3.4 Anatomy Trains and force transmission Starting at about fourteen days in embryological development, as cells proliferate and specialize, they create an extracellular matrix (ECM) between them (Moore & Persaud 1999). This delicate web-like intercellular gel provides the immediate environment of most cells, mixing varying proportions of fiber, gluey proteoaminoglycans, and water with diverse and circulating metabolites, cytokines, and mineral salts (Williams 1995). It is this ECM that provides most of the “tissue” bulk in many connective tissues, as the cells alter the ECM to form bone, cartilage, ligaments, aponeuroses, and the rest (Snyder 1975). The ECM grows along with the cells themselves and together they form a single organism connected, joined, and held together by the ECM. The ECM is intimately connected to cell membranes and through them to the cytoskeleton via hundreds or thousands of binding integrins on the cell surface (Ingber 1998). Forces from outside the cell are transmitted via these adhesive connections to the inner workings of the cell (Ingber 2006a). Thus, we can now understand that each cell, as well as “tasting” its chemical milieu, is “feeling” and responding to its mechanical environment – leading to the relatively new field of “mechanobiology” (Ingber 2006b). Forces also move in the other direction – from the cell to the ECM – in the case of muscular or (myo) fibroblast contraction that gets conveyed through the membrane to the surrounding ECM (Tomasek et al. 2002). To allow trillions of cells to stand up and walk around in an organismic fashion, the ECM must: • Invest every tissue without exception – muscle, nerve, epithelia, and of course all the connective tissues themselves, from blood to bone. • Be permeable enough to allow all local cells to be in the flow of metabolism yet tough enough to protect those cells from endogenous and exogenous forces. • Vary widely, both across the body from tough bone and resilient cartilage to the lymphatic network of the breast and the aqueous humor of the eye. • Be able to remodel itself over time to meet altered biomechanical conditions in growth, performance, healing, and repair (or pathologically in disease or degeneration). • Transmit forces from one tissue to another with maximum precision, and maximal adaptability to sudden changes in load, while sustaining minimal cellular tissue damage. The ECM acts as the “metamembrane” for the organism, creating an organismic boundary, restraining and directing movement, protecting delicate tissues, and maintaining the recognizable shape most of us maintain from day to day (Juhan 1987; Varela & Frenk 1987). Although the ECM is manifestly one single whole, it is convenient to divide it into three sections: • The tissues of the dorsal cavity – the numerous glia within the nervous system itself, the meninges around the brain and spinal cord, and the perineural extensions out into the rest of the body (Upledger & Vredevoogd 1983). • The tissues of the ventral cavity – the strings, sheets, and sacs that separate the organs and hold them to the body wall, including the mesentery, mediastinum, and peritoneum (Barral & Mercier 1988). • The tissues of the locomotor system – the bones, joints, capsules, ligaments, fasciae, aponeuroses, and all the tissues surrounding and investing the skeletal muscles – endomysium, perimysium, epimysium, and their tendinous extensions (Chaitow 1980). • An “outer” myofascial layer consisting of 600 or so muscles imbedded in the fascia necessary to hold them together, organize their movement, and deliver their force to the bones and other tissues. • An “inner” layer of joint capsules, ligaments, and periostea that surrounds the skeleton and organizes its growth, protects it from dismemberment, and limits movement, thus providing efficient force transmission from one joint to the next (Myers 2009). All of the above divisions are imprecise, due to the integrated nature of the ECM; it is sometimes impossible to tell where one section stops and the other begins, and functionally they are all in league with each other. This last division between the outer and inner “bags” within the musculoskeletal system is particularly porous, since these structures have been shown to work in series more often than in parallel (Van der Wal 2009). After this preamble to holism, the remainder of this chapter will focus on some patterns within this “outer bag” of myofasciae. The traditional view of anatomy that has broadened our knowledge considerably has been gained by a reductionistic parsing of the body, largely with a scalpel. The result is “the muscle” as the predominant label for making named units from the unified soft tissue of this layer. Once a muscle is dissected from its neurovascular fascia, from its overlying areolar layer, and from its neighbors right and left, and the ligaments below, the muscle is analyzed solely in terms of what would happen if the two end points north and south (the so-called proximal and distal attachments) were pulled together in a concentric, isometric, or (with an opposing outside force) eccentric contraction (Williams 1995; Biel 2005; Muscolino 2010). This isolationist muscle analysis separates one function out of the many and raises it to the level of the function. Most analyses of posture and movement proceed from the idea that individual muscles move bones while individual ligaments stabilize them (Kendall & McCreary 1983). Despite the couple of centuries of kinesiology that has taken this model to its limits, one may question whether the nervous system “thinks” in terms of individual muscles, or whether the muscle, a convenient division for the dissector, is even a distinct physiological unit. Neuromotor units within muscles may be a more useful division (Van der Wal 2009), or larger patterns – our focus for the rest of this chapter – may also tell us something useful about human movement and stability functioning. More recent thinking, much of which is described in the previous chapter, has focused on functional wholes and interconnected patterns within this outer layer, rather than looking for the muscle or particular fascial structure as the culprit for systemic failure such as injury (or more pointedly, lack of injury repair). The Anatomy Trains Myofascial Meridians is yet another of these maps, owing much to the work that has come before, from Raymond Dart through Tittel, Mezières, Hoepke, and others, yet at the same time this system has some unique features (Hoepke 1936; Dart 1950).
Introduction – extracellular matrix as metamembrane
Dividing the indivisible
Isolating a muscle
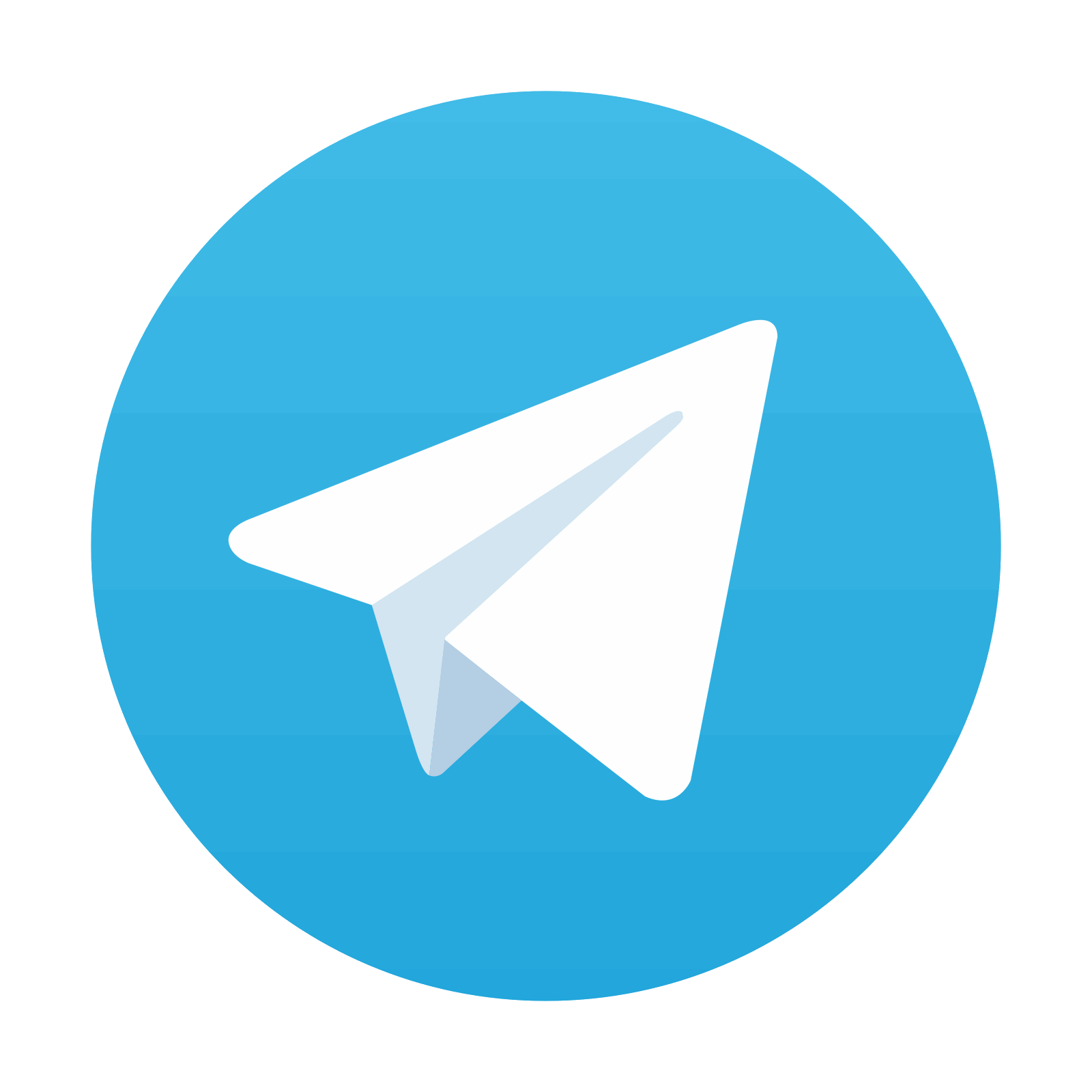
Stay updated, free articles. Join our Telegram channel

Full access? Get Clinical Tree
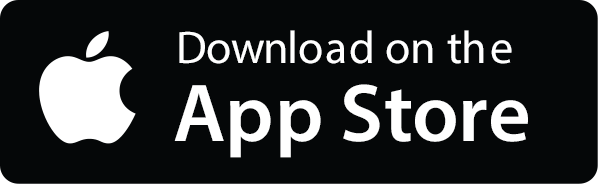
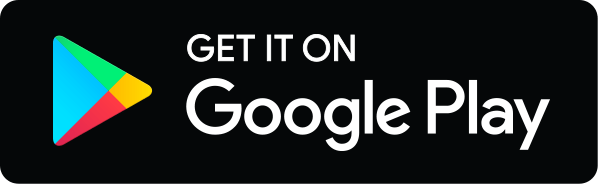