Summary
The right main thoracic (MT) curve pattern is the prototypic scoliotic deformity seen in adolescent idiopathic scoliosis (AIS). This chapter addresses the characteristics of the right MT curve pattern in AIS and discusses the criteria used to decide when and how to address this spinal deformity. Options for selective versus nonselective fusion are discussed, as are the surgical approaches currently used for the correction of MT curves. Specifically, the particular indications and contraindications for each approach are evaluated, and recommendations are made for surgical technique and for the selection of fusion levels. In most cases, a reliable outcome is achievable with careful preoperative assessment. Posterior instrumentation has over the past decade become the standard for the surgical treatment of nearly all thoracic curves, and pedicle screws seem to be at the root of the change from prior practice, in which anterior instrumentation was more common. Although careful prospective assessment of the thoracoscopic approach suggests that it is a viable option for some patients, it appears to be impractical for the vast majority of surgeons. With similar radiographic and patient-reported outcome measures, the additional operative time and technical challenges of the thoracoscopic approach do not seem to be worth the effort for most patients. As spine surgeons strive for the increasing correction of scoliosis, it is necessary to always remember the primary goal of surgery of safely achieving a balanced spinal fusion that will limit the risk of curve progression and late sequelae.
Key words
adolescent idiopathic scoliosis – right main thoracic curve – three-dimensional analysis – deformity–flexibility quotient – selective versus nonselective fusion – open anterior spinal fusion – thoracoscopic anterior approach – posterior spinal fusion16 Surgical Treatment of the Right Thoracic Curve Pattern
16.1 Introduction
The right main thoracic (MT) curve pattern is the prototypic scoliotic deformity seen in adolescent idiopathic scoliosis (AIS). By definition, an MT curve in AIS has an apex between T2 and T11 and has the largest coronal deviation as measured by the Cobb angle method. Measuring apical deviation, relative apical lordosis, and transverse plane rotation provides further means of describing the major curve and captures some of the three-dimensional (3D) nature of this deformity.
Characteristics of the minor curves proximal and distal to the MT curve are as important as the features of the 3D thoracic deformity. Many of the decisions to be made in the treatment of MT curves depend on these minor-curve characteristics. Understanding how minor curves will “respond” to surgical correction of the MT curve is critical in the treatment of scoliosis.
This chapter addresses the characteristics of the right MT curve pattern in AIS and discusses the criteria used to decide when and how to address this spinal deformity. Options for selective versus nonselective fusion are discussed, as are the surgical approaches currently used for the correction of MT curves. Specifically, the particular indications and contraindications for each approach are evaluated, and recommendations are made for surgical technique and for the selection of fusion levels.
16.2 Deformity Classification
Both the King–Moe 1 and Lenke 2 classification systems for AIS have been useful in characterizing the classic right MT curve pattern. Three of the five curve types in the King–Moe classification describe a major thoracic spinal deformity: King–Moe II, King–Moe III, and King–Moe IV. These curve types roughly correlate with Lenke 1B/1C, Lenke 1A/1B, and Lenke 1A curve types, respectively. Although none is perfect, all three provide insight into which region of the spine needs to be surgically treated for this common pattern of scoliosis.
The major feature distinguishing right MT curves from one another is the nature of the lumbar deformity. For example, the lumbar modifier in the Lenke classification system describes the varying degree of apical deviation of the lumbar curve. The “A” modifier is used when the center sacral vertical line (CSVL) falls medial to the pedicle of the lumbar apical vertebra and describes both lumbar curves with no apical deviation (King–Moe IV) and lumbar curves with slight apical deviation (King–Moe III). The “B” lumbar modifier is applied when the minor lumbar curve has moderate apical deviation as defined by the CSVL falling between the medial pedicle wall and the lateral edge of the apical vertebral body. The “C” modifier represents a more substantial lumbar curve where the entire lumbar apical vertebral body is deviated lateral to the CSVL. These distinctions are important when selecting the lowest instrumented vertebra (LIV) for each curve type.
The definitions of Lenke type 1 or MT curve patterns are largely reproducible. The original study that described the Lenke classification system 2 reported high interobserver (0.92) and intraobserver (0.83) kappa values among the five investigators who developed the system. An independent analysis 3 in 2002 reported lower kappa values with the Lenke system than with the King–Moe classification system (0.62 and 0.73, respectively); however, they were still noted to be significantly higher than those historically reported for the King–Moe system. 4 , 5 Despite the relatively high level of agreement in the classification of MT curves, variability exists in both the selection of an operative approach and fusion levels for treating these deformities, confirming the current lack of standardized treatment paradigms in scoliosis surgery, and highlighting that the classification systems to date do not aid in fusion level selection or surgical approach but rather allow for a broader approach of when the treating surgeon should consider a selective versus a nonselective fusion.
The Lenke system works because it has relatively simple rules that define the curve patterns in scoliosis. The system was developed to aid in choosing which regions of the spine need to be surgically treated and therefore does not necessarily distinguish among truly different curve patterns. This is most obvious for Lenke type 1A curves. In an analysis of these curves done from the Harms Study Group (HSG) database, two distinct curve patterns emerged. 6 The Lenke type 1A curve with L4 tilted to the right, denoted as type 1AR, is a long thoracic curve which resembles the King–Moe IV pattern (Fig. 16‑1). The fusion level for this curve is more distal than when L4 is tilted to the left (type 1AL; Fig. 16‑2). The type 1AL curve resembles the Lenke type 1B/C pattern, especially from the standpoint of choosing the LIV (Fig. 16‑3). A recent 3D analysis of these two curve patterns demonstrated clear differences in all three planes indicating that the differing features of the nonstructural lumbar curves may help define the variance in fusion level selection and risk for adding-on. 7



16.2.1 Three-Dimensional Analysis of the MT Curve
Another area of controversy with regard to the classification of right thoracic curves relates to the sagittal and axial planes. The Lenke classification has added a sagittal modifier, bringing attention to this important aspect of the deformity in scoliosis. However, the T5–T12 sagittal measure used to grade the sagittal alignment as hyperkyphotic, normal, or hypokyphotic does not characterize the sagittal deformity at the thoracic apex, which is nearly always hypokyphotic or even lordotic compared to children without scoliosis. This apical “lordosis” has been suggested for years by numerous authors 8 , 9 , 10 , 11 , 12 as a common feature of thoracic scoliosis and can be difficult to appreciate on standard lateral radiographs because of the presence of vertebral rotation. Stagnara and Queneau 11 suggested a rotated anteroposterior (AP) and lateral view to identify the “true” nature of the deformity in both planes. However, this rotated view does not capture the global deformity or the 3D relationship of the thoracic curve to the other regions of the spine.
Three-dimensional analysis and simultaneous upright biplanar imaging of the scoliotic spine have gained popularity to better understand the deformity present. An analysis of the apical segments of 66 patients, done with custom Matlab software and 3D reconstructions, demonstrated consistent reduction in kyphosis of almost 10 degrees compared to standard lateral radiographs. 13 It is clear that an understanding of all three planes of deformity in each patient with AIS is required to optimize surgical treatment.
Additionally, an axial rotational deformity that in many cases represents the primary deformity in the eyes of the patient is associated with the apical lordosis (whether relative or absolute). Clinical deformity of trunk shape as determined by coronal decompensation, truncal shift, difference in shoulder height, a thoracic rib prominence, and lumbar prominence varies among patients with right thoracic curves. These clinical findings, as well as radiographic measures, must be incorporated into the surgical plan for each patient. Those with a lumbar curve to the left, of varying magnitude (Lenke types 1AL, 1B, 1C), whose head may be relatively balanced over their pelvis (Fig. 16‑4), and those with an isolated thoracic curve (Lenke type 1AR) who tend to have a greater right-sided truncal shift and a larger thoracic rib prominence (Fig. 16‑5), require different treatment strategies.


16.3 Decisions Relating to Surgical Treatment
A number of decisions must be made before and during the surgical correction of right MT curves in scoliosis. The questions to be addressed in each case are listed below and will frame the discussion that follows.
Is an instrumented fusion indicated?
Which, if any, of the minor curves should be included in the fusion?
To what extent should the thoracic curve be corrected for ideal global balance?
What vertebral levels should be included in the fusion?
What is the best approach?
Is an anterior release indicated?
16.3.1 Is a Surgically Instrumented Fusion Indicated?
Whether a surgical instrument fusion is indicated in a case of AIS remains one of the more controversial questions in its treatment. This is particularly true for curves in the 40- to 50-degree range. Treatment assumes that both the short- and long-term outcomes will be better with a fused spine than with the untreated natural history of AIS. It is the lack of knowledge in both of these situations over the long term that leaves the need for surgical treatment of these patients open for debate.
Traditionally, the magnitude of the spinal curvature in AIS as reflected by the Cobb angle has been the primary determinant of risk for curve progression in adulthood. Weinstein and colleagues 14 , 15 suggested the tidemark of 50 degrees as the criterion for surgical decision-making in thoracic curves. With curvature greater than 50 degrees there is a higher risk for progression. The risk with curves between 40 and 50 degrees is less clear, and with curves of less than 40 degrees the risk of adult progression appears low. There are, however, various other ways to define the qualities of a spinal curve besides the Cobb angle, and it seems almost certain that there are more precise ways to predict late progression. 16 , 17 , 18 Until these are identified, we use limited data and prudent judgment to make this critical decision.
Another unproven variable used in the decision to operate in cases of AIS relates to the length of the curve. The more vertebrae within the Cobb angle, the more severe the clinical deformity. Coronal balance is also an important variable, and when a lumbar curve exists that matches or balances the thoracic deformity, as in many Lenke type 1C curves, surgical treatment may be delayed. In addition, for patients who have not completed growth, the remaining growth potential may weigh into the decision about whether or not to operate, with surgery often being indicated for younger patients with curves greater than 40 degrees as compared with the traditional 50-degree indication for those who have completed growth.
16.3.2 The Inclusion of Minor Curves in the Fusion
Selective thoracic fusion for the MT curve pattern of AIS was suggested by Moe more than 50 years ago, 19 and for the most part this concept remains as valid today as it was then. The difficulty has been to find a reliable way to determine if the minor lumbar curve needs to be surgically treated. The Lenke classification system was designed with the goal of answering this specific question and suggests that minor nonstructural curves (which side-bend to < 25degrees) can be spared from fusion if there is no appreciable junctional kyphosis. Several recent studies have supported the guidelines provided by the Lenke classification as appropriate for the majority of cases of AIS, supporting the use of selective thoracic fusion in King type II and Lenke type 1C curves (Fig. 16‑6). In 2003, Lenke and colleagues 20 reported that selective thoracic fusion of the major curve could be successfully accomplished even when the minor lumbar curve deviated completely from the midline (lumbar modifier C), thus optimizing the postoperative number of mobile lumbar segments. In 2004, Edwards et al 21 also described satisfactory results after selective thoracic fusion of properly selected curves with a “C” lumbar modifier. These authors concluded that mild coronal imbalance was well tolerated and did not necessitate the distal extension of the fusion in such cases. The problem remains in that what is considered a satisfactory result of surgery in such cases varies in the viewpoints of surgeons and patients.

Clements and coworkers 22 reviewed the surgical procedures used for the cases in the HSG database before and after the Lenke classification was developed. The Lenke classification was applied retrospectively to cases treated before 2001 and was found to correctly predict which curves were actually fused in 82% of the cases. After the HSG adopted the use of the Lenke classification, the system predicted the curves actually fused in a significantly greater percentage of cases (88%; p = 0.001). Thus, the uniformity of treatment improved with the use of the Lenke classification system, but the system did not completely explain the practice of this group of experienced scoliosis surgeons. “Rule-breakers” were defined as patients whose treatment did not follow the recommendations of the Lenke classification system. From 6 to 29% of the time (depending on the curve pattern), other aspects of a patient’s clinical and radiographic deformity suggested deviation from the recommended treatment paradigm. From this “rule-breaking,” it can be concluded that the classification system does not identify the structural lumbar curve in 100% of cases of AIS.
Breaking the “rules” of the Lenke classification system for choosing the minor curves to treat or not to treat in patients with AIS is appropriate; the trick is to know when to break the rules and to understand why they are broken. Being wrong 10% of the time is not good enough when addressing the motion of a child’s spine over its lifetime. Puno et al 23 evaluated the usefulness of the Lenke classification system in providing treatment recommendations for idiopathic scoliosis. They compared the postoperative Cobb angle correction and truncal shift in patients who were either treated or not treated according to the Lenke classification system and reported better radiographic results when the Lenke classification system was used to select fusion levels with avoidance of the unnecessary fusion of nonstructural lumbar or thoracic curves and avoidance of the undercorrection of structural secondary curves. However, the distinction between different types of curves in the Lenke classification can be difficult (i.e., types 1C, 3C, and 6C curves with large thoracic and large thoracolumbar/lumbar [TL/L] spinal deformities) and can influence treatment decisions. Differentiation of these types of curves according to the Lenke classification system is based on arbitrary values for the magnitude and flexibility of the TL spine and does not address the 3D and clinical aspects of the deformity.
Not surprisingly, the debate about when to include the lumbar curve in the fusion levels in cases of Lenke types 1C and 3C curves is most controversial (Fig. 16‑7). In 1992, Lenke and colleagues 24 described strict criteria for preventing lumbar decompensation in the performance of selective thoracic fusion. The rules were based on ratios of the magnitudes of the thoracic and lumbar Cobb angles, apical vertebral deviation from the midline, and apical vertebral rotation on the standing coronal radiograph. Despite these guidelines, a review by Newton et al 25 of the frequency with which surgeons in the HSG included the lumbar curve in the fusion in cases of Lenke types 1B and 1C curves found a substantial variation in the frequency of nonselective fusion into the lumbar curve (6–33%). Excluding cases of junctional kyphosis, the lumbar curve was included in the fusion for Lenke type 1B curves in only a few cases (2%). However, the incidence of inclusion of the lumbar spine in fusions of Lenke 1C curves varied from a low of 6% for one surgeon to a high of 67% for another.

The disparity of this magnitude suggests a void in the knowledge base about the current definition of the structural lumbar curve. Factors associated with nonselective fusion in Newton and colleagues’ review included a lumbar curve of larger preoperative magnitude (42 ± 10 degrees vs. 37 ± 7 degrees, p < 0.01), greater lumbar apical deviation (3.1 ± 1.4 cm vs. 2.2 ± 0.8 cm, p < 0.01), and a smaller ratio of thoracic-to-lumbar curve magnitude (1.31 ± 0.29 vs. 1.44 ± 0.30, p = 0.01). In addition, selective fusions were also performed if the trunk and chest wall were clinically more prominent than the corresponding features of the lumbar spine on examination in both the upright and forward-bending positions.
The concept underlying the initial analysis of a patient with a Lenke type 1 curve should be to fuse only the thoracic curve. With this approach, the surgeon seeks a reason to include the lumbar spine in the fusion rather than a reason not to do so. One of the predictors of success of a selective thoracic fusion is a preoperative thoracic Cobb angle to lumbar Cobb angle ratio greater than 1.2. Similarly, if the preoperative thoracic apical translation from the C7 plumb line is at least 1.2 times the lumbar apical translation from the CSVL, a selective thoracic correction will probably be acceptable. 20 Ratios of relative rotation can also be considered but, when based on plain radiography, will yield a less reliable assessment of axial deformity. Lastly, relative clinical deformity continues to be one of the most useful determinants of success in fusion surgery. The outcome of a selective thoracic fusion will not be favorable if the clinical deformity associated with the lumbar curve is more severe than that of the thoracic curve.
If a selective thoracic fusion for a Lenke 1C curve is attempted and the patient’s spine decompensates to the left, the opportunity exists to add the lumbar spine to corrective surgery at any time in the future. If the surgeon “plays it safe” and includes the lumbar spine unnecessarily, the patient may be predisposed to a greater risk of lumbar degenerative disease that might have been avoided. At some point, the contrary argument can be made that in the long term, a large residual lumbar deformity would be better fused but straight. The degree of lumbar deformity with the intact motion that would in the long term yield the best outcome if treated by fusion at an early point has been ill-defined and undetermined. Even the strongest proponents of preserving motion in the lumbar region would in most cases prefer lumbar curves of less than 40 degrees after selective thoracic fusion.
The balance between maximizing correction and maintaining lumbar motion is critical. Newton et al 26 defined values for quantifying this postoperatively using the deformity–flexibility quotient (DFQ). The DFQ consists of the postoperative residual lumbar deformity divided by the number of unfused motion segments (Fig. 16‑8). All other aspects being equal, a lower DFQ implies a better outcome (less deformity and more motion). This concept has been validated in two ways within the HSG. First, the members of the HSG were asked to compare pairs of postoperative radiographs of varying degrees of lumbar deformity and LIVs. Use of the DFQ predicted with high probability those radiographs that the group considered “most ideal.” Additionally, the DFQ was calculated retrospectively for 155 AIS patients in the HSG database, and a lower DFQ was statistically correlated with greater satisfaction scores on the SRS-24 instrument at 2 years postoperatively. Following patient outcomes over a longer period is critical to assessing the success of a fusion, and correlating a patient’s DFQ with these outcomes may be of future value in deciding whether to do a selective or nonselective fusion.

With this in mind, some idea of the factors that predict spontaneous lumbar curve correction (SLCC) is required in preoperative decision-making. Patel et al 27 found an average of 50% SLCC after selective thoracic fusion. Radiographic features that correlated with the percent SLCC included the level of the LIV, lumbar curve flexibility, and percent thoracic curve correction. In no case did the SLCC reach the degree of correction seen on the lumbar bending film, which always exceeded the correction ultimately obtained.
16.3.3 To What Extent Should the Thoracic Curve Be Corrected for Ideal Balance?
An ideal balance exists when the head and trunk are centered over the pelvis in the coronal and sagittal planes. If both lumbar and thoracic curves are present in a case of scoliosis, they are generally of similar magnitude when the trunk is coronally balanced. The greater the lumbar curve as compared with the thoracic curve, the greater the risk of decompensation to the left. If the patient’s trunk is preoperatively shifted to the left, it is hard to imagine how a selective thoracic fusion could do anything but worsen this; yet, in fact, this is not always the case. Lumbar curve correction following selective fusion is not always predictable.
The safest approach to selective thoracic fusion in the treatment of Lenke type 1C curves is to limit the degree of coronal plane correction of the thoracic curve obtained during surgery. Correcting the thoracic curve to 50% of the magnitude of the preoperative lumbar curve and assuming an average of 50% SLCC should limit the risk of decompensation. Intraoperative radiographs can assist in determining the amount of correction. However, this may overestimate the SLCC, because it does not include the effect of gravity. Correction of a thoracic apical lordosis should be maximized, as this may relieve the torsional pressures that result from the relative anterior overgrowth of the spine after fusion and may in fact improve SLCC. This concept requires further investigation with 3D data sets.
Through the years there have been conflicting data about whether an anterior or a posterior approach yields the greatest SLCC. Early studies suggested more SLCC with an anterior approach. More recent analyses, using third-generation instrumentation, suggest better correction with posterior methods. A comprehensive review of the HSG database, using multivariate regression analysis, determined that the LIV was important in determining the percent SLCC. 27 Because posterior instrumentation was traditionally longer than that used in anterior procedures, some of the “spontaneous” correction with the use of posterior techniques was in fact due to the result of instrumented correction of the upper vertebra of the lumbar curve. When controlling for the LIV, there was no difference in SLCC in 28 cases treated with an anterior approach and 28 matched cases treated with a posterior approach.
The response of the upper thoracic curve to MT curve correction should also be considered. Whether appropriate or not, relatively little value is given to the upper thoracic motion segments in selective fusion. Rather, the trend seems to include more of the upper thoracic spine, especially with increasing correction of the MT curve. Suk and colleagues 28 have stated that the upper thoracic curve should be included if the right shoulder is less than 1 cm higher than the left. Side-bending to less than 25 degrees is especially arbitrary in the upper thoracic spine, and this measure does not give enough information about when to include this region in a fusion. It is not clear whether a greater attempt should be made to preserve upper thoracic motion segments, limiting thoracic correction to preserve both balance (of the shoulders) and motion. Some nonstructural upper thoracic curves should be treated. Management strategies for these curves can be found in the discussion of Lenke type 2 curves.
16.3.4 What Levels Should Be Included in the Fusion?
After the curves to be instrumented have been chosen in planning a selective fusion, selection of the precise upper and lower limits for the fusion of a given curve remains a major challenge. There is often disagreement about this among surgeons; the most appropriate levels may depend on the surgeon and the surgeon’s particular techniques of correction. As previously discussed, there are two patterns of right thoracic curves, those with a lumbar curve (Lenke types 1AL, 1B, and 1C) and those without a true lumbar curve (Lenke type 1AR). We believe that these two patterns require different rules for selecting the vertebral levels for caudal fusion.
For the more common thoracic curves with an accompanying minor lumbar curve, the LIV is chosen as the stable vertebra 29 of the thoracic curve, and is generally between T11 and L1 (Fig. 16‑3). The concern with these types of curves is extending the fusion more distally than the stable vertebra, which increases the risk of decompensation to the left. In contrast, for Lenke type 1AR curves, the LIV is selected as the most proximal lumbar vertebra that is substantially “touched” by the CSVL (Fig. 16‑1). This is generally one to two levels distal to the stable vertebra, most frequently at L2 and L3, and always at or distal to the end vertebra. Thus, in all cases the entire measured Cobb angle should be included in the instrumentation. These rules are applied to posterior instrumentation with pedicle screw implants at the distal extent. For anterior constructs, the levels fused are the levels included in the Cobb angle, with the exception that if there is a parallel disc at the distal end of the curve, the level below the parallel disc should also be included (Fig. 16‑9).

As mentioned earlier, the selection of the distal level of fusion remains controversial. Suk et al evaluated the relationship of the LIV to the neutral vertebra. 30 They reviewed 203 patients with major thoracic curves who were followed up for a minimum of 5 years after fusion. When the end vertebra of the Cobb angle and the neutral vertebra were within two levels of each other, they recommended fusing to the neutral vertebra. However, when the neutral vertebra was more than two levels from the end vertebra, fusion extending to the neutral vertebra minus 1 was recommended. In cases in which the fusion was extended to neutral vertebra minus 2, unsatisfactory results were more frequent. Unsatisfactory results were described as a truncal shift of more than 2.0 cm decompensation, or extension of the primary curvature (adding-on).
In determining fusion levels, it is also important to consider any sagittal deformity. Consideration should be given to crossing a TL junctional kyphosis of greater than 10 to 15 degrees. Similarly, the occasional globally hyperkyphotic patient may require a more distal extention of fusion to L2 or L3, resembling what is done in a patient with Scheuermann kyphosis.
Choosing the proximal extent of fusion is also controversial and is often less emphasized than choosing the distal level. However, given the frequency of proximal junctional kyphosis and shoulder imbalance in patients with idiopathic scoliosis, the importance of this decision should not be underestimated. At the least, the proximal end vertebra should be included in the fusion. However, the appropriate levels to include above the proximal end vertebra depend on the degree of planned correction of the MT curve, preoperative shoulder balance, and proximal sagittal alignment. When the right shoulder is elevated preoperatively and correction of the thoracic curve will be limited (to preserve balance with a lumbar curve), the upper instrumented vertebra (UIV) may be safely chosen as the uppermost level of the Cobb angle. Level shoulders preoperatively require an inclusion of some portion of the PT curve to limit postoperative left-shoulder elevation. Similarly, patients with preoperative left-shoulder elevation will have even greater shoulder asymmetry if most of the PT curve is not instrumented. These concepts apply when maintaining shoulder balance as a surgical goal; however, the “physiological cost” of fusion in this region of the spine is poorly understood.
16.3.5 What Is the Best Approach?
In general, the spine can be fused from either an anterior or a posterior approach or both. Three surgical options exist for Lenke type 1 curves: (1) open anterior spinal fusion (OASF), (2) thoracoscopic anterior spinal fusion (TASF), and (3) posterior spinal fusion (PSF). The HSG recently published a prospective study designed to identify the optimal surgical approach for Lenke type 1 MT idiopathic scoliosis. 31 One hundred and forty-nine patients (17 OASF, 55 TASF, 77 PSF) were included from seven spinal-deformity treatment centers in Europe and North America, and 91% of patients had a minimum 2-year follow-up. All three approaches resulted in similarly satisfactory outcomes for the majority of patients. The patients with PSF had more level fused, but with the shortest operative time. The TASF group had the smallest incisions and the lowest requirement for transfusion. The OASF group had a modest loss of pulmonary function without any clear advantages compared with the other two groups.
During this prospective study, the use of thoracic pedicle screws became more popular and accepted. This improvement in posterior technique seems to have swung the pendulum back in favor of posterior methods among most surgeons. The isolated open anterior approach to fusion in thoracic scoliosis has been nearly abandoned because of its approach-related morbidity in terms of both trunk musculature and pulmonary function. Some surgeons and patients continue to choose thoracoscopic correction in select situations. The thoracoscopic approach remains the least invasive option but continues to come with substantial technical demands. Correction of scoliosis without a posterior incision is possible but requires more operative time and is associated with a high rate of pseudarthrosis (~5 to 10%), particularly in the early phase of a surgeon’s learning curve.
16.3.6 When Is an Anterior Release Indicated?
Traditionally, anterior release and fusion followed by posterior instrumentation has been utilized in treating severe scoliosis (curves > 75 degrees) to increase curve flexibility and improve the correction of deformity and for younger patients at risk for developing a crankshaft deformity. 32 , 33 , 34 , 35 , 36 However, the indications for an anterior release in MT scoliosis have been changing, particularly with the increasing use of pedicle screws in the thoracic spine.
In 2005, Luhmann and colleagues 37 reviewed 84 patients with MT curves of 70 to 100 degrees in a comparison of anteroposterior spinal fusion (APSF) with purely PSF. They concluded that APSF allowed greater coronal correction than did posterior thoracic hook or hybrid constructs; however, the correction was similar with the use of posterior constructs having only pedicle screws. In terms of postoperative sagittal alignment, no differences were found in the treatment groups. In 2007, Suk and colleagues 38 reported obtaining better than 60% correction of deformity in AIS and maintenance of the correction at 2-year follow-up with the use of posterior segmental constructs having only pedicle screws in patients with curves of 70 to 100 degrees. They also found no evidence of the development of crankshaft deformity in immature patients older than 9.8 years, proposing that pedicle screws were better able to control all three columns of the spine than were other anchors as growth continued. Suk and colleagues recommended changing the indications for anterior release to curves greater than 110 degrees having flexibility of less than 20% when using segmental pedicle screws.
There is little doubt that thoracic pedicle screws can apply a greater corrective force to the spine in all three planes of motion than can other anchors and prevent the cardiopulmonary complications of an anterior approach; however, the indication for an anterior release is to some extent based on the desired result. It is simple enough to say that with current posterior methods, an anterior release is never required; however, there are no published studies comparing anterior release with posterior pedicle screw fixation to posterior pedicle screw constructs alone. For severe curves, a purely posterior approach will either result in less satisfactory correction or be associated with a more aggressive posterior procedure consisting of pedicle subtraction or vertebral resection osteotomies. The ideal treatment should balance the risk of the procedures used with the benefit of the correction achieved.
Although there is clear support for avoiding an anterior release for many curves that in the past would have been treated with one, it is open to question whether the overall result would in fact be better if an anterior release were used to shorten the anterior column in addition to the powerful posterior techniques now available. The use of an anterior approach should be based on the 3D thoracic deformity that exists in addition to the flexibility of the thoracic curve. Thoracic curves greater than 75 degrees that are associated with severe apical lordosis and an angle of trunk rotation greater than 25 degrees as measured with a scoliometer remain an indication for thoracoscopic anterior release in the view of some surgeons (Fig. 16‑10).

Beyond the initial learning curve, 39 thoracoscopic procedures for anterior release are associated with little morbidity. Disc removal in these cases allows the shortening of the anterior column that is critical in achieving both axial-plane correction and the restoration of thoracic kyphosis, particularly at the apex of the thoracic curve. Derotation in the transverse plane is associated with increasing anterior spinal length. Without an anterior column shortening (by disc excision), and often a posterior apical lengthening (by Ponte-type osteotomies), complete 3D correction of a scoliotic curve is impossible. Ultimately, the decision to include an anterior procedure should be made on a case-by-case basis according to the time, risk, and morbidity entailed by either an open or a thoracoscopic release.
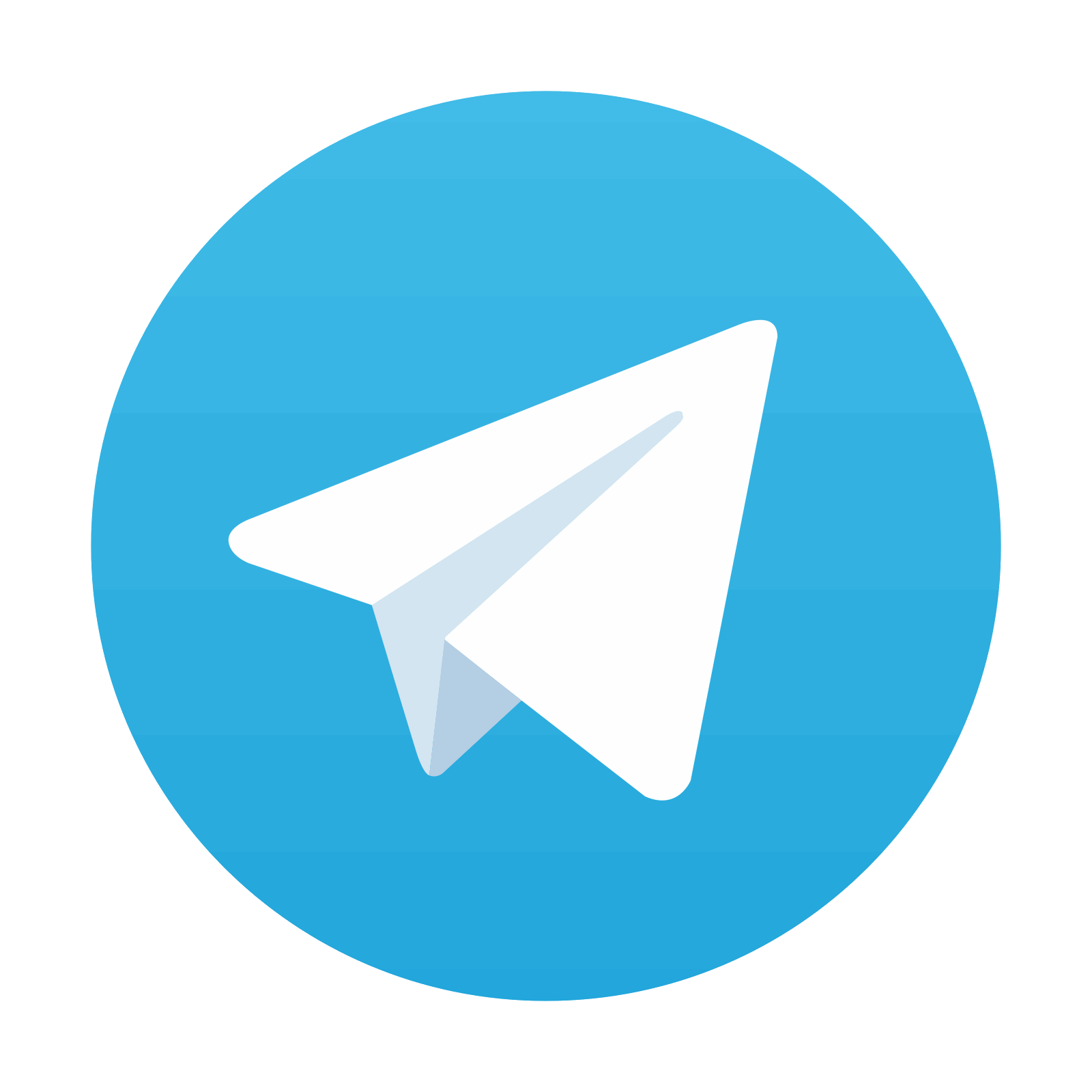
Stay updated, free articles. Join our Telegram channel

Full access? Get Clinical Tree
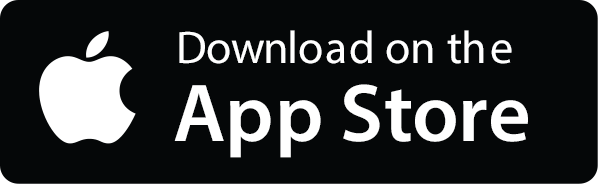
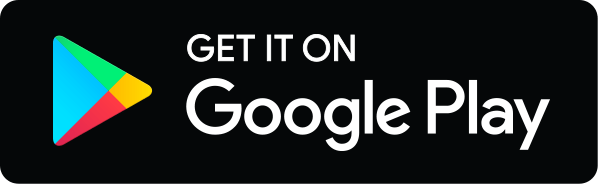
