A thorough understanding of the spinal nerve and its implications in diagnosis and case management necessitates a review of its development in the embryo, as discussed in Chapter 4. In this light, the basic architecture of the nerve and its components (i.e., the tree analogy) becomes clear. This chapter offers a more functional approach, with emphasis on information processing in the peripheral nervous system and its relationship to certain activities in the central nervous system. The information presented here is by no means comprehensive, but it serves as a springboard for further study (see Suggested Reading).
Neurons convey information in the form of impulses or action potentials, which typically travel in a specific direction. The peripheral nervous system (PNS), which consists of the spinal nerve roots, spinal nerves, and peripheral nerves, is responsible for bringing information about the external and internal environments to the central nervous system (CNS), which consists of the brain and spinal cord. This type of input information is referred to as sensory or afferent. The PNS also transmits responses to sensory stimuli, bringing motor or efferent output information from the CNS to target tissues in the periphery.
BASIC STRUCTURE OF THE NEURON
The neuron is made up of three basic parts. The soma, or cell body, is the point at which the cell nucleus resides along with other cellular organelles (Fig. 5-1). Extending from the soma are two types of processes. The dendrite, which receives signaling information from the local environment, contains, or is associated with, receptors that are designed to sense specific stimuli or react to specific chemical transmitters. Neurons may have hundreds or even thousands of dendrites, depending on the size and complexity of their receptive fields. The second type of cellular process is the axon, along which a signal travels as an action potential. Action potentials are typically generated in an axon via the summation of signals received from the dendrites, which can be either stimulatory or inhibitory. When the action potential reaches the end of the axon, it causes the release of one or more neurotransmitters, which signal either other neurons or specific target tissues. Axons can branch extensively, allowing a single neuron to communicate with and send signals to many other neurons. This phenomenon is known as divergence and is significant when the spread of information is desirable for the organism (Fig. 5-2). In this case, the signal is said to be amplified. Conversely, when signals from multiple neuronal sources terminate on a single neuron, convergence is said to exist (Fig. 5-3). When convergence exists, some signals may be excitatory; others may be inhibitory. As with all neurons, the summation of signals may (or may not) reach the threshold for the stimulated neuron to generate an action potential.
![]() |
Fig. 5-1 Schematic view of a typical neuron, indicating synaptic inputs to its dendrites (although other sites are possible) and information flow down its axon, reaching synaptic endings on other neurons. Information flow is unidirectional because of molecular specializations of various parts of neurons. The segments covering the axon represent the myelin sheath that coats many axons, and the gap in the axon represents a missing extent that might be as long as 1 meter in length. (From Nolte J: The human brain: an introduction to its functional anatomy, ed 5, St Louis, 2002, Mosby.) |
![]() |
Fig. 5-2 Divergence in neuronal pathways; A, divergence within a pathway to cause amplification of the signal; B, divergence into multiple tracts to transmit the signal to separate areas. (From Guyton AC: Hall J: Textbook of medical physiology, ed 10, Philadelphia, 2001, WB Saunders.) |
![]() |
Fig. 5-3 Convergence of multiple input fibers onto a single neuron; A, multiple input fibers from a single source; B, input fibers from multiple sources. (From Guyton AC: Hall J: Textbook of medical physiology, ed 10, Philadelphia, 2001, WB Saunders.) |
FACTORS INFLUENCING CONDUCTION VELOCITY
Neurons of the PNS are associated with Schwann cells (myelin). These cells are responsible for supporting and insulating axons, allowing for more efficient propagation of action potentials. Neuronsare said to be myelinated or unmyelinated, depending on the number of times a Schwann cell wraps around a given axon (often referred to as a fiber) (Fig. 5-4).
![]() |
Fig. 5-4 Function of the Schwann cell to insulate nerve fibers; A, the wrapping of a Schwann cell membrane around a large axon to form the myelin sheath of the myelinated nerve fiber. B, Partial wrapping of the membrane and cytoplasm of a Schwann cell around multiple unmyelinated nerve fibers (shown in cross section). A,(Modified from Leeson TS, Leeson R: Histology, Philadelphia: 1979, WB Saunders.); B,(From Guyton AC: Hall J: Textbook of medical physiology, ed 10, Philadelphia, 2001, WB Saunders.) |
Action potentials are propagated along axons with varying speeds. The speed of an action potential depends primarily on two factors: the diameter of the axon and the degree of myelination. Larger diameter and heavily myelinated axons (A fibers) convey impulses considerably faster than smaller diameter unmyelinated axons (C fibers). The difference in propagation speeds becomes important when discussing the transmission of pain (see later discussion).
A cross section of the spinal cord reveals two basic components: gray matter and white matter (Fig. 5-5). The gray matter is a butterfly-shaped column of nerve cell bodies, axons, and dendrites. Because of the metabolic needs of the neuronal cell bodies, a robust blood supply is contained in the gray matter, providing its characteristic appearance. The butterfly is subdivided into a pair of dorsal (or posterior) horns, or columns (when seen three dimensionally), and a pair of ventral (or anterior) horns, or columns. The dorsal horns are responsible for processing sensory information, which enters the cord where the dorsal root of the spinal nerve joins it. Axons from the dorsal root will penetrate the dorsal horn to varying depths before terminating and synapsing with other neurons, or they will leave the gray matter to form a tract.
![]() |
Fig. 5-5 General cross sectional anatomy of the spinal cord, represented in this case by the eighth cervical segment. PF, Posterior funiculus; AF, anterior funiculus; LF, lateral funiculus; PH, posterior horn; AH, anterior horn; IG, intermediate gray. (From Nolte J: The human brain: an introduction to its functional anatomy, ed 5, St Louis, 2002, Mosby.) |
The white matter, in contrast, consists primarily of myelinated axons forming ascending and descending tracts that convey information toother areas of the CNS. Specialized cells called oligodendrocytes are responsible for myelination in the CNS. The white matter is subdivided into columns or funiculi, whose names are derived from their relationship with the gray matter: anterior, posterior, and lateral. The fatty myelin sheaths are responsible for the coloration of the white matter.
SENSORY NEURONS
The sensory neurons contained in the spinal nerves are of the pseudounipolar variety (Fig. 5-6). The cell bodies of these sensory neurons are located in the dorsal root ganglion (DRG).
![]() |
Fig. 5-6 Scheme showing the constitution of a typical spinal nerve. In the upper part of the diagram, the spinal cord nerve roots show the somatic components. In the lower part of the diagram, the spinal roots show the visceral components, somatic efferent and preganglionic sympathetic fibers, somatic afferent and visceral afferent fibers, and postganglionic sympathetic fibers. (From Williams PL, editor: Gray’s anatomy, ed 38, London, 1995, Churchill-Livingstone.) Churchill-Livingstone |
A ganglion is a collection of functionally related nerve cell bodies outside the central nervous system. Nerves whose cell bodies reside in ganglia can be sensory (somatic or visceral), or motor (visceral). All ganglion cells are derived from the embryonic neural crest.
These nerves convey a wide range of sensations. Each specific sensation is referred to as a modality. Some examples are light touch, proprioceptive sensations (giving a sense of position to the body), and pain. These and other modalities are discussed later in this chapter. Specific receptors exist for each modality (Fig. 5-7). Sensory receptors are divided into three basic types: exteroceptors, which carry information that originates outside or on the surface of the body, interoceptors, which convey information originating from inside the body, and proprioceptors, which provide information on body position and movement.
![]() |
Fig. 5-7 Some of the sensory endings found in glabrous (smooth and bare) skin. M, Meissner corpuscle; Me, Merkel cell; PC, Pacinian corpuscle; R, Ruffini ending. (From Nolte J: The human brain: an introduction to its functional anatomy, ed 5, St Louis, 2002, Mosby.) |
Subclasses of the exteroceptors include:
• Mechanoreceptive (touch, hearing)
• Thermoreceptive (temperature)
• Nociceptive (pain)
• Chemoreceptive (smell, taste)
• Electromagnetic (vision)
The proprioceptors, which respond to mechanical stimuli, such as the state of contraction of a muscle (muscle spindles) or the tension on a tendon or joint (Golgi tendon organs), are also classified as mechanoreceptors.
Sensory receptors generate an action potential by changing the neuronal cell membrane in some way. This action can take the form of physical deformation (mechanoreceptors) or interaction of chemicals with the membrane. Both types of stimuli cause the opening of ion channels in the cell membrane. In addition, changes in temperature (thermoreceptors) or electromagnetic radiation (visual) can alter membrane permeability. The receptors of spinal nerves can also be classified as encapsulated or free nerve endings. Encapsulated receptors include the touch receptors and proprioceptors. Free nerve endings convey pain and temperature sensations.
Most sensory receptors can be classified as slowly adapting (SA) or rapidly adapting (RA). In either case, the receptors are responding to some type of change in the local environment. Adaptation of receptors occurs when a constant stimulus is applied. As the stimulus is applied over time, the rate of impulse response to the stimulus will gradually decrease to a very low level (i.e., The individual “gets used to it.”). Therefore SA receptors will adapt more slowly to a stimulus, and it will be perceived for a longer period. SA receptors are also referred to as tonic receptors. In contrast, RA receptors will respond more efficiently to changes in stimulus strength, such as occurs with vibration, and are referred to as phasic, rate, or movement receptors. Nociceptors, or receptors that perceive painful stimuli, are referred to as nonadapting, implying that there is no getting used to pain. (See Chapter 9 for an extensive discussion of nociceptors.)
The neurons contained in the DRGs of spinal nerves generally convey either somatic information from the body surface, walls of body cavities, and extremities or visceral information from the internal organs and contents of body cavities. The special senses (smell, vision, hearing, and taste) are conveyed by the cranial nerves. An important point to remember is that each neuron has a specific receptor or set of receptors on its peripheral end that are consistent with the type of information that the neuron conveys (i.e., neurons are modality specific). Also worthy of note is that some DRG neurons are quite long. For example, a single neuron carrying pain information from the foot might be 3 to 4 feet or longer, depending on the height of the individual.
The peripheral distribution of the sensory neuron conforms to its distribution in the embryo and fetus (see Chapter 4). In addition, these neurons can branch or collateralize quite extensively on their peripheral ends. This branching can result in an extensive sensory field for a single neuron. Thus the sensory component (along with the motor component) of the spinal nerve is distributed to the dermatome (for touch, temperature, and pain), the myotome, and the sclerotome (for proprioception and pain). The dermatomes of the spinal nerves follow a specific pattern, which is routinely exploited by clinicians (Fig. 5-8). For example, if a spinal nerve is damaged, the patient may experience numbness or tingling along the nerve’s dermatome. By contrast, if a spinal nerve is impinged by another structure such as an intervertebral disk, the patient may experience radiating pain along the entire length of the dermatome, in spite of the site of impingement being quite small and proximal.
![]() |
Fig. 5-8 Dermatomes. (Modified from Grinker RR, Sahs AL: Neurology, Springfield, Ill, 1996, Charles C Thomas; cited in Guyton AC: Hall J: Textbook of medical physiology, ed 10, Philadelphia, 2001, WB Saunders.) |
The central process of the DRG neuron projects through the dorsal root of the spinal nerve into the dorsal horn of the spinal cord. As these nerves collateralize (branch) peripherally, they can also collateralize centrally, resulting in a specific field of distribution for the incoming signal. This property means that a single DRG neuron can communicate with many neurons in the spinal cord, producing a variety of effects. From the dorsal horn of the spinal cord, modality-specific neurons relay to or directly enter the nerve tracts that convey specific types of information upward to the brainstem and cerebral cortex (discussed later). This specificity in conveying a specific type of information from the periphery to a specific tract and subsequently to a specific area of the CNS is known as a labeled line.
Somatic motor neurons come in two varieties: alpha and gamma (Aα and Aγ) motor neurons. Alpha motor neurons are responsible for innervating and activating skeletal muscle fibers through the myoneural junction. These neurons fall into the category of large diameter, fast-conducting nerve fibers. Acetylcholine is secreted into the myoneural junction, which, in turn, stimulates the muscle to contract. Gamma motor neurons are responsible for innervating muscle spindles, which establish a set point for muscle tone (Fig. 5-9). These neurons are somewhat smaller in diameter than alpha motor neurons and thus conduct impulses at a somewhat slower velocity. Motor neurons, similar to sensory neurons, can have exceedingly long axons, projecting from the spinal cord all the way to the target muscle.
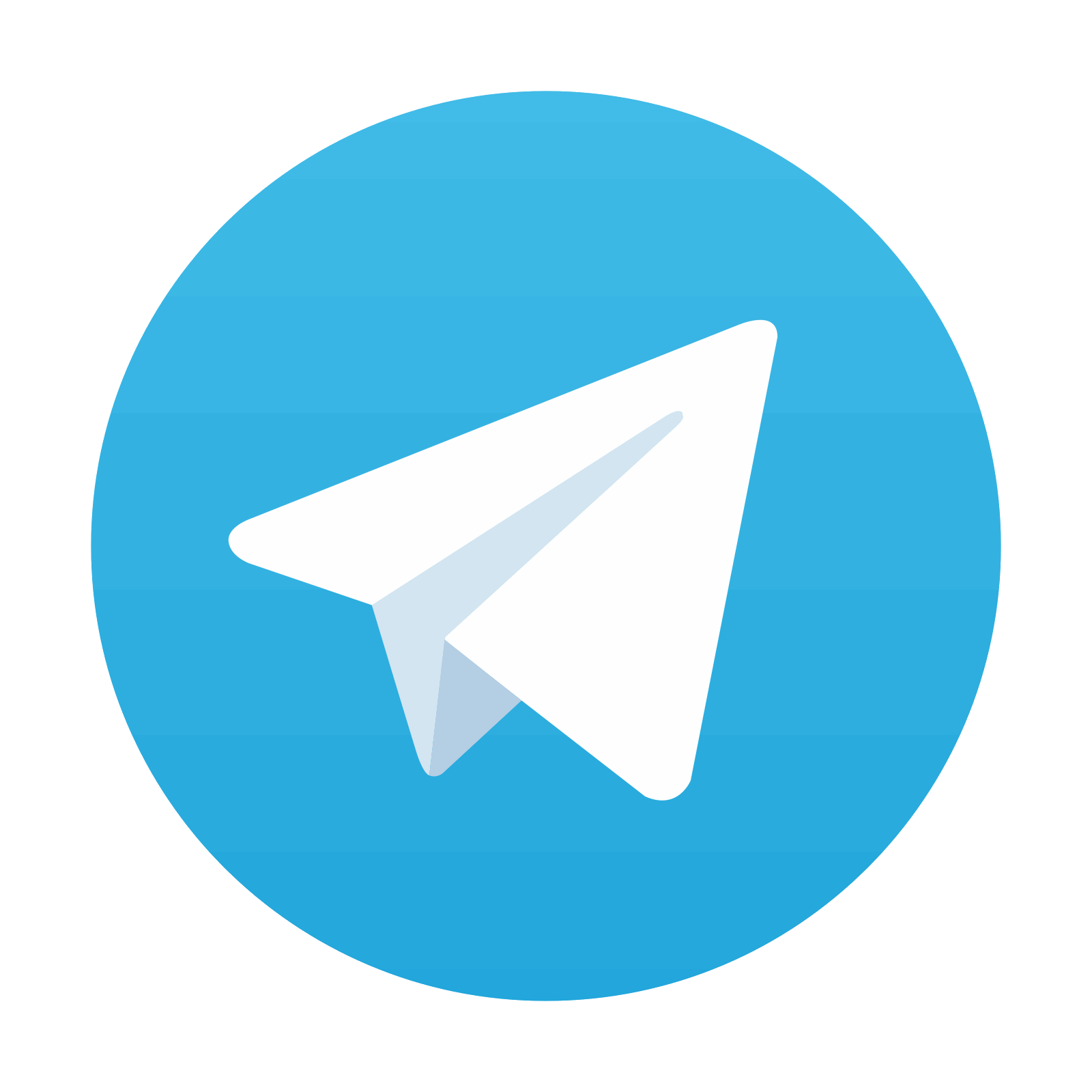
Stay updated, free articles. Join our Telegram channel

Full access? Get Clinical Tree
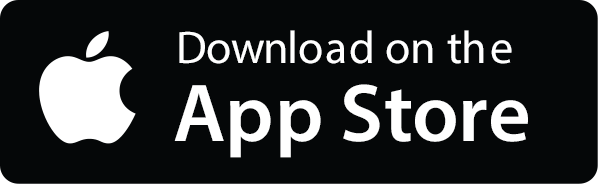
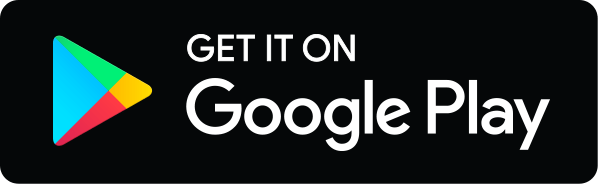
