Fig. 7.1
(a) Transversal ultrasound image shows a complete rupture of the quadriceps muscle. (b) Intraoperative view of the quadriceps lesion. (c) The lesion after sutures. (d) Final aspect of the suture
Partial tears with an avulsion involving one or two tendons with greater than 2 cm retraction, or a musculotendinous avulsion injury to the direct head of the rectus femoris that is symptomatic for more than three months should be considered for an operative management, depending on the severity of symptoms, or functional limitations in patients unwilling or unable to modify their activity levels [15].
7.4 Return to Play and Prevention
Reconditioning the injured muscle group is mandatory. Return to play should be based on the patient’s ability to stretch the injured muscle as much as the contralateral healthy muscle, pain-free use of the injured muscle in sport-specific movements (mainly in eccentric exercise), comparable strength between injured and healthy muscles and functional tests [16].
There are strategies that can be adopted to minimize the recurrence rate and to enhance performance.
- A.
An appropriate warm-up is protective because it increases range of motion and reduces stiffness. Warm up should occur before any exercise session or sports activity, including practice. This will help increase speed and endurance [17].
- B.
Avoid muscle tightness by stretching slowly and gradually, holding each stretch to give the muscle time to respond and lengthen.
- C.
A regular program of strengthening exercises should be instituted.
- D.
Recovery of strength and muscular endurance should focus on the transitional period due to inactivity and deconditioning. The relative state of fatigue may be increased making the muscles more susceptible to injury. Training with low levels of resistance and many repetitions should be recommended [4, 18, 19].
7.5 Future Therapies
Novel treatments for muscle healing—such as the use of growth factors and cytokines—are still under investigation in Europe and have not been approved by the U.S. Food and Drug Administration (FDA). Hyperthermia is emerging as an effective treatment for some injuries. Rapid, precise application of heat at the injured site improves and accelerates the healing process. It stimulates muscle precursor cells and protein synthesis.
Gene and cellular therapy appear to be the two main future therapies for muscle injury treatment. Gene therapy is the addition of genes into cells with the aim of restoring function, establishing new cells or even enhancing already existing activities through the expression of introduced genes [20]. Thus, gene therapy can be applied to most diseases, whether genetic or acquired. The therapeutic gene is introduced into the patient in two ways: in vivo or ex vivo. The choice of one type or another depends on a number of factors such as the molecular pathology of the disease, the target cell, the size and type of the therapeutic gene and the duration of the therapy [20]. The system used for transferring therapeutic genes into the target cell is called a vector which can be a viral-based structure or a plasmid [20].
A major obstacle to the application of gene therapy in clinical practice is the construction of suitable vectors to ensure patient safety and a high transfection rate to allow for a sufficient level of expression of the therapeutic gene for the treatment of the disease. The plasmid vectors are superior to the viral system in terms of biosecurity, but are generally inferior in terms of efficiency of gene transfer [21]. Because there were deaths associated with the use of viral vectors, research has turned to the development of new systems of non-viral gene transfer [21]. Electroporation [22], sonoporation [23] use of lipoplexes [24], polyplexes [25], transfection by hydrodynamics [26] and nucleofection [27] are examples of non-viral gene transfer that are efficient and comparable to the viral systems. As currently available gene transfer systems permit the transfer of most genes with variable sizes, many diseases can be treated by gene therapy [21].
There have been few studies involving gene therapy and muscle injuries. The plasmid is the vector of choice due to ease of manipulation, and gene expression for only a short period of time, lower cost of production on a large scale and safer drug formulation [20]. Many modifications can be easily performed on plasmid vectors to enhance gene expression and ease gene transfer [20].
Most studies use growth factors to enhance angiogenesis and myogenesis. Piccioni et al. [28] have shown that the Shh gene (Sonic Hedgehog) was able to activate the expression of myogenic and angiogenic factors thus increasing the capacity of muscle regeneration after injury. The IGF-1 (insulin-like growth factor) gene has been used to treat muscle injuries. It was effective in regenerating muscle fibers and was able to attract bone marrow stem cells to the injury site and, thus, accelerate tissue repair [29, 30]. Arsic et al. [31] constructed an adenovirus expressing VEGF (vascular endothelial growth factor) and injected it five days after the injury; the treated group showed better and faster muscle regeneration [31]. Vectors and genes that allow faster and improved recovery of injured tissue are the objectives of gene therapy for the treatment of muscle injuries. Generally, the use of a vector expressing a factor is much cheaper than the injection of the protein itself. And the effect lasts longer.
Cell therapy involves the use of cells for treatment. In the case of muscle damage, the cells that have been used more frequently in preclinical trials are mesenchymal stem cells (MSC) and muscle-derived stem cells (MDSC).
MSCs are non-hematopoietic multipotent stem cells that adhere to culture plates [32]. MSCs have the ability to renew and differentiate into multiple lineages of connective tissue, including bone, fat, cartilage, tendon, muscle, and bone marrow stromal cells [32]. These cells were first described by Friedenstein who found that MSCs adhere to culture plates, resemble fibroblasts in vitro, and form colonies [33]. MSCs are present in all adult tissues and in the wall of fetal tissue vessels as part of the pericyte population [34]. In vivo, MSCs are identified by expressing CD146 and CD271, and within the adipose tissue as part as the CD34 positive population [35]. A very rich source of MSC is the adipose tissue and these cells are called ADSCs (adipose-derived stem cells). Peçanha et al. [36] showed that the ADSCs were able to accelerate muscle recovery in treated animals.
The MDSC are multipotent stem cells that were isolated from mouse skeletal muscle and are obtained through a series of steps of plating on collagen plates [37, 38]. The MDSC have a high expression of Sca-1, very low levels of viementin (a fibroblast marker), low levels of desmin and other markers of muscle differentiation [38]. Ota et al. [39] showed that injection of MDSCs four days after injury increased angiogenesis and reduced scar tissue. This group found high levels of VEGF one week after injection. In addition, MDSCs expressed high levels of antioxidant and GSH (glutathione) and superoxide dismutase, allowing greater survival of these cells after injection [40, 41].
Currently the major concern is to retain these living cells at the injury site so that they can have a prolonged effect; many researchers have turned their attention to this problem. Distefano et al. [42] used electrical stimulus and the cells remained longer at the site of injury. Park et al. [43] used losartan, an antihypertensive, and achieved a greater reduction in fibrosis following injury.
7.6 Conclusion
Treatment of muscle injuries depends on knowledge of the cause and nature of the injury. Treatment can be conservative or surgical. Gene and cell therapies are promising but much more research is need before we can proceed to a clinical protocol that is safe and efficient. The combination of the properties of stem cells with growth factors such as VEGF and IGF may, in the future, provide faster recovery from muscle injuries.
References
1.
Garrett Jr WE. Muscle strain injuries. Am J Sports Med. 1996;24(6 Suppl):S2–8.
2.
Mueller-Wohlfahrt HW, Haensel L, Mithoefer K, Ekstrand J, English B, McNally S, Orchard J, van Dijk CN, Kerkhoffs GM, Schamasch P, Blottner D, Swaerd L, Goedhart E, Ueblacker P. Terminology and classification of muscle injuries in sport: the Munich consensus statement. Br J Sports Med. 2013;47(6):342–50. doi:10.1136/bjsports-2012-091448 CrossRefPubMed
3.
Medvecky M. Skeletal muscle. In: Lieberman JR, editor. AAOS comprehensive orthopaedic review. Rosemont: AAOS; 2009. p. 83–91.
4.
5.
Bleakley CM, Glasgow P, Webb MJ. Cooling an acute muscle injury: can basic scientific theory translate into the clinical setting? Br J Sports Med. 2012;46(4):296–8. doi:10.1136/bjsm.2011.086116 CrossRefPubMed
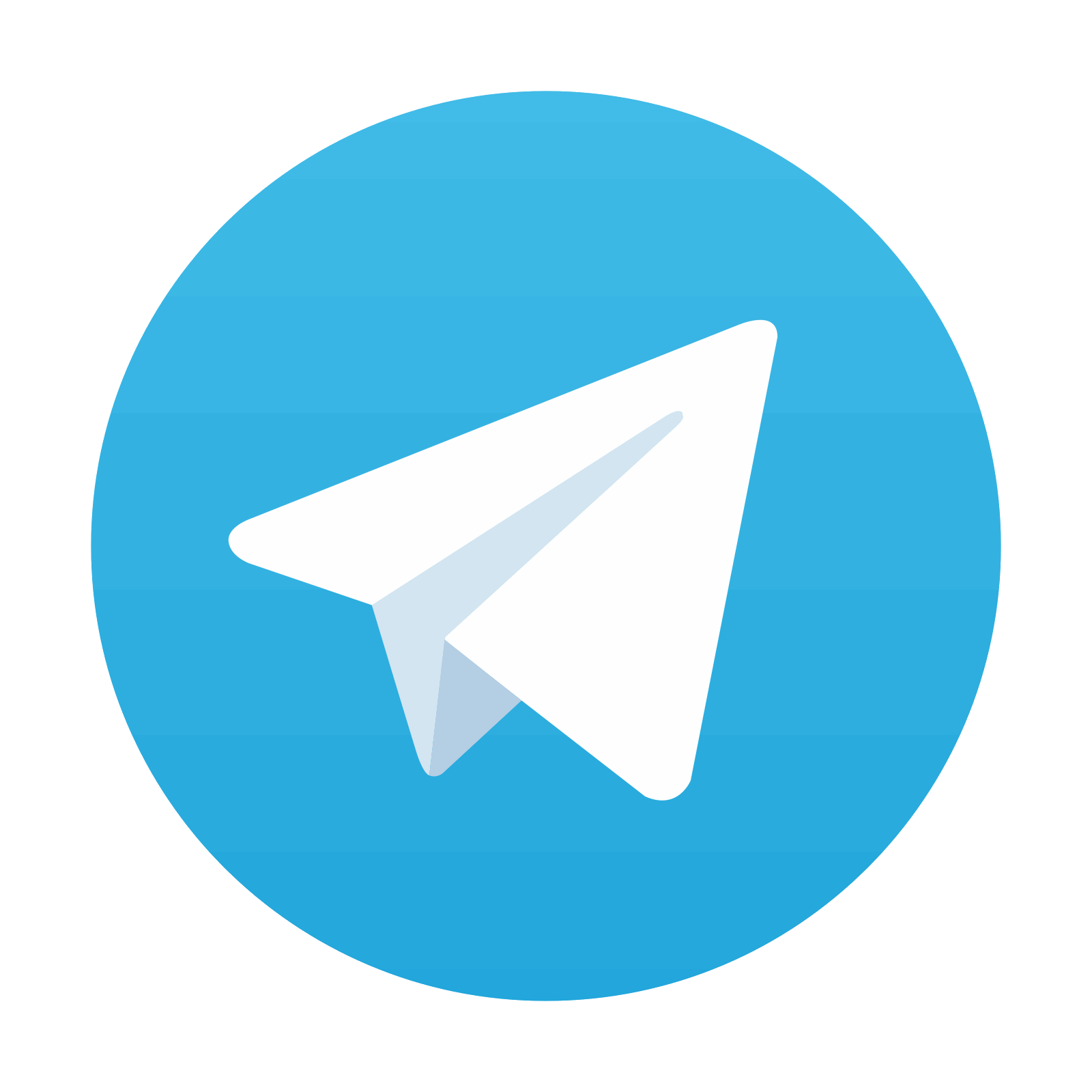
Stay updated, free articles. Join our Telegram channel

Full access? Get Clinical Tree
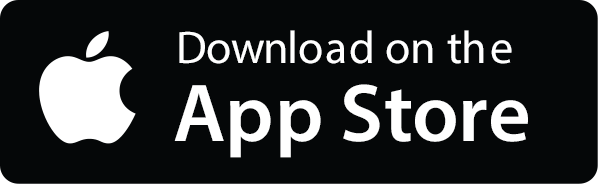
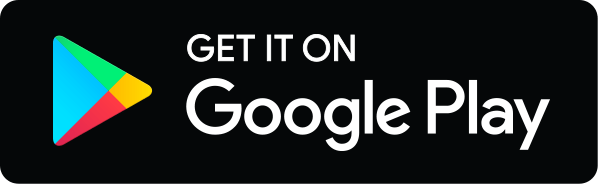