Chapter 143 Advances in Mechanical Compression Devices
Venous thromboembolic disease (VTED) continues to be a major threat to patients undergoing total knee arthroplasty. The incidence of total deep vein thrombosis (DVT) without prophylaxis is between 40% and 85%, and it is between 5% and 22% for proximal DVT. Symptomatic pulmonary embolus (PE) may occur in as many as 10% of patients and may be fatal in up to 1.7%.17 It is estimated that roughly 90% of these symptomatic pulmonary emboli originate from lower extremity DVT.15 Because it is impossible to predict which patients will develop VTED, screening protocols are unreliable, and because treatment of established VTE does not reliably prevent secondary complications, prophylaxis of VTED after total knee arthroplasty (TKA) is mandatory to minimize the sometimes catastrophic sequelae of this disease. Furthermore, with increased scrutiny by regulatory agencies, VTED and its prophylaxis have become measures of quality of care and determinants of reimbursement by payers. From medical, economic, and social perspectives, VTED is one of the great challenges facing the knee replacement surgeon.
Evidence-based guidelines are available to help guide the orthopedic surgeon in choosing an effective VTED prophylaxis regimen. Those published by the American College of Chest Physicians (ACCP)17 acknowledge reduction in the rate of venographically proven DVT as an acceptable surrogate for reducing global VTED and all of its sequelae, including asymptomatic and symptomatic DVT and PE, fatal PE, chronic pulmonary hypertension, recurrent DVT, and postphlebitic syndrome. This is based on an understanding that DVTs will propagate and embolize in a certain percentage of patients, and that reducing the occurrence of DVT should in turn reduce the most feared of outcomes—symptomatic and fatal PE. These guidelines call for aggressive pharmacologic anticoagulation to prevent DVT formation and propagation. Bleeding as a result of this anticoagulation, although rare, may create serious complications at the surgical site, such as wound drainage, hematoma, need for return to the operating room, and infection, which, in turn, may delay rehabilitation or impair the overall outcome of knee replacement.10
In contrast, guidelines published by the American Academy of Orthopaedic Surgeons (AAOS) dispute the causal relationship between DVT and PE, and note that no scientific evidence suggests that any prophylactic regimen, in particular any of those recommended by the ACCP, has actually reduced the incidence of PE after TKA. The goal of these guidelines therefore is to prevent symptomatic PE, and not DVT. The AAOS guidelines reject the underlying assumptions of the ACCP guidelines and therefore aggressive recommendations for chemoprophylaxis and anticoagulation. In contrast, risk stratification is recommended, and for those patients at low risk for VTED, alternative prophylactic regimens are offered that significantly reduce the risk of bleeding complications.20,26,27,32,50
Mechanical Devices
German physician Rudolf Virchow is attributed with describing in 1856 a triad of risk factors associated with DVT—venous stasis, hypercoagulability, and endothelial damage.56 Mechanical compression devices historically have been used to reduce venous stasis in an immobilized patient, one who has difficulty with dorsal and plantar flexion of the foot, or one with other risk factors for DVT. In these patients, venous flow and velocity are impaired, and the resulting pooling of blood within the deep veins of the lower extremity predisposes to DVT formation. Graded compression stockings statically compress the lower extremities, reducing swelling. Pneumatic compression devices utilize a generator to fill air cells in sleeves or cuffs worn on the lower extremities. The repetitive pumping action of the cyclical filling of these air cells compresses the veins, stimulates flow, and reduces stasis. In addition, intravascular fibrinolytic activity may be increased as a result of mechanical compression, potentially counteracting a second arm of Virchow’s triad, hypercoagulability, although the data are mixed.* Venous foot pumps compress the rich venous plantar plexus,55 while other cuffs compress the calf and thigh, depending on their length. Other differentiating features that have been associated with the variability of clinical efficacy are the speed of inflation, whether the air cells are inflated simultaneously or sequentially, and the symmetrical or asymmetrical orientation of the air cells.44,59
The Role of Mechanical Compression After TKA
Graded compression stockings have been studied in orthopedic surgery patients, as well as in other patient cohorts.1,2 Although they may have some beneficial impact, they are not effective as a sole method in significantly reducing VTED after TKA.22,35 Reverse gradients should be avoided,4 and below knee stockings seem to be equivalent to above knee stockings.61
Insall, in the first edition of his classic textbook on knee surgery, noted, “All patients with clinical or scintigraphic evidence of pulmonary embolism also had positive venography, suggesting that the positive venogram … is of clinical significance.” He went on to note, “An early review of the first 50 patients so treated [with intermittent pneumatic compression stockings] indicated a reduction in positive venography from 64 to 11%.”25 Subsequent studies have established that pneumatic compression devices provide effective thromboprophylaxis following TKA, with an incidence of 0% to 48% depending on whether the devices were used alone or with aspirin or low-molecular-weight heparin (LMWH), and whether unilateral or bilateral TKA was performed.* Similarly, older data on venous foot pumps (VFPs) indicated moderate efficacy with a DVT rate of 50% when used alone62 and 27% when used with aspirin. Those patients without DVT wore the pumps 19.2 ± 5.1 hours per day (80% compliance), whereas those who developed DVT wore the pumps only on average 13.4 ± 4.3 hours per day (56% compliance) (P < .001). This established one of the fundamental principles of compression technology—that length of use is directly correlated with efficacy.60 A compression device simply cannot increase venous flow, velocity, pulsatility, and intravascular anticoagulation without actually being worn. This conclusion from VFP data has been extrapolated to all pneumatic compression devices. Other studies of the VFP, however, done during the same time frame demonstrated inferiority when compared with LMWHs.5,47
More recent studies have demonstrated greater efficacy of pneumatic compression devices, perhaps as a result of more aggressive rehabilitation and early mobilization—factors that were not addressed scientifically in these studies. Westrich compared a combination of LMWH and a calf mechanical compression device with aspirin (ASA) and the same device in patients receiving spinal epidural anesthesia for TKA. The incidence of DVT in the ASA group was 17.8%, which was not statistically different from that in the LMWH group.58 Callaghan classified 73% of 427 consecutive TKAs as at low risk for VTED and used ASA, foot pumps, and screening ultrasound for prophylaxis. DVT occurred in only 2.6% of patients, and no PEs or deaths were reported. Comparable results have been reported by other authors using similar protocols.9,14,52 Lotke studied 3473 consecutive TKAs treated with aspirin, foot pumps, early mobilization, and regional anesthesia and demonstrated a maximum 0.14% fatal PE rate at 6 weeks.37 A similar protocol of ASA, pneumatic calf compression, and regional anesthesia achieved a 90-day mortality rate of 0.14%, with a DVT rate of 9.3% and a symptomatic PE rate of 0.5%.34 A meta-analysis of articles published between 1980 and 1997 requiring routine venography and lung scanning when appropriate reviewed 136 articles and determined that of all methods evaluated, including ASA, warfarin, LMWH, and pneumatic compression, pneumatic compression provided the lowest DVT rate at 17% and asymptomatic PE rate at 6.3%, as well as symptomatic PE at 0%.57
Not all pneumatic compression devices achieve the same results. In fact, many have not been clinically evaluated for efficacy in reducing VTED, no industry standards are available for these devices, and demonstration of efficacy is not a requirement for FDA approval and subsequent marketing.17 Rapid calf compression has been associated with the largest increase in venous volume and velocity below the saphenous vein in a study comparing three calf-to-thigh pumps, two foot pumps, one foot-to-calf pump, and one calf pump.59 In addition, Lachiewicz compared a rapid inflation asymmetrical compression device with a sequential circumferential compression device in 472 TKAs. The rapid inflation asymmetrical compression device conferred a significantly lower DVT rate at 6.9% versus 15% (P = .007).33
Based on these and other studies, it can reasonably be concluded that pneumatic compression alone, but mostly as part of a multimodal approach to VTED prevention after TKA, is moderately to significantly effective, with no risk for increased bleeding. However, results have been highly variable and somewhat inconsistent. The generally superior ability of chemoprophylaxis to prevent DVT and the challenges of compliance and portability have relegated the use of pneumatic compression to adjunctive treatment for more aggressive anticoagulation or for those at high risk for bleeding. However, with heightened awareness of and sensitivity to bleeding risks with aggressive chemoprophylaxis, and data demonstrating that ACCP guidelines may increase complications related to bleeding,7,48,49,51 there has been a resurgence of enthusiasm for the use of safer regimens such as pneumatic compression. In addition, new pneumatic compression technology promises to improve efficacy dramatically and may ultimately create an entire paradigm shift in the thromboprophylaxis arena.
Emerging Technology
A novel intermittent pneumatic compression device, the ActiveCare with Synchronized Flow Technology (SFT) (Medical Compression Systems, Or Akiva, Israel), delivering continuous enhanced circulatory therapy (CECT), has been developed with the specific aim of overcoming the key challenges of compliance—ease of use, patient comfort, and portability—while still delivering an impulse that generates a high-peak venous velocity. It is based on a more contemporary understanding of Virchow’s risk factor of global stasis of the lower extremities, as a more local stasis phenomenon occurring at the level of the venous valve sinuses. In the absence of pulsatile flow through these sinuses, local hypoxemia leads to endothelial injury and death, which, in turn, initiates the clotting cascade and results in deep vein thrombosis. Therefore, delivering a high-peak venous velocity is critical to avoiding this local stasis and DVT pathway.*
Clinically, this has been confirmed by the study of Lachiewicz, quoted earlier, which compared two different pneumatic compression devices. The device with greater pulsatility significantly reduced the incidence of DVT (6.9% vs. 15%; P = .007),33 despite the fact that both devices had previously been shown to augment total venous return equivalently.59 Achieving a higher peak venous velocity and pulsatility is more important than total venous return as a means of preventing DVT.
Delivering this high-peak venous velocity, however, has been associated with a jarring impact sensation for the patient, adding to the already formidable barriers to compliance, including nonportability, and difficulty in application and use of the sleeves. Recent data suggest that the peak venous velocity generated by a calf muscle contraction can be significantly enhanced by synchronizing it with the phase of the respiratory cycle that maximally reduces resistance to venous return.42 Because pneumatic compression devices mimic the normal calf contraction, coupling the compression to the expiration phase of diaphragmatic respiration should increase peak venous velocity and should minimize the force of the impulse necessary to generate this effect, in turn improving comfort and effectiveness simultaneously. This has been confirmed in studies performed at the laboratories of Medical Compression Systems.3
With this information as a foundation, the ActiveCare with Synchronized Flow Technology was designed with a sensor enabling inflation of the air cells contained within the compression device to be synchronized with the expiration phase of the respiratory cycle. External calf compression occurs therefore when resistance to venous return is minimized. Maximum peak venous velocity is achieved with gentler and more comfortable filling of the sleeve, eliminating the usual explosive and high-pressure compression and its associated patient discomfort. In addition, this new device is completely portable, weighing only about 1.5 pounds, with a generator operated by a standard electrical current or by a small, contained rechargeable battery lasting up to 6 hours (Fig. 143-1). The sleeves are designed to be form-fitting and easy to apply, and compliance can be monitored directly from the data screen on the front of the device (Fig. 143-2).
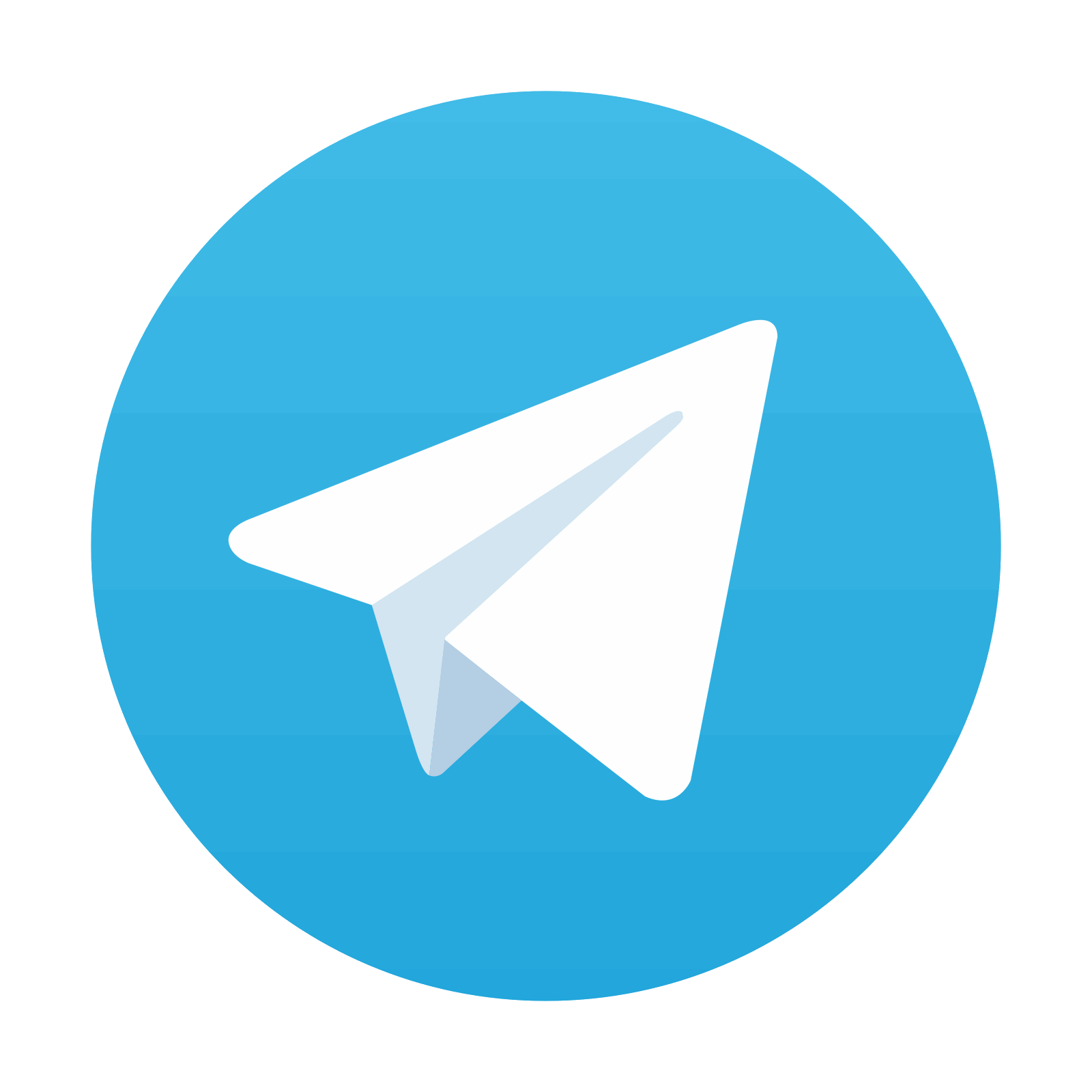
Stay updated, free articles. Join our Telegram channel

Full access? Get Clinical Tree
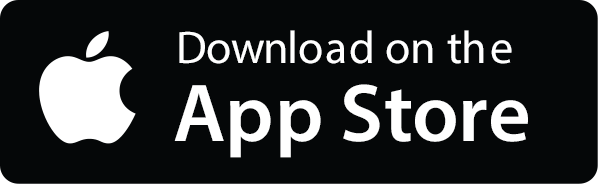
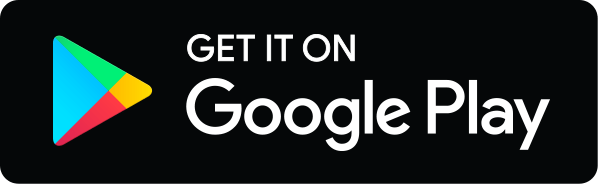