Summary
Posterior correction of the three-dimensional deformity of idiopathic scoliosis requires selection of levels to instrument, appropriate placement of anchors that are strongly fixed to the posterior elements, and rods of a material that overcomes the inherent stiffness of the spine without compromising the anchor fixation. Applying and manipulating the spinal screws and rods may allow correction of deformity by translation and cantilever maneuvers. Manipulating the spinal instrumentation after insertion may also allow correction through rod rotation, compression/distraction of anchors, in situ rod contouring, temporary working rods, and various types of vertebral derotation. Spine flexibility may be improved through the posterior element releases and intraoperative spinal traction.
Key words
posterior correction – implant properties – rod manipulation – vertebral derotation12 Posterior Correction Techniques in Adolescent Idiopathic Scoliosis
12.1 Introduction
Idiopathic scoliosis is a three-dimensional (3D) deformity with lateral deviation in the coronal plane, thoracic hypokyphosis in the sagittal plane, and rotation in the transverse plane. With pedicle screw fixation and modern corrective techniques, true 3D correction of the spinal deformity in scoliosis can be accomplished. Intuitively, in typical thoracic adolescent idiopathic scoliosis (AIS), this would mean optimal coronal correction, restoration of normal thoracic kyphosis, and realignment of thoracic torsion by lifting the thoracic rib concavity out of the chest and reducing the convex rib deformity without the need for thoracoplasty. There is no single technique for the surgical correction of scoliosis that will work in every situation. Careful preoperative planning to maximize the usefulness of implants and observation of the correction intraoperatively are mandatory for success. Criteria to be considered are curve type, spinal flexibility, the material properties of the implants being used, the type of vertebral fixation to be used, and anticipation of the reduction techniques that will be needed. With respect to spinal flexibility, an assessment of how much correction is possible or necessary should be made. Both coronal and sagittal plane flexibility should be considered; coronal flexibility is readily apparent on bending or traction films, but assessment of sagittal plane flexibility, especially thoracic lordosis, has been elusive. Hypokyphotic or lordotic thoracic deformities are often very difficult to reduce with posterior-only techniques. Is a release required to achieve the necessary correction? If so, what sort of release is needed? Is a simple intraspinous ligament resection with facetectomy sufficient, or is some sort of osteotomy required? Flexible spines usually respond to any reduction maneuver. Stiff spines may defy all attempts at reduction until appropriately mobilized with a combination of techniques.
12.2 Implant Properties
The material properties of implants are an important consideration in preoperative planning for the surgical treatment of scoliosis. By varying the diameter and composition of a rod, a surgeon can match the stiffness of the rod to the stiffness of the patient’s spinal curve, the quality of the patient’s bone, or both. Stainless steel (SS) has distinct advantages in correcting spinal deformities. The stiffness and strength of SS are beneficial when reducing the spine to the rod because there is greater corrective power in conforming the spine to the rod and less potential for rod deformation. For example, for a stiff, lordotic thoracic scoliosis, SS would provide a better correction in both planes than would a titanium (Ti) rod, which would be expected to bend into the deformity and provide less corrective ability. However, there is such a thing as a rod that is too stiff; thus, if the stiffness of a rod exceeds the strength of the bone–implant interface, a pullout of the implant is possible. The bending characteristics of SS allow its in situ contouring for challenging coronal and sagittal plane deformities because SS undergoes plastic deformation over a narrower range than Ti because of the latter’s greater elasticity. Although SS is stiffer and stronger than Ti, and Ti is more elastic, both materials are acceptable once a construct is assembled because construct rigidity also depends on the number and type of anchors for the construct.
Ti has other cited advantages in the areas of imaging, infection, corrosion, and metal-induced sensitivity. 1 , 2 Because Ti does not create as many artifacts as SS, better postoperative computed tomography (CT) and magnetic resonance (MR) imaging with Ti has made it more popular than SS, especially in situations in which visualization within the spinal canal or evaluation of adjacent segment degeneration is valuable for clinical decision-making. Some centers favor the use of Ti for its resistance to infection in that it does not easily allow the formation of bacterial glycocalyx on its surface, which could permit it to harbor organisms that might cause deep late-onset infection. Moreover, the surface composition and ion charge of Ti are friendlier to osteoblasts than are those of SS. Explant corrosion studies cite less corrosion in Ti constructs, which may also be a factor in decreasing infection since Ti tempers host immune responses. 3 Furthermore, Ti is a better choice of construct material for patients who have a known sensitivity to nickel, a component of the SS alloy.
For surgeons who favor Ti implants but are frequently disappointed by their lack of strength and stiffness in an application for treating a spinal deformity, cobalt chromium (CoCr) rods may offer a satisfactory compromise. The material properties of CoCr are close to those of SS in rods of the same diameter and are compatible with Ti spinal anchors, with the result that a surgeon seeking to use Ti implants may not need to give up corrective capacity when using CoCr rods. Other materials that may be available in the future for use in corrective constructs are nitinol, a superelastic nickel–titanium alloy that has shape-memory properties, and dynamic rods made of polyetheretherketone (PEEK) or polyethylene polymers. Recent work has also supported use of “mixing metals” so that there is no hesitation if a surgeon uses Ti screws with SS rods, for instance.
12.3 Other Rod Materials
A properly contoured and applied rod provides most of the correction of a spinal deformity during surgery (unless intraoperative traction is also employed). Many reduction maneuvers can be applied during such correction. The most effective of these maneuvers are performed while only one rod is in place. The second rod adds stability and resistance to fatigue failure of the corrective construct. Occasionally, temporary or working rods may be required to facilitate reduction. These working rods will usually be placed on the side opposite that of the correcting or primary rod. Three-column osteotomy stabilization, large curves with nonharmonious sagittal segments, or single long curves containing different sagittal contours are common indications for using temporary or working rods.
To a great degree, the techniques used to correct spinal deformities depend on the spinal implants used for the fixation of corrective constructs. Reduction techniques possible with first-generation implants using nonsegmental hooks (Harrington rod constructs) were limited to the global en bloc distraction of multiple vertebral segments, correcting a deformity in the coronal plane, often at the cost of correcting it in the sagittal plane. Second-generation implant systems using “segmental hook” constructs (Cotrel–Dubousset constructs) allowed some flexibility in applying distraction as well as compressive forces across a deformity. 3 Although the hook-and-wire–based systems provide some additional options for reducing spinal deformities, they fall short in several important ways. First, even segmental hook systems do not allow truly segmental fixation (i.e., fixation at every vertebral level treated with a construct), and, second, neither hook nor wire constructs provide rigid fixation, and therefore complete control, over the vertebral segments included in a corrective construct. Currently available pedicle screw systems overcome both of these failings by allowing truly segmental spinal fixation at every level as well as direct control over each vertebral segment included in a construct since it is the only implant to extend past the instantaneous axis of rotation (IAR). Now, with the power to directly control each individual segment, the ability to reduce a spinal deformity has more to do with the adequacy of release (i.e., spinal mobilization), the creativity in designing reduction maneuvers that consider the response of the spine during reduction, and the persistence of the surgeon in attempting to achieve a complete reduction of the deformity.
The types of devices used for vertebral body fixation will also dictate or influence reduction strategies. Fixed-angle, uniplanar, and polyaxial reduction screws (including those that can lock the screw head articulation independently of the rod connection), hooks, and wires or bands will lend themselves to specific reduction strategies. The type of implant and its position in a construct should be considered in the preoperative plan for its use.
12.4 Correction Maneuvers
The following sections describe a variety of techniques and maneuvers that can be applied individually or in combination to achieve the reduction of spinal deformities. Although at one time the technique for reduction of scoliosis was dictated by the implants to be used or by philosophical constraints, multiple techniques can now be used to achieve the correction of a deformity. Because not every technique will work equally well in all situations, the success of a correction for scoliosis depends on the adequacy of spinal release and the skill and experience of the surgeon in applying the techniques used for the correction.
12.4.1 Compression–Distraction
Distraction on the concave side of a construct decreases scoliosis. In the thoracic spine, distraction also increases thoracic kyphosis, which is generally desirable in view of the frequent loss of normal thoracic kyphosis noted in idiopathic scoliosis. Compression is useful to reduce hyperkyphotic thoracic deformities. Similarly, compression applied to implants along the rod on the convex side of a lumbar deformity corrects scoliosis, shortens the spine, and restores or maintains lumbar lordosis. When compression and distraction are used as primary reduction maneuvers, it is important to remember the kyphosing and lordosing effects of these techniques so as not to negatively affect spinal balance in the sagittal plane. However, when compression–distraction is used primarily as a technique to refine a reduction after both rods of the corrective construct are in place and appropriately contoured, the positive and negative consequences of intrasegmental compression and distraction on the patient’s sagittal profile are probably negligible. This segmental “fine-tuning” is particularly enhanced by using bilateral segmental pedicle screws.
The possible adverse effects of the compression–distraction technique include transmission of asymmetric forces to adjacent levels of the spine and especially to adjacent uninstrumented levels, resulting in junctional malalignment. The use of excessive forces may loosen implants. Fixed-angle screws do not lend themselves to this technique because they need to either plow through the pedicle or return to their previous orientation (precompression or distraction) when the set screws are tightened, and the screw head must be perpendicular to the rod for a secure connection. Uniplanar or polyaxial screws are effective in compression–distraction maneuvers. Distraction of the thoracic spine to induce kyphosis in the typical hypokyphotic situation in AIS is more effective when adequate releases of the facets and ligamentum flavum are achieved, but this should be done with intraoperative monitoring.
12.4.2 Rod Derotation Maneuver
For a typical lordotic thoracic curve (Fig. 12‑1), the classic “derotation” maneuver of Cotrel and Dubousset may be applied. 4 A rod contoured for the coronal plane deformity of the curve is placed in the screws on the concave side of the deformity. The set screws should be engaged within the screw head but not tightened. The rod is then rotated into the correct position and the second rod is placed. This technique theoretically converts lateral deviation into deviation in the sagittal plane. Given that thoracic curvatures are often hypokyphotic, this maneuver can often both correct coronal plane deformity and restore natural thoracic kyphosis. However, some points should be made about this technique. Most important is that the derotation with this en bloc derotation maneuver really amounts to lateral translation or an in situ relocation of the apex of the treated curve. In addition, the desired sagittal contour is sometimes not concordant with the coronal plane of the scoliotic deformity. Hence, the conversion from a coronal plane deformity to kyphosis or lordosis may occur at the wrong location. Moreover, the rod used for the derotation must be stiff enough to maintain its contoured shape, the bone must be strong enough to withstand screw pullout, and the spine must be flexible enough to be displaced posteriorly. This technique may not work well with Ti rods, which are more flexible than SS or CoCr rods and have a tendency to “bend out” and lose the sagittal contour that was created in them before their implantation.

Proponents of the Cotrel–Dubousset procedure believed that rotational correction of a scoliosis would occur with the rod derotation maneuver. This was later disproved with pre- and postoperative CT scans of instrumented patients. Labelle et al 5 used 3D digitizers intraoperatively and showed that the Cotrel–Dubousset rod derotation maneuver did produce coronal and sagittal plane correction and relocated the instrumented portion of the spine, but with little axial plane correction. In fact, the rod rotation maneuver may aggravate the rib prominence in a stiff curve temporarily when the concave rod is being implanted. Hook-and-wire fixation lacks the ability to derotate the spine because force is applied posterior to the IAR and the moment arm is inadequate to apply sufficient torque. True correction of vertebral rotation in the axial plane, and consequently the elimination of the convex thoracic or thoracolumbar (TL) prominence in scoliosis is difficult to achieve without posterior release/osteotomy or anterior discectomy and chest wall violation with thoracoplasty.
12.4.3 In Situ Contouring
With the use of appropriate bending tools for the rods used in a corrective construct, in situ contouring in both the coronal and sagittal planes can improve spinal alignment in scoliosis. This is a very useful technique for reestablishing the coronal contour of the spine if the rod used in the procedure bends out during rod rotation. It is much easier to effectively change the coronal plane than the sagittal plane once all the implants used with the rod are engaged on the rod. In situ sagittal rod benders are often very useful in working a rod into the vertebral implants for a corrective construct during difficult rod-to-implant reductions. Sagittal rod benders are also very helpful in creating lumbar lordosis. With the use of either technique, great care should be exercised to prevent the catastrophic failure of implant fixation in the vertebral body. This is especially a concern when using hooks for vertebral fixation. In situ contouring is not a useful technique with Ti rods because they require too much bend (deformation distance) to reach the plastic deformation zone to make this technique practical. The in situ contouring technique also does not work well for reducing thoracic hypokyphosis.
12.4.4 Coronal and Sagittal Translation
Pure translation is very effective for correcting thoracic curves. This can be achieved with screws, bands, or sublaminar wires on the concave side of the apical and periapical vertebrae of a thoracic curve. Reduction screws can be implanted in the four or five apical and periapical levels of a deformity to facilitate rod placement and correction of the deformity, or if rod reduction instruments are required. The sequence of steps in reduction by translation involves first placing the rod in the distal or proximal implants, which are then captured loosely—the spine needs to lengthen as it is corrected. Next, the rod is sequentially reduced into each adjacent implant, including the apical implants. Apical screws are initially left untightened. Rings should be placed on reduction implants to prevent premature release of the flanges of the reduction screws. Following this, the rod, which has been precontoured into the desired sagittal contour and left straight in the coronal plane, is rotated into the correct sagittal and coronal orientation, if necessary. The inner screws on the reduction implants are then slowly and sequentially tightened to achieve the reduction of the spine by pulling the spine to the rod. This results in the correction of scoliosis and produces posterior sagittal plane translation, increasing kyphosis. A benefit of this technique is that it allows slow reduction of the thoracic deformity in scoliosis, taking advantage of viscoelastic creep. It also allows the forces of reduction to be distributed over multiple segments of the deformity. The screws serve as both the final implants and the tools for reduction, eliminating the need for any additional instrumentation in the operative site. The extended tabs allow rotation of the rod into the final position with little stress applied to the rod. This helps prevent rod “bend-out.” Translation with reduction screws is not as effective with stiff curves or with Ti rods for the reasons mentioned earlier. Care must be taken to look for the pullout of anchoring screws. To some degree, this is prevented by assessing the flexibility of a curve and the strength of the patient’s vertebral bone and being satisfied with a reasonable reduction. Maximizing the diameter, length, and position of a screw within a vertebral body may also help prevent screw pullout.
12.4.5 En Bloc Vertebral Derotation
An alternative technique for reducing a scoliotic curve is regional vertebral derotation. This technique is used after the Cotrel–Dubousset procedure of rod derotation when the rod is in its final position. The maneuver involves the direct application of force to the entire periapical segment of a deformity. This is achieved by placing devices on the apical pedicle screws that facilitate a derotation moment to be imparted to three or four of the periapical vertebrae of a deformity. This produces derotation around the concave rod. Once this is done, the set screws are tightened to hold the treated vertebrae in place.
12.4.6 Segmental Vertebral Derotation
The challenges in the 3D correction of scoliosis with posterior-only surgery are well addressed with the use of segmental pedicle screw fixation, specifically the application of direct vertebral rotation, first described by Lee et al. 6 Pedicle screws extend into the vertebral body anterior to the IAR and can be manipulated with the use of long derotator instruments attached to the screw heads to achieve true 3D correction of a scoliotic deformity. Segmental fixation with pedicle screws addresses the most rigid, rotated portion of the spine, spreads the corrective force over multiple implants, can pull the scoliotic rib concavity out of the chest, and will result in little loss of correction over time.
The concept of direct vertebral body derotation is the same as for regional derotation, but the derotation maneuver is applied to an individual vertebral segment. This technique allows segmental derotation incrementally in the same way that repeated compression and distraction maneuvers can be used to incrementally improve coronal alignment. To use the technique, the concave side screw in the treated vertebra must be loose during the derotation step. It may also be beneficial to have the mobility of the vertebrae above and below the derotation site. The sequence of steps in the procedure can be initiated from either end of the treated vertebral segment, but the authors favor starting from the lower instrumented vertebra (LIV). The distal end of the construct is stabilized by tightening the set screws, and the adjacent level is then derotated by directly applying a derotation moment to the vertebra through the screw(s) to bring that level to a neutral position. Once the desired derotation has been achieved, the set screws are tightened. This maneuver is then repeated at each instrumented level proceeding cephalad. Additional derotation can be achieved by repeating the process at each level until a satisfactory result is achieved.
The goals of vertebral derotation are to achieve true 3D correction of a spinal deformity and reverse the torsional asymmetry induced by scoliosis. Intuitively, in typical thoracic AIS, this would mean optimal coronal correction, restoration of thoracic kyphosis, and the realignment of thoracic torsion by lifting the rib concavity out of the chest and reducing the convex rib deformity. The upper instrumented vertebra and LIV would be level, and along with the apex of the curve would be brought into the stable zone as defined by the center sacral vertical line (CSVL). The rib prominence would be virtually eliminated without thoracoplasty.
Some technical considerations are important in executing a direct vertebral body derotation safely and effectively. Fixed angle or uniplanar pedicle screws offer better axial plane control of the vertebral segment being derotated than do polyaxial screws, 7 and an attempt should be made to use them in the strategic areas of the spine (i.e., the apex of the deformity). Uniplanar screw technology has become available and allows the cephalad/caudad movement of a polyaxial screw, although the screw remains fixed in the coronal and axial planes for vertebral body derotation maneuvers. Application of force to the screws in a construct being used for derotation should be slow, deliberate, and controlled, and depends on the bone mineral density of the patient’s spine and integrity of the bone–screw interface. More force can be applied to the screws on the convex side of a deformity because the corresponding pedicles are typically larger than the concave side pedicles in the apex of the scoliosis, 8 and the medial wall of the convex side pedicle is thicker than the lateral wall of the pedicle. 9 When rotated to failure, the convex side screws will fracture the medial wall of the pedicle and enter the spinal canal; the concave screws will fracture the lateral pedicle wall, rib/pedicle unit, and transverse process, and could injure the aorta. 10 Finally, care must be taken not to transfer torsional forces beyond the instrumented segments and to compensatory curves or neutrally rotated vertebral levels to create iatrogenic torsion; the neutral end vertebrae must be locked before derotation of the apical levels of the deformity being treated. 11
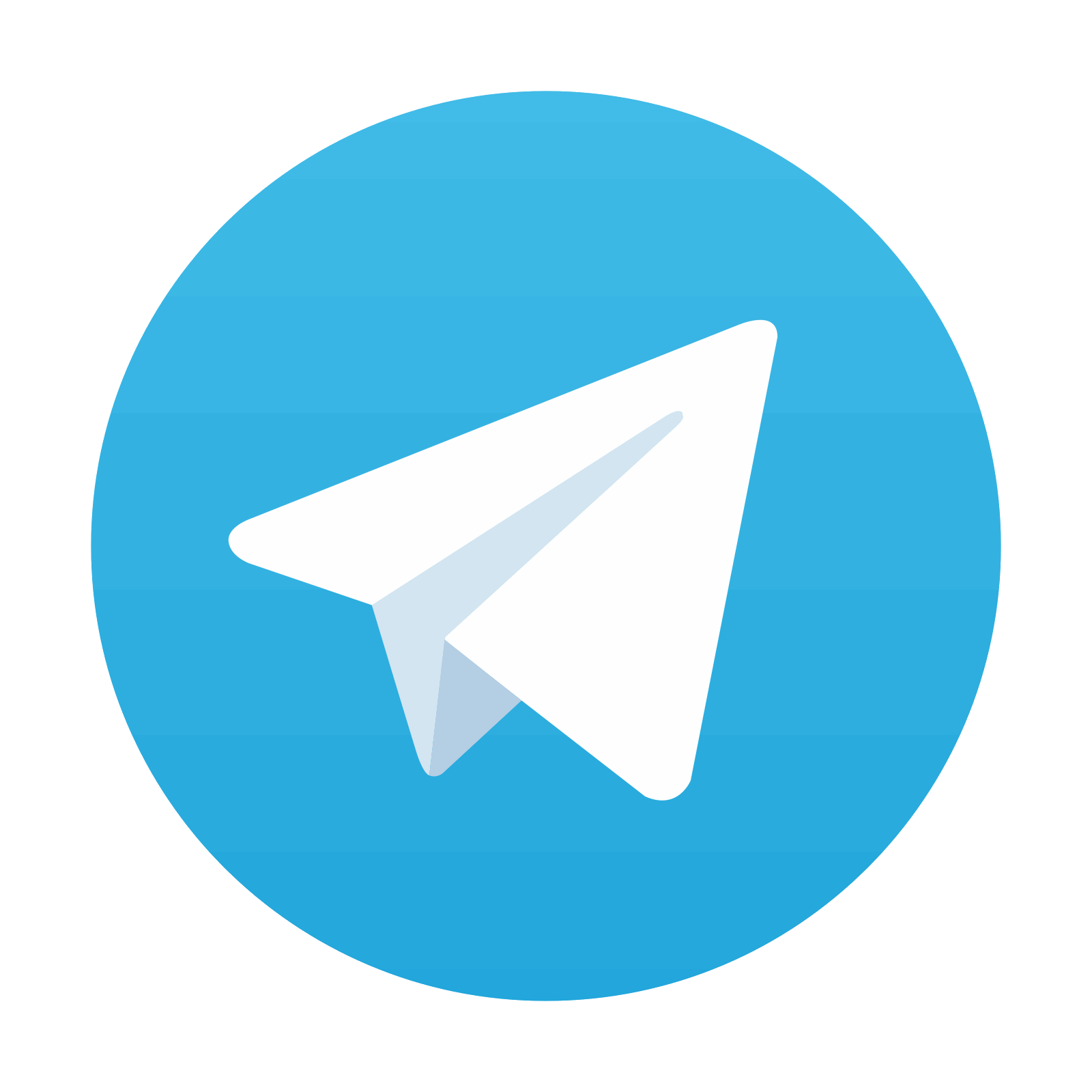
Stay updated, free articles. Join our Telegram channel

Full access? Get Clinical Tree
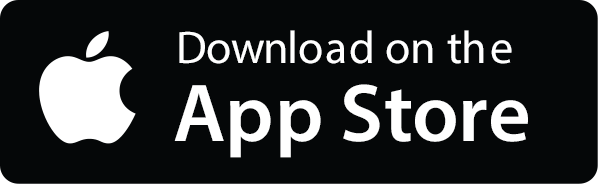
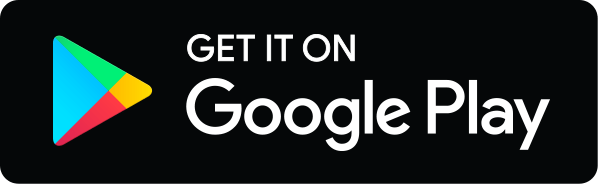
