (TRPA1, TRPC1, TRPC6, and TRPV1–4) families, and peptide content. CGRP calcitonin gene-related peptide, GFRa1 and GFRa3 glial cell line-derived neurotrophic receptor subtypes a1 and a3, P2X3 ATP-gated ion channel subtype P2X3, SP substance P, TMP thiamine monophosphatase, VR1 vanilloid receptor subtype 1 (Modified from Garcia-Cosamalon et al. (2010))
The overall conflict with the hypothesis that changes in the intervertebral disc would transmit pain to the central nervous system is the observation that the innervation is located only in the superficial layers of the annulus fibrosus (Malinsky 1959; Hirsch et al. 1963; Groen et al. 1988; Ashton et al. 1994; Roberts et al. 1995; Palmgren et al. 1999; Fagan et al. 2003). This is the case in normal nondegenerated discs. On the other hand, degenerated disc specimens exhibit ingrowths of newly sprouted nerves into the deeper portions of the disc. However, this study was performed mainly on the anterior portion of the intervertebral disc, and ingrowths were only demonstrated in the inner part of the annulus fibrosus and, in some cases, possibly extending into the nucleus pulposus (Yoshizawa et al. 1980; Ashton et al. 1994; Coppes et al. 1997; Freemont et al. 1997; Johnson et al. 2001). A later study acknowledged that the posterior region of the disc might be more relevant, and indeed an ingrowth of nerves positive for substance P and VIP was noted extending to the outer part of the nucleus pulposus (Peng et al. 2005). Since this ingrowth was always present when there was granulation tissue and annular fissures, the authors concluded that the zone of granulation may be responsible for discogenic pain due to neo-innervation of the disc and possibly a cause of pain from discography (Peng et al. 2005). It was also recently demonstrated that when autologous nucleus pulposus tissue fragments were placed subcutaneously in pigs, there is an ingrowth of newly formed nerves and blood vessels. In contrast, similar ingrowth were not seen when disc tissue was placed in retroperitoneal fat. It was also observed that cytokine inhibitors reduced this ingrowth (Olmarker 2005). It has also been suggested that bFGF and TGF-β1 as well as macrophages and mast cells are involved in the ingrowth of nerves and blood vessels into the intervertebral disc and that this phenomenon is related to the injury repair of the annulus fibrosus (Peng et al. 2006a).
Semaphorin is an axonal guidance molecule that serves to repel axonal growth (Rohm et al. 2000; Nakamura et al. 2000; Liu and Strittmatter 2001). Recently, it was found that this molecule is present in the healthy intervertebral disc, mainly in the outer annulus (Tolofari et al. 2010), where it presumably could prevent axonal ingrowth. Even more interesting is that the same authors found that the levels of semaphorin are lowered in degenerate discs, which would then enhance neo-innervation (Tolofari et al. 2010). Recently, it was suggested that neurotrophins family members were involved in the ingrowth of nerves into the central part of the intervertebral discs (Purmessur et al. 2008; Garcia-Cosamalon et al. 2010). In the human, this family comprises NGF, BDNF, NT-3, and NT-4/5 (Ebendal 1992; Barbacid 1995; Lessmann 1998). The neurotrophins regulate cell proliferation and differentiation via the Trk family of receptor tyrosine kinase and via p75-NTR (Dechant and Barde 1997; Lu et al. 2005; Skaper 2008). Since the neurotrophins seem to be expressed at higher levels in degenerate than in nondegenerate discs, it may be assumed that they may facilitate ingrowth of new nerve fibers into the deeper parts of the intervertebral disc during the degeneration process (Freemont et al. 2002). In summary, based on existing knowledge, there is indeed reason to assume that there is neo-innervation and neovascularization of the annulus fibrosus and nucleus pulposus and that such ingrowth relates to the presence of bioactive substances generated during repair following annular fissures.
Although there may be neo-innervation of injured intervertebral discs, the function and properties of such newly formed nerves are not known (Fig. 16.1). The normal innervation of the disc comprises a mixture of fibers that are nociceptive, related to mechanoreceptors, and sympathetic in nature (reviewed in Garcia-Cosamalón) (Garcia-Cosamalon et al. 2010). In this regard, the disc nerve fibers are positive for PGP 9.5, substance P, calcitonin gene-related peptide (CGRP), acetylcholinesterase, vasoactive intestinal peptide (VIP), neuropeptide Y, C-flanking peptide, and synaptophysin (Garcia-Cosamalon et al. 2010). Interestingly, following degenerate disc injury and repair, the nerve fibers that penetrate deep into the annulus fibrosus, and later even further, appear to exhibit a similar distribution of fiber types (Takahashi et al. 2009). As neo-innervation and the neovascularization occur in parallel, it has been suggested that newly formed nerve fibers are mainly vasoregulatory in function (Freemont et al. 1997, 2002; Johnson et al. 2001, 2007). However, nerve endings not related anatomically to newly formed vessels have also been identified (Ashton et al. 1994). In addition, since the peptide content of the nerve fibers found in degenerate intervertebral discs includes those related to pain transmission and since the nerves related to blood vessels seem to be of sympathetic origin (Yamada et al. 2001), the possibility exists that there are nerve fibers that may be involved in nociception.
Based on what was considered above, it begs the question: are nerve fibers with peptides related to nociception activated in the center of the intervertebral disc, or is their presence merely accidental and that they are dormant? Most innervation studies of intervertebral discs have reported the presence of “nerve endings” or axons (McCarthy et al. 1991; Palmgren et al. 1999; Fagan et al. 2003; Aoki et al. 2004), and the only receptor types are mechanoreceptors (Roberts et al. 1995; Dimitroulias et al. 2010; Cavanaugh et al. 1995). The receptors are always found in the superficial layers of the annulus (Roberts et al. 1995; Cavanaugh et al. 1995). However, it is questionable if the mechanoreceptors could produce pain; thus, induced pain is probably related to direct effects on axons or so-called free nerve endings in the intervertebral disc.
It is known that during degeneration of the nucleus pulposus there are a number of biochemical changes. One such change is the accumulation of metabolites such as lactate and the concomitant pH decrease (Diamant et al. 1968; Nachemson 1969; Buckwalter 1995; Bartels et al. 1998; Keshari et al. 2008; Rajasekaran et al. 2010). Accordingly, pain could be due to chemical excitation of adjacent nerve fibers. Similarly, the increased expression of various bioactive substances, such as members of the neurotrophin family, may activate pain receptors (Freemont et al. 2002; Purmessur et al. 2008; Sugiura et al. 2008; Garcia-Cosamalon et al. 2010; Orita et al. 2011). Biomechanical instability of the motion segment may lead to excess mobility of the degenerated intervertebral disc. Likewise, it has been suggested that such mobility may actively induce signaling in newly formed nerve fibers in the deeper parts of the intervertebral disc, another plausible cause for “disc-triggered” pain (Morgan and King 1957; Kirkaldy-Willis and Farfan 1982; Pope and Panjabi 1985; Bradford 1994; Kim et al. 2005). Finally, injured axons are known to be stimulated by the nerve signals from adjacent axons, so-called cross-excitation (Rasminsky 1987; Lisney and Devor 1987; Devor and Wall 1990; Amir and Devor 1992). Injury can cause a myelin defect due to the loss of its electro-isolating properties: an electrical phenomenon in which nerve impulses in one axons cause an impulse in adjacent axons. Such artificial “ectopic” impulses may be interpreted as pain by the central nervous system, regardless of the axon that originally transmits proprioceptive, temperature, or pressure information (Burchiel 1984; Zimmermann 1984; Devor 1991, 2006; Han et al. 2000; Costigan et al. 2009). Whether this occurs in the intervertebral disc seems unlikely due to its sparse innervation.
In summary, in recent years, knowledge of intervertebral discs innervation has increased tremendously, and the possibility exists that there is “disc-triggered” or “discogenic” pain. However, pain mechanisms still need to be demonstrated convincingly before any conclusions can be drawn as to its clinical relevance.
16.5 Degeneration of the Intervertebral Disc: A Normal Aging Process?
The intervertebral discs are usually referred to as the largest avascular structures of the human body (Holm et al. 1982; Horner and Urban 2001; Roberts 2002; Grunhagen et al. 2011). The nutrition of the cells of the discs, mainly located in the nucleus pulposus, is mainly provided by diffusion from the vertebral end plates. Accordingly, if the nutritional supply becomes insufficient, the nucleus pulposus may undergo changes that may be referred to as age-related or degenerative. This process may be initiated as early as the second decade of life, and its frequency will increase with increasing age (Takatalo et al. 2009; Samartzis et al. 2011). There are reports that 40 % of individuals aged 30 years, 53 in the age group 30–55 years, and 90 % aged 50–55 years exhibit degeneration of one or more discs (Kanayama et al. 2009; Cheung et al. 2009). Degeneration would be expected to lead to changes in the biochemical composition of the disc as well as changes in its mechanical properties. The nucleus pulposus will be more liquefied and there may also be a weakening of the containing structure, the annulus fibrosus. Eventually, the disc will form a more solid and organized structure, which is also usually associated with a reduction of the disc height (Burton et al. 1996; Benneker et al. 2005; Inoue and Espinoza Orias 2011). During the transition from a young healthy disc to the more solid state, there is risk of leakage of the liquefied nucleus pulposus with its bioactive components through fissures in the weakened annulus fibrosus into the spinal canal. Similar leakage may also occur on the ventral aspect of the disc and also through the vertebral end plates into the adjacent vertebral bodies (nodes of Schmorl) (Schmorl 1929). What is not known is whether these variants of leakage/herniation produce clinical symptoms (Sward et al. 1990; Takahashi et al. 1995; Zhang et al. 2010). More importantly, the question remains, should these changes in disc structure be considered as normal aging, or should they be described as a degenerative process?
As discussed previously, the term degeneration may be related to the aging process, and hence, the pathophysiological connotation cannot be ignored. Indeed, if the aging processes are shown to induce pain, it might rightfully be termed degeneration. However, signs of spinal pathology, including so-called disc degeneration, are often encountered in asymptomatic individuals (Boden et al. 1990; Jensen et al. 1994; Boos et al. 1995; Stadnik et al. 1998). In this regard, it might be more appropriate to talk about normal and pathological/symptomatic disc aging instead of degeneration. It is thus still not evident how to apply a proper definition to this state. However, according to this author, the term degeneration may be unfortunate and misleading, particularly in persons without any symptoms.
16.6 LBP and Disc Degeneration in Clinical Studies
The literature suggests that there is a strong correlation between degenerative disc changes and LBP (Lindblom 1948; Kelsey and White 1980; Lutz et al. 2003; Chou et al. 2011). However, since no causal link has been confirmed and since a majority of non-symptomatic patients have degenerative disc changes, a causal relationship may be unlikely. This is analogous to the recent discussion on the relationship between Modic-type changes in the vertebrae and LBP. Similar to disc degeneration, there is a correlation between Modic changes and low back clinical pain (Braithwaite et al. 1998; Kjaer et al. 2005; Jensen et al. 2008; Thompson et al. 2009). Again, there is no established causal pathophysiological link. One must therefore consider that there may be a transferred statistical significance, i.e., the Modic changes and low back pain may coexist and have a common pathogenic source but no peer relationship. One such pathogenetic factor might be disc injury. For example, it is known that osteoarthritis in the knee joint may result in changes, fairly similar to the Modic changes, in the femur and that these changes/erosions are considered to be strong indicators of knee cartilage injury (Alexander 1960; Rose and Cockshott 1982; Pattrick et al. 1993). As will be discussed later, disc injury with annular tears and leakage of liquefied nucleus pulposus tissue to the superficial and innervated part of the annulus fibrosus could be one cause of low back pain. In this regard, disc injury would be the “common denominator,” and an apparent statistical relationship would exist although Modic changes and low back pain merely coexist with no pathophysiologic link. The same phenomenon may be applied to the relationship between disc degeneration and LBP. Since no causal link has been established, there may be another “common denominator” perhaps in this case, secondary to changes in the intervertebral disc.
16.6.1 Box 16.1 Modic Changes
The so-called Modic changes were first described by Michael Modic and collaborators in 1988 (Modic et al. 1998). Magnetic resonance images of vertebral body changes were reviewed of 474 patients. It was found that 4 % of the patients had decreased signal intensity on T1-weighted spin-echo images and increased signal intensity on T2-weighted images, a finding that was given the grade 1. In 16 % of the patients, there was increased signal intensity on T1-weighted images and isointense or slightly increased signal intensity on T2-weighted images, which was graded as 2. A third grade was added later and is defined as bone scar tissue. The authors concluded, “These signal intensity changes appear to reflect a spectrum of vertebral body marrow changes associated with degenerative disk disease.” Today it is known that these changes closely correlates to low back pain (Braithwaite et al. 1998; Kjaer et al. 2005; Jensen et al. 2008; Thompson et al. 2009). However, no causal pathophysiological explanation has been found. It is therefore a possibility that there might be a transferred statistical significance, i.e., the Modic changes and low back pain may coexist but have a common pathogenic source and no peer relationship. One such likely source might be disc injury. It is known that there is an increased incidence of Modic changes in patients with disc herniations (Albert and Manniche 2007). If the Modic changes might be responsible for low back pain or not is thus debatable, but it seems plausible that these changes may serve as an indicator of disc injury.
References
Albert HB, Manniche C (2007) Modic changes following lumbar disc herniation. Eur Spine J 16:977–982
Braithwaite I, White J, Saifuddin A et al (1998) Vertebral end-plate (Modic) changes on lumbar spine MRI: correlation with pain reproduction at lumbar discography. Eur Spine J 7:363–368
Jensen TS, Karppinen J, Sorensen JS et al (2008) Vertebral endplate signal changes (Modic change): a systematic literature review of prevalence and association with non-specific low back pain. Eur Spine J 17:1407–1422
Kjaer P, Leboeuf-Yde C, Korsholm L et al (2005) Magnetic resonance imaging and low back pain in adults: a diagnostic imaging study of 40-year-old men and women. Spine (Phila Pa 1976) 30:1173–1180
Modic MT, Steinberg PM, Ross JS et al (1998) Degenerative disk disease: assessment of changes in vertebral body marrow with MR imaging. Radiology 166:193–199
Thompson KJ, Dagher AP, Eckel TS et al (2009) Modic changes on MR images as studied with provocative diskography: clinical relevance – a retrospective study of 2457 disks. Radiology 250:849–855
16.7 Secondary Changes of Disc Degeneration That May Induce Pain
About 80 % of the population worldwide will experience LBP sometime during their lifetime (Andersson 1995, 1999). However, in only a minority of cases, a definitive clinical diagnosis such as vertebral fracture, infection, or tumor will be established (Staiger et al. 1999; Goupille et al. 2000; Della-Giustina and Kilcline 2000; Henschke et al. 2009). The remaining 95 % will therefore be termed idiopathic. Most likely, there may be one or more specific mechanisms that may be hidden within these 95 % that have yet to be discovered. Although events occurring within the disc might theoretically induce LBP (discogenic LBP), the degeneration of the intervertebral disc may also cause secondary changes that could be overlooked.
One such effect is the subsequent reduction of the disc height during the degeneration process. A consequence of this lowered height is that there may be malpositioning of the facet joints (Ghomley 1993; Eisenstein and Parry 1987; Mooney and Robertson 1976). Theoretically such a change could lead to cartilage injury and damage to the capsule facet joint (Fujiwara et al. 2000a, b; Kong et al. 2009). It is known that the facet joints are richly innervated (Bogduk and Long 1979; Bogduk 1983), and attempts have been made to treat facet-joint-related problems with local injections of anesthetics (Carrera 1980; Manchikanti et al. 2007, 2008). However, it is likely that osteoarthritis of these joints and the ensuing production of inflammatory agents that can leak through the injured joint capsule could serve to irritate and stimulate intraspinal nervous structures including the nerve root and nerve endings on the posterior aspect of the intervertebral disc (Hasue 1993; Willburger and Wittenberg 1994; Igarashi et al. 2004).
Another striking feature of the degenerative changes of the intervertebral discs is the formation of annular tears or fissures (Hilton et al. 1980; Videman and Nurminen 2004; Ross et al. 1989; Osti et al. 1992). Such fissures may allow leakage of the degenerated and partly “liquefied” nucleus pulposus (Stadnik et al. 1998; Saifuddin et al. 1999; Derby et al. 2005; Peng et al. 2006b). Biologic product from the degenerative nucleus pulposus could diffuse to the outer annulus fibrosus or even the spinal canal. MRI analysis has shown that a zone of increased inflammatory activity, the high-intensity zone (HIZ), exists in the superficial regions of the annulus (Schellhas et al. 1996; Peng et al. 2006b; Carragee et al. 2000). This zone is associated with LBP although it may also be found in asymptomatic patients (Weishaupt et al. 1998; Stadnik et al. 1998; Carragee et al. 2000; Wang et al. 2008).
It is known that nerve endings and mechanoreceptors exist on the posterior aspect of the annulus fibrosus; while these are normally dormant, they can be activated by inflammatory stimuli (Rang et al. 1991; Dray 1995; Ozaktay et al. 1994; Cavanaugh 1995; Coutaux et al. 2005). It is therefore reasonable to assume that biologically active substances released from the degenerating nucleus pulposus may activate cognate receptors by diffusing into the spinal canal through the annular fissures. One diagnostic approach for assessing symptomatic intervertebral discs is through discography, a procedure in which a fluid is injected under pressure into the center of the disc to provoke pain (Moneta et al. 1994; Schellhas et al. 1996; Carragee 2000; Stout 2010). Possibly, the pain is not related to activation of newly formed nerves within the intervertebral disc, but to a washout of bioactive substances into the spinal canal and the innervated structures including the disc, the longitudinal ligament, and facet-joint capsules.
In a pioneering study, in patients undergoing surgery for disc herniation under local, progressive anesthesia, Kuslich and colleagues stimulated various parts of the spinal complex (Kuslich et al. 1991). They found that stimulation of a nerve root did not generate pain, whereas mechanical stimulation of the nerve root adjacent to the herniated disc produced a radiating pain out into the lower limb, similar to sciatic pain. The most interesting observation, however, was that stimulation of the posterior part of the annulus fibrosus produced pain in the lower back, similar to LBP. The authors concluded that the posterior annulus fibrosus was “the site of back pain” (Kuslich et al. 1991). There is thus compelling evidence that activation of nociceptive nerve endings or receptors in the posterior aspect of the annulus fibrosus (Weber et al. 2006) may produce pain in the lower back; this finding may be due to the release of agents from the nucleus pulposus during discography and also possibly at non-provoked sites (Fig. 16.2).
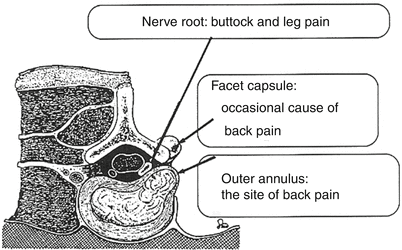
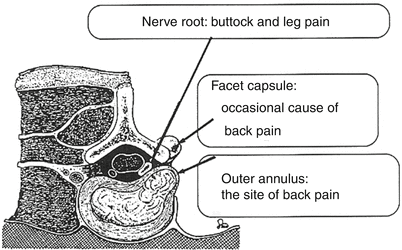
Fig. 16.2
Observations at probing in conscious patients under local anesthesia during laminectomy. Probing of the nerve root adjacent to a disc herniation reproduces buttock and leg pain. Probing of the fact capsule occasionally reproduces back pain, whereas probing of the outer annulus always reproduces back pain and is also concluded to be “the site” of back pain (Republished from Kuslich et al. (1991))
Our group studied whether application of nucleus pulposus samples to the superficial annulus fibrosus might induce LBP in rodents. As discussed in Chap. 18, assessment of LBP in an animal model is a significant challenge. While alterations in specific nerves activity may be assessed by neurophysiologic recordings, pain itself is dependent on its interpretation by the central nervous system. In animals, there is a limitation in available experimental methodologies to assess whether the transmitted signals are perceived as pain. Methods that can be used for assessing pain is through functional MRI (Hsu et al. 1998; Weber et al. 2006; Adamczak et al. 2010) (see Chap. 12); another approach is to study behavioral changes (Kawakami et al. 1994; Abbott et al. 1995; Olmarker and Myers 1998).
In rodents, we have assessed spontaneous behavioral changes following experimental disc herniation (Olmarker et al. 2002). In contrast to most other assessment modalities, spontaneous behavior analysis, which has been used to evaluate psychological parameters (Wuttke and Hoffmeister 1968; Monti and Carlini 1975; Rodriquez-Enchandia et al. 1986; Garcia-Cabrera and Berge 1990), examines an animal’s instinctive involuntary activities. Despite the high variation in behavior between animals, changes in spontaneous behavior may reveal discomfort or irritation that is not easily obtained by other behavior modalities. In collaboration with the Department of Physiology, University of Bergen, Norway, spontaneous behavior assessment was adapted to study changes following experimental disc herniation. Procedures used included disc puncture with transfer of the nucleus pulposus tissue to, and a slight mechanical deformation of, the adjacent nerve root (Olmarker and Myers 1998; Olmarker et al. 1998, 2003). The study showed that experimental disc herniation induced an increased rotation of the head towards the hind paw on the operated side and an elevation in paw lifting (Olmarker and Myers 1998). These two behaviors were most pronounced the day after surgery and then gradually declined during the following 14 days. At day 21, the rats displayed increased “immobility” and reduced “locomotion.” The behavior changes during the first 14 days suggested that following surgery, animals experienced focal pain or irritation located in the hind paw on the operated side. During days 14–21, a more “chronic” non-focal pain component was present and seen as reduced mobility. Using this assessment tool, the authors studied changes induced by disc puncture, thus simulating a leakage of nucleus pulposus onto the posterior aspect of the annulus fibrosus, but with no contact with the adjacent nerve root. The assumption was that the rats would display reduced motion, analogous to LBP, similar to that reported at 21 days in the radiculopathy model. While the rats did not exhibit reduced mobility, they evidenced increased “grooming” and an uncharacteristic shaking of the head and upper body: both behaviors were displayed significantly more often in disc punctured rats than in rats with non-punctured discs (Olmarker 2008). Increased grooming has been observed in other studies and has been suggested to reflect anxiety, stress, and chronic neuropathic pain (Millan and Emrich 1981; Crawley and Moody 1983; Vos et al. 1994; Deseure and Adriaensen 2002; Eriksson et al. 2005). The observed shaking is commonly seen in psychopharmacological studies and termed wet-dog shakes (WDS): it is a typical sign of withdrawal from opiates, benzodiazepines, and barbiturates (Colasanti and Khazan 1975; Baldino et al. 1979; Horowitz and Allan 1982; Martin et al. 1982). The shakes have also been considered to relate to stress (Treptow et al. 1986; Deschamps and Couture 2005; Brotto et al. 1999) and pain (Papir-Kricheli et al. 1987; Kitamura et al. 2007). It was concluded that rats with punctured discs experienced some kind of discomfort. However, it was not possible to determine if this discomfort was analogous to LBP.
In a follow-up study, we evaluated if the observed behavior changes were induced by disc injury or the presence of nucleus pulposus tissue on the posterior aspect of the annulus fibrosus. It was found that disc puncture and application of nucleus pulposus induced similar behavioral changes. In contrast, the same behavioral changes were not evident following ventral disc puncture and a superficial disc injury without penetration of the annulus fibrosus, with no leakage of nucleus pulposus tissue (Olmarker 2011). It was also observed that inhibition of TNF also markedly reduced the behavioral effects (Nakamae et al. 2011). Interestingly, a recent randomized clinical trial focused on evaluating the effects of epidural TNF inhibition on sciatic pain also revealed that in addition to the inhibitors’ beneficial effects on the sciatic pain, it also induced a marked reduction of LBP (Cohen et al. 2009). In summary, there is emerging experimental and clinical evidence that leakage of disc material onto the posterior annulus fibrosus may be an actual cause of LBP possibly mediated through TNF.
16.7.1 Box 16.2 Molecular Events That Are Characteristic of the Aging Process
There is limited information on factors that characterize the normal aging process in the intervertebral disc. However, since the aging disc shares some genetic as well as molecular characteristics of cartilage, changes that are evident in cartilage are listed below:
Mild fibrillation of and softening of the articular surface
Osteophyte formation
Loss of matrix tensile strength and stiffness
Responsiveness to inflammatory stimuli
Changes in metabolism
Accumulation of ROS and glycation products
Changes in IGF, insulin, and growth hormone levels
Progressive chondrocyte senescence
Erosion in of chondrocyte telomere length
Changes in phenotype
Decrease in average size of proteoglycan monomers
Decrease in the aggregation capacity of the proteoglycan monomers
Expression of the senescence-associated enzyme beta-galactosidase
Mitochondrial degeneration
16.8 Therapeutic Strategies: Should the Target Be Nucleus Pulposus Degeneration?
Inevitably, a chapter on disc degeneration and LBP must provide insights into possible therapeutic strategies. Although it is not fully understood if the disc tissue per se can produce clinical pain, the target of much of the work is the degeneration process itself and the disc as a pain generator. Surgically, this has been approached by fusion of the potentially painful disc function unit, a procedure that is frequently performed with varying degrees of success (Gibson and Waddell 2005; Carreon et al. 2008; Glassman et al. 2009). There are also promising attempts to revitalize the disc by injection of stem cells or biological components (Wehling et al. 1997; Nishida et al. 2000; Yoon et al. 2004; Sakai et al. 2003; Brisby et al. 2004; Risbud et al. 2004) (see Chaps. 23 and 24). However, although these procedures might be successful in the future, they do raise a set of new questions. Based on current knowledge, it will be difficult to assess when, how often, and in which discs should such interventions be performed. We know that there are “dangerous years”: from the initiation of degeneration/aging, when the disc may leak, herniate, and produce pain, until the end stage when the disc has been transformed to a more solid connective tissue, with less likelihood to produce pain. A provocative but appropriate question would be: would it not be a better strategy to increase the degeneration/aging process so as to shorten the duration of these “dangerous years”? This notion was suggested 50 years ago by Hirsch who recommended that injection of a “chondrolytic enzyme” into the disc would increase the degeneration process and convert the disc to a solid, asymptomatic structure (Hirsch 1959). This original idea was later modified by Smith who indicated that chymopapain would disintegrate the inner structure of the disc and thus be of clinical use for the treatment of sciatica; this procedure was later known as chemonucleolysis (Smith 1964). Bearing this in mind, it might be prudent to consider the apparent risk of delaying the degeneration/aging process of the disc by biological means, in the sense that instead of having mostly “asymptomatic” discs at the age of 60–70, there is the possibility that “symptomatic” discs may be present at much higher ages than would be expected if the disc would be allowed to age normally. The author opines that this potential problem, a reduction in the degeneration process, has not being fully considered and needs to be further explored.
An alternate strategy to that of modifying the degeneration/aging process per se is to control pain pathways. Since the source of the pain has not been identified, this form of “symptomatic” treatment would be analogous to aiming water at flames and not at the source of the fire. In fact, since it is not evident that the disc generates the pain, secondary changes such as loss of disc height might eventually prove to be involved (Twomey and Taylor 1985; Berlemann et al. 1998; Shao et al. 2002). For example, the loss of disc height could influence facet-joint function. Misalignment of the articular surfaces could induce osteoarthritic changes and the release of bioactive molecules. Diffusion of these agents to the intraspinal nervous structures could then induce pain by similar mechanisms to those discussed previously (Hasue 1993; Willburger and Wittenberg 1994; Igarashi et al. 2004).
Another inevitable consequence of degeneration is disarrangement of the fibrils of the annulus fibrosus and leakage of the partly liquefied degenerate disc. To the author, this seems to be a very likely scenario and could well be the cause of LBP of “idiopathic origin.” Perhaps, in the future, LBP treatment may comprise a dual strategy: epidural administration of agents that inhibit key biological processes and a “sealant” of annular tears, possibly in combination with surgical immobilization of the spinal segment and the preservation of disc height.
16.9 Disc Degeneration and Its Relationship to LBP: Conclusions
Although emerging evidence indicates that degeneration may induce innervation of deeper parts of the intervertebral disc, it seems unlikely that the degenerative process produces pain per se. More likely, it is the result of secondary changes associated with degeneration (Fig. 16.3). As challenging as ever are questions that include whether future treatment strategies should be directed at the degeneration process itself, what is the importance of controlling secondary changes, and should the disc be rejuvenated and when and how should this be accomplished. While this chapter is both speculative and somewhat provocative, it is merely the result of the apparent lack of understanding of the pathophysiologic background of LBP, and it is the hope that this contribution may stimulate continued discussion and research in this complex field both from a basic scientific and a clinical perspective.
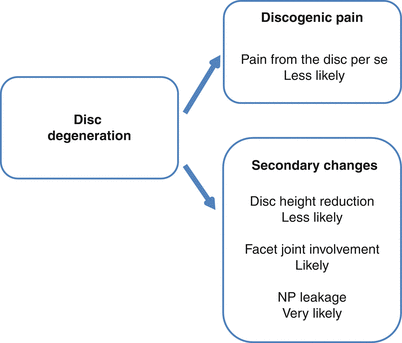
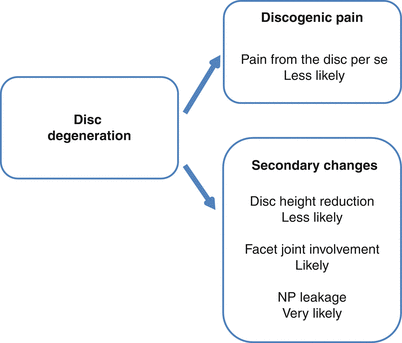
Fig. 16.3
The author’s view of pain mechanisms and their likelihood as the result of disc degeneration
16.10 Summary of Critical Concepts Discussed in the Chapter
The origin of disc pain is still not understood. Pain most likely originates from receptors on nerve fibers on the surface of the posterior aspect of the intervertebral disc.
Pain activation may be due to leakage of disc-derived biologically active molecules which diffuse through annular tears of the annulus fibrosus.
The paucity of innervating nerve fibers would indicate that pain is unlikely to originate from the center of the intervertebral disc.
Disc degeneration and degenerative disc disease are inappropriate terms for changes in the intervertebral disc that may well be merely a stage in the normal aging process.
Efforts to rejuvenate the intervertebral disc by injection of cells or biologic substances may be contraindicative, since it might prolong the degeneration process.
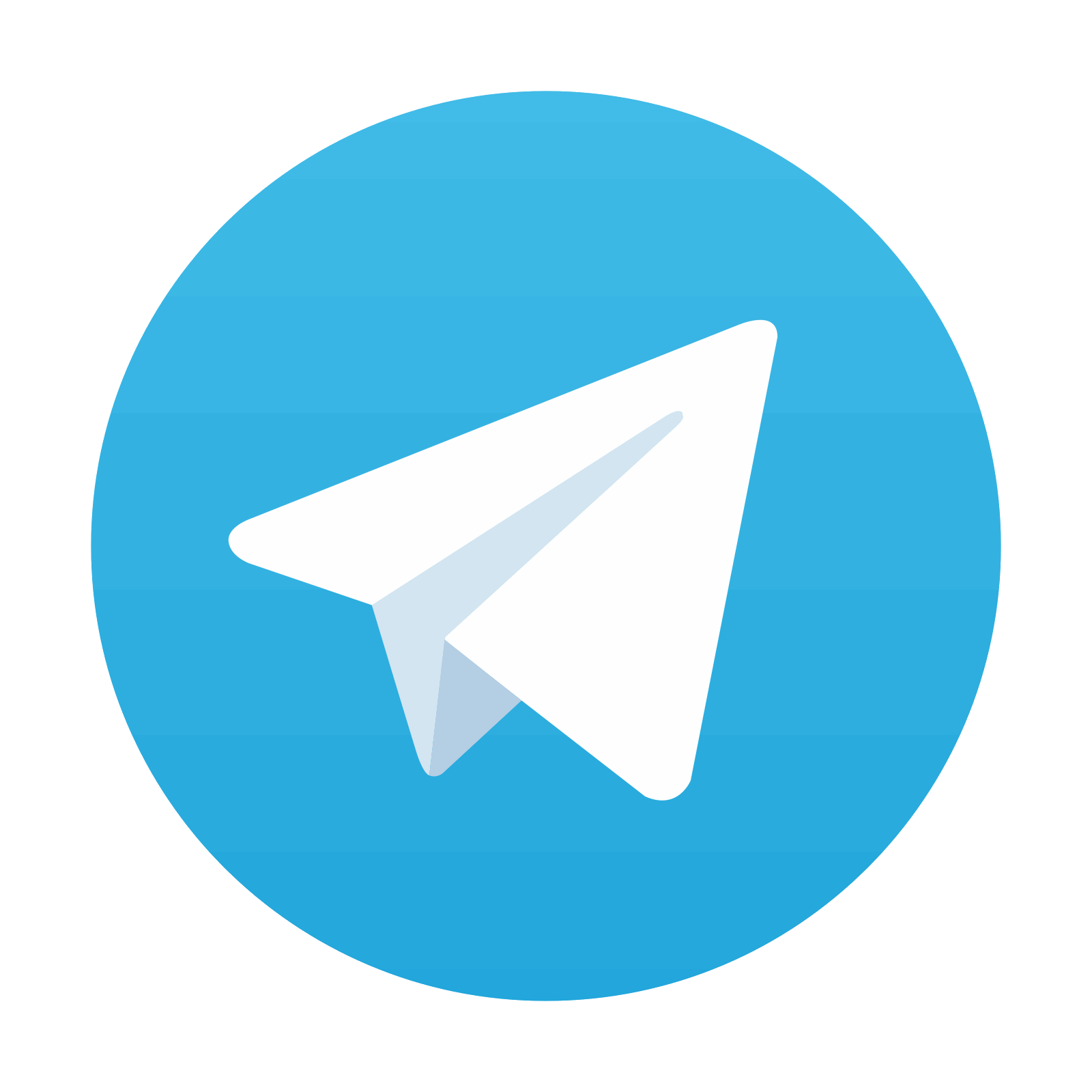
Stay updated, free articles. Join our Telegram channel

Full access? Get Clinical Tree
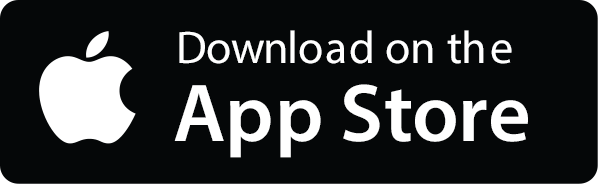
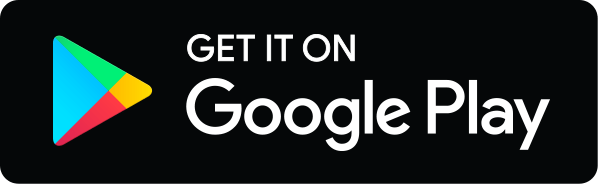