Fig. 3.1
Stability of the rTSA construct against applied joint forces. rTSA construct is stable if the resultant force vector (F, black arrow) points within the cup (a). The stability is also dependent on the diameter (b) and the depth (c) of the cup as well as the radius of the hemisphere. Used by permission from [38]
Another factor that effects the stability of the construct is the depth of the humerosocket [15, 16]. However, although an increase in humerosocket depth is proportional to stability, it is inversely proportional to impingement-free range of motion for the joint [17]. This relationship, in turn, requires that a balance be maintained between stability and impingement-free arc of motion. As a result, many modern rTSA designs provide components with various glenosphere and socket radii in order to individually maximize impingement-free motion without compromising stability.
Although impingement-free glenohumeral motion of the rTSA construct has an undeniable impact on postoperative range of shoulder motion, it should also be noted that studies on shoulder kinematics following rTSA have demonstrated that scapular rotation is significantly increased [18, 19]. Kwon et al., for example, analyzed the postoperative kinematics of shoulder motion in rTSA and found that the contribution of scapulothoracic motion to overall humeral elevation was significantly increased when compared to normal subjects [19]. Furthermore, relative contribution of scapulothoracic motion to humeral elevation was even more pronounced when loads are applied to the extremity [19]. These findings suggest that shoulder motion after rTSA is affected by multiple factors and that isolated implant modifications to increase impingement-free motion may not significantly alter the overall shoulder motion after rTSA.
Medialized Center of Rotation
In a typical “ball and socket” joint, the center of rotation is maintained at the center of the convex surface. Thus, the center of rotation in an anatomic TSA and rTSA is at the center of the humeral head and at the center of the glenosphere, respectively (Fig. 3.2). Since the rTSA glenosphere is fixed to the native glenoid surface, the distance from the glenoid surface to the center of rotation is directly proportional to the mechanical torque about the component and the shear forces at the glenoid bone prosthesis interface.
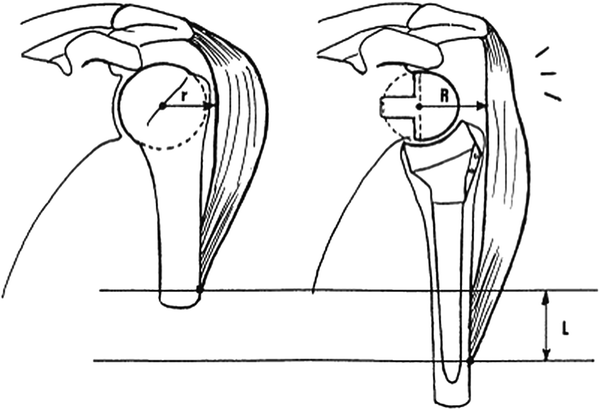
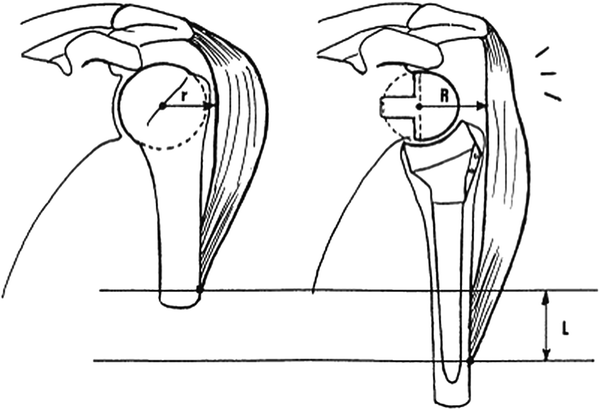
Fig. 3.2
Biomechanical changes of rTSA [9]. Reprinted with permission from Elsevier. In comparison with normal shoulder (left), rTSA construct (right) contains a medialized center of rotation with a greater lever arm for the deltoid and distalization of the humerus with increasing resting tension across the deltoid. Used by permission from [5], January–February 2005
As mentioned previously, the concept of the rTSA with a fixed fulcrum for rotation was originally adapted from the total hip arthroplasty designs. These original designs maintained the center of rotation of a normal shoulder joint. Unfortunately, fixation of the glenoid component could not withstand the shear forces created at the bone prosthesis interface and resulted in unacceptably high rates of early mechanical failures [9]. In order to address this issue, Grammont eliminated the “neck” of the previous implant designs by utilizing one-third of a sphere that was fixed directly onto the glenoid. This change medialized the center of rotation to the glenoid surface, effectively minimizing the shear forces across the glenoid bone prosthesis interface.
Peak joint forces across a rTSA are comparable to those across a normal shoulder [10]. However, in contrast to the normal shoulder where joint forces have been measured to be at their highest at 90° of elevation, the highest joint forces across rTSA occur at approximately 60° of elevation [10]. At 60° of elevation, a greater percentage of these joint forces are perpendicular, rather than parallel, to the glenoid surface. Medialization of the center of rotation thus effectively converts the mechanical torque at the glenosphere into more compressive forces across the prosthesis–bone interface and decreases the peak shear forces experienced by the glenoid component fixation [10, 20, 21]. In fact, in a cadaveric model, these joint forces across the Grammont-type rTSA led to micromotion of less than 60 μm on the glenoid baseplate, which is well below the 100 μm threshold believed to induce osteointegration [10, 22]. As a result, these alterations in biomechanics have made mechanical failures of the rTSA increasingly rare.
Another beneficial outcome of medializing the center of rotation is to increase the lever arm of the deltoid for elevating the arm [9, 12, 21, 23–25]. Since the deltoid originates at the borders of the acromion, the torque created by deltoid muscle contraction is directly proportional to the horizontal distance from the acromion border to the center of rotation. By medializing the center of rotation further away from the acromion border, the rTSA construct increases this “lever arm” for the deltoid [9, 12, 21, 26]. This, in turn, allows the deltoid to produce a larger torque to rotate the humerus and elevate the arm (Fig. 3.3). Ackland et al. confirmed this hypothesis by analyzing the performance of each muscle group about the rTSA shoulder and found a significant increase in the abductor moment arms for the anterior and middle deltoid muscles. In addition, they noted that the posterior deltoid muscle was also acting as an abductor in a rTSA construct, in contrast to its physiologic role as an adductor in normal shoulders [27].
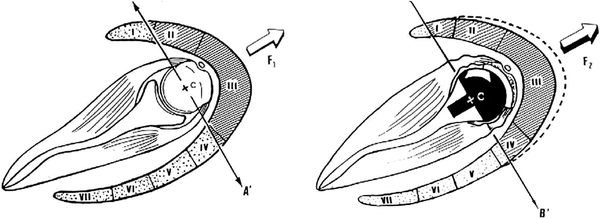
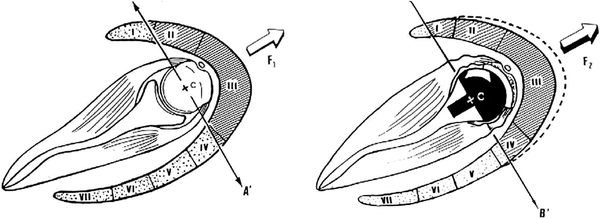
Fig. 3.3
Changes to the deltoid action within rTSA [9]. Reprinted with permission from Elsevier. While acting as an adductor in normal shoulders (left), the medial portions of the anterior deltoid (zone I) and the mid portion of the posterior deltoid (zone IV) acts as an abductor in rTSA shoulders (right) with a medialized center of rotation. Used by permission from [5], January–February 2005
Although medialization of the center of rotation clearly results in biomechanical improvements, there are also some potential disadvantages. First, while increasing the lever arm of the deltoid for elevation of the humerus, excessive medialization of the center of rotation will likely decrease the efficiency of the external rotators of the shoulder joint such as the infraspinatus and teres minor. As these muscles originate medial to the shoulder joint, medialization will consequently decrease the mechanical lever arm to externally rotate the shoulder. Perhaps as a result, clinical outcome studies with the Grammont design rTSA have demonstrated only minimal improvements in active external rotation [9, 16]. Second, medialization of the center of rotation also brings the infero-medial aspect of the humeral component against the inferior aspect of the scapula neck in adduction. Consequently, the humeral cup can impinge at the inferior scapular neck, causing polyethylene component erosion and inferior scapular notching (Fig. 3.4) [9, 28, 29]. This complication has a reported incidence of 50–96 % and is generally observed within the first six months following surgery [12, 30, 31].
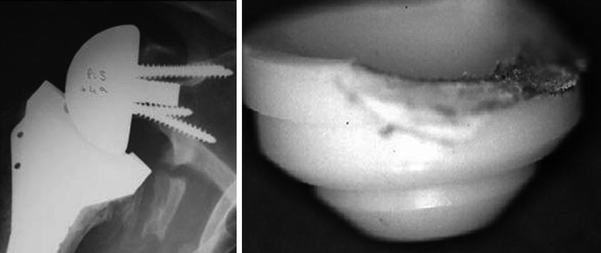
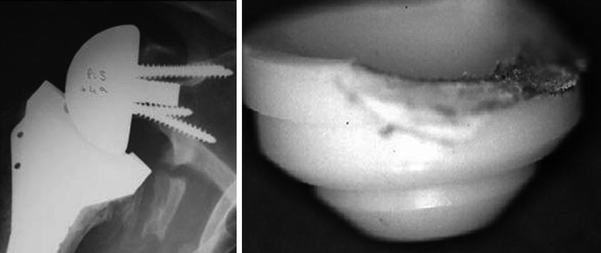
Fig. 3.4
Scapula notching of the Grammont rTSA design [9, 30]. Reprinted with permission from Springer (left) and Elsevier (right). With medialized center of rotation and non-anatomic humeral neck cut, the inferior medial portion of the humerus can impinge against the inferior glenoid neck and cause osteolysis (left) as well as polyethylene humeral cup wear (right). Left Used by permission from [39]. Right Used by permission from [5], January–February 2005
Given these potential disadvantages, some surgeons have advocated rTSA designs that place the center of rotation slightly lateral to the glenoid surface. As the center of rotation is still medial in comparison with anatomic TSA, the increased mechanical lever arm for the deltoid is mostly maintained. It has been demonstrated that this slight “lateralization” can also minimize the incidence of scapula notching as well as improve external rotation after rTSA [23, 24, 32]. An additional study has also confirmed that moving the center of rotation slightly lateral to the glenoid surface can minimize the amount of component impingement against the inferior scapular neck at even small angles of elevation, thus allowing for a greater impingement-free range of shoulder motion [33]. Critics, however, argue that this deviation from Grammont’s original design could potentially increase the shear forces at the glenoid bone prosthesis interface and result in early mechanical failures. Although this “lateralization” can result in increased joint forces, recent studies have demonstrated a potential greater compressive-to-shear force ratio when the center of rotation is slightly lateral to the glenoid surface, possibly negating the mechanical stress on the implant fixation [10, 20]. In fact, after cyclical loading in a cadaveric model, Kwon et al. noted that micromotion of glenoid baseplate in rTSA with this slight “lateralization” was always less than 65 μm, well below the 100 μm threshold believed to induce osteointegration [10, 22]. In addition, total migration of the glenoid baseplate after the cyclical loading for the rTSA with a slightly lateralized center of rotation was actually less than that for the Grammont design rTSA [10].
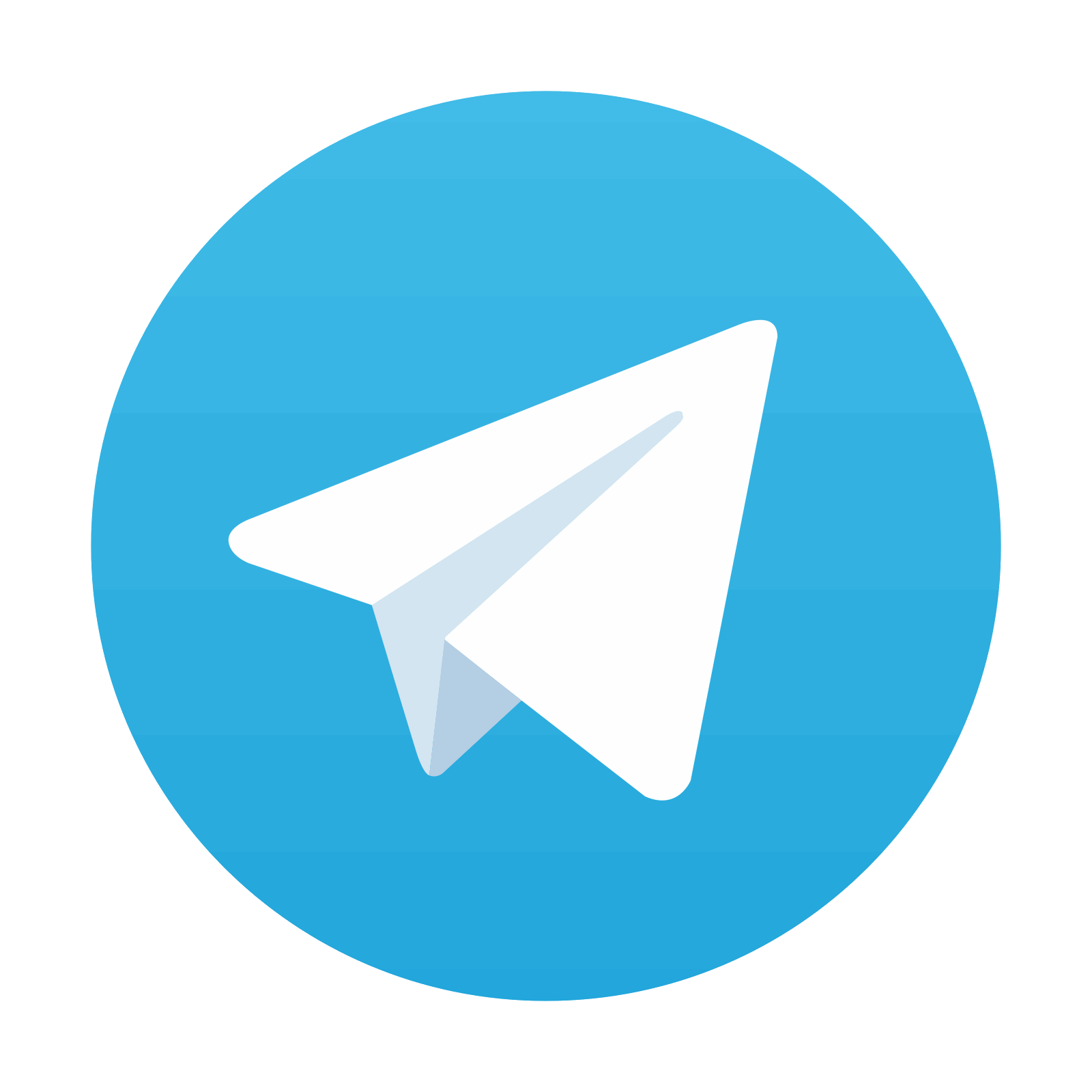
Stay updated, free articles. Join our Telegram channel

Full access? Get Clinical Tree
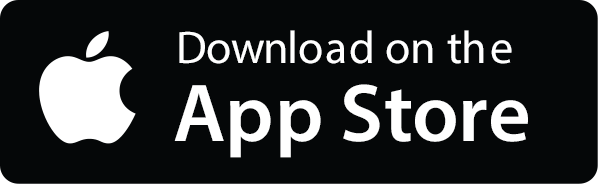
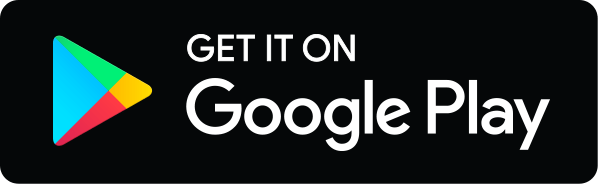