© Springer Japan 2016
Shin’ichi Takeda, Yuko Miyagoe-Suzuki and Madoka Mori-Yoshimura (eds.)Translational Research in Muscular Dystrophy10.1007/978-4-431-55678-7_77. Toward Regenerative Medicine for Muscular Dystrophies
Lessons from Regeneration Processes
(1)
Division for Therapies Against Intractable Diseases, Institute for Comprehensive Medical Science, Fujita Health University, Aichi, Japan
(2)
Laboratory of Molecular and Cellular Physiology, Graduate School of Pharmaceutical Sciences, Osaka University, 1-6 Yamada-oka, 565-0871 Suita, Osaka, Japan
Abstract
The US National Institutes of Health defines regenerative medicine as “the process of creating living, functional tissues to repair or replace tissue or organ function lost due to age, disease, damage, or congenital defects.” In many cases, physiological regenerative processes give us multiple clues for creating new therapeutic methodologies. In skeletal muscle fields, the first attempt at regenerative medicine was transplantation of myoblasts into Duchenne muscular (DMD) patients in the early 1990s, but the clinical trials were not successful. At that time, not much was known about the regenerative process in skeletal muscle. However, due to the efforts of scientists and progress in technologies, many important discoveries have emerged. One is the study of muscle stem cells, named satellite cells, and another is identification of muscle-resident mesenchymal progenitors. In this chapter, we focus on satellite cells and mesenchymal progenitors and discuss the newest information concerning them to realize regenerative medicine.
Keywords
Muscle satellite cellMuscle-resident mesenchymal progenitorsCell transplantation7.1 Introduction
Skeletal muscle has great regenerative ability, which depends on muscle stem cells, also known as muscle satellite cells, and skeletal muscle differentiation is one of the best models for the study of cellular differentiation. Establishment of myogenic cell lines, discovery of myogenic transcription factors, and studies of developmental myogenesis allow us to understand the outline of regenerative and myogenic differentiation processes. However, some fundamental issues remain to be elucidated. Among them, molecular regulation of satellite cells in both a steady homeostatic state and during regeneration is of great interest. In addition, in order to exploit the efficient regenerative potential of satellite cells, the contributions of non-myogenic cells are also considered essential. The representative type of non-myogenic cell is the hematopoietic cell, especially the macrophage, because a lack of macrophages results in a decreased number of proliferating myogenic cells and eventually leads to impaired regeneration with fibrosis [1]. In addition to macrophages, recent studies have shown the contribution of regulatory T cells to muscle regeneration [2]. Skeletal muscle-resident mesenchymal progenitors, which were originally identified as a source of both fibrosis and fat accumulation in muscle [3, 4], have attracted great attention for their vital roles in both undamaged muscle and muscle regeneration. Similarly, new factors in maintaining the homeostasis of skeletal muscle have been proposed recently, and a deeper understanding of them will lead to the development of new therapeutic approaches. We introduce satellite cell and mesenchymal progenitors on the basis of new findings that are closely related to the progression of muscular disorders, including muscular dystrophies, and the establishment of regenerative medicine for skeletal muscle.
7.2 Cellular Components of Skeletal Muscle
Well-recognized cellular components of skeletal muscle are myofibers, endothelial cells, nerve cells, hematopoietic cells, and satellite cells. The myofiber is multinuclear and the largest cell in the body, surrounded by the basal lamina and connected to bone via tendons. Except for myofibers, all other cells are mononuclear. When mononuclear cells derived from uninjured skeletal muscle are analyzed by FACS, endothelial cells account for 60–70 % of them. Endothelial cells are positive for both CD31 (PECAM-1) and Sca-1, but negative for CD45. Nerve cells innervate myofibers via neuromuscular junctions and are essential in skeletal muscle for locomotion. In uninjured muscle, only a few hematopoietic cells exist, but when muscles are damaged, abundant neutrophils appear and then infiltration by macrophages follows them [1]. Besides these myeloid lineage cells, the importance of lymphoid cells in the regeneration process is also reported. Recent studies showed that a special population of regulatory T (CD4+Foxp3+) cells controls muscle inflammation and promotes skeletal muscle repair [2].
The most indispensable type of mononuclear cell for muscle regeneration is the satellite cell, which is known as the physiological stem cell of skeletal muscle [5, 6]. Only satellite cells are found between the basal lamina and plasma membrane of myofibers [7]. Pax7, M-cadherin, calcitonin receptor, Syndecan4, HeyL, and Odz4/Ten-m4 are specifically expressed in satellite cells in skeletal muscle [8–14]. Besides satellite cells, many studies have reported other myogenic stems/progenitors, for example, bone marrow cells, side population (SP) cells, and muscle-resident interstitial cells, that can participate in generating myofibers [15–24]. However, their potentials for generating myofibers are limited. Therefore, they seem inadequate as cell sources for regenerative medicine. When Pax7-expressing cells (satellite cells) are conditionally depleted utilizing a CreERT2-DTA (diphtheria toxin) system, skeletal muscle regeneration is almost completely inhibited [25, 26]. Therefore, these two studies confirmed the essential roles of satellite cells during skeletal muscle regeneration. On the other hand, an early response in muscle hypertrophy does not depend on satellite cells in a compensatory hypertrophy model mice [27], although satellite cells had been thought to be required for muscle hypertrophy based on the “myonuclear domain theory.” This study implied that satellite cells do not contribute to an acute change of myofiber size. On the other hand, there is a decrease in myofiber size during the lifelong process known as sarcopenia, the age-related loss of muscle mass. Although the contribution of the loss of the satellite cell pool to the onset of sarcopenia remains to be elucidated, many investigations have demonstrated a decreased number of satellite cells and impaired muscle regeneration in aged animals [28, 29]. In order to reveal the mechanism of the decline in the satellite cell pool, we need to reveal the molecular mechanism for maintaining the satellite cell pool. Fortunately, recent studies are starting to uncover the mechanism, which is expected to lead to the development of an ideal cell source for regenerative medicine [30–32].
Several types of stem cells or progenitors in the interstitial space of muscle are reported. Among them, the mesenchymal progenitor was recently identified as the origin of fibrosis and adipogenesis [3, 4, 33]. Mesenchymal progenitors are positive for both PDGFRα and Sca-1 and negative for CD31, CD45, and satellite cell markers. In addition, they do not have myogenic potential; therefore, mesenchymal progenitors are a completely distinct population of satellite cells. Notably, although mesenchymal progenitors were originally identified as a cell contributing to a pathogenic condition, they likely promote successful regeneration as well [34]. The physiological roles of mesenchymal progenitors are largely unknown, but an elegant in vivo study implied that mesenchymal progenitors play potent roles in muscle repair [35]. Utilizing Tcf4-CreERT2 knock-in and DTA mice, Mathew et al. ablated Tcf4+ cells and found impaired muscle regeneration with premature satellite cell differentiation, depletion of the early pool of satellite cells, and smaller regenerated myofibers. The direct relationship between mesenchymal progenitors and Tcf4+ fibroblasts is not fully understood, but Tcf4+ fibroblasts express PDGFRα. Therefore, we can speculate that the roles of mesenchymal progenitors overlap with those of Tcf4+ fibroblasts in many aspects. In addition to their roles in the regenerative process, a supportive effect of mesenchymal progenitors on myofibers has been proposed, even in a steady condition [36]. Taken together, the mesenchymal progenitor, like the satellite cell, is becoming recognized as an essential type of cell in skeletal muscle. In order to utilize the full potential of muscle regeneration for the treatment of muscle disorders, we have to understand their roles in skeletal muscle biology. Below, we introduce recent research on both satellite cells and mesenchymal progenitors and discuss their functions in muscular dystrophies, sarcopenia, and cancer-induced loss of muscle mass (cancer cachexia).
7.2.1 Satellite Cells
There is no doubt that prior research on embryonic stem (ES) cells enabled the generation of iPS cells. If there were no established methodology for maintaining ES cells in an undifferentiated state, it would be impossible to generate iPS cells. In the same way, in order to expand satellite cells in vitro while maintaining their original ability, we have to elucidate the maintenance and self-renewal mechanisms of satellite cells in physiological events. In this part, we introduce a brief history of satellite cells and the key molecules for maintaining satellite cells.
7.2.1.1 Brief History of Satellite Cells
In 1961, Dr. Alexander Mauro first reported the existence of satellite cells in frog muscle [7]. Subsequently, satellite cells were discovered in mammalian skeletal muscle. The name is derived from its specific location. One side of a satellite cell is directly attached to a myofiber via cell-cell adhesion molecules such as M-cadherin, and the other side is attached to the basal lamina; therefore, satellite cells are located between the myofiber and basal lamina. Other types of cells are never found in this position. Before the satellite cell-expressing molecules were identified, electron microscopy was the only means to identify and observe satellite cells. However, identifying the proteins expressed in satellite cells and establishing methodologies (FACS analysis, single-myofiber culture, and so on) allow us to observe satellite cells more easily [11, 37–39].
A stem cell is defined as a cell having both differentiation and self-renewal potentials. In 2008, Sacco et al. transplanted a single satellite cell and demonstrated that the satellite cell has both potentials [6]. Although there are many reports indicating the existence of muscle stem cells besides satellite cells, as described above, many recent studies leave no doubt that the satellite cell is a physiological stem cell having both differentiation and self-renewal potentials [5, 25, 26, 40].
7.2.1.2 Maintenance Mechanism of Satellite Cells
Like other stem cells, satellite cells are maintained in an undifferentiated and quiescent state [41]. This state is considered essential for maintaining a satellite cell pool. In general, each tissue stem cell can be found in a specific location, called its niche, composed of certain types of cellular and extracellular matrix proteins. The satellite cell locates beneath the basal lamina and attaches to a myofiber; therefore, both of them together are thought to create the niche for satellite cells. In addition, Christov et al. reported that satellite cells exist in close proximity to endothelial cells [42]. Therefore, endothelial cells might also be a niche cell for maintaining satellite cells, like hematopoietic, neural, and spermatogonic stem cells [42–44].
Recent studies are starting to reveal the molecular mechanism for keeping satellite cells in an undifferentiated and quiescent state [31]. A report by Seale and colleagues was the first that showed a decreased number of satellite cells in genetically modified animals. They found expression of Pax7 in myogenic cells and discovered the loss of the satellite cell pool in Pax7 knockout mice [12]. Because Pax7 is specifically expressed in satellite cells in skeletal muscle, Pax7 has been the best molecule for identifying satellite cells in skeletal muscle. Importantly, the expression of Pax7 has been also confirmed in across species (human, rodent, avian). Although Lepper et al. had reported that the role of Pax7 in adult satellite cells was dispensable [45], two independent groups demonstrated that it was essential even in adult satellite cells [46, 47]. The discrepancy is likely due to the construct of Pax7-floxed mice [46].
What is the role of Pax7 in satellite cells? Taking the abovementioned studies together, Pax7 is involved in both the generation and maintenance of satellite cells. Originally Seale et al. proposed the specification of muscle satellite cells by Pax7 [48]. Relaix et al. showed the antiapoptotic roles of Pax7 in satellite cells [49]. In addition to those roles, McKinnell et al. indicated that Pax7 directly regulates Myf5 expression by association with the Wdr5-Ash2L-MLL2 histone methyltransferase complex that causes methylation of histone H3 lysine 4 [50]. The phenotype of Myf5-null mice [51] is much milder than that of Pax7-null mice; therefore, other targets of Pax7 might be also important for the specification and/or survival of satellite cells. Significantly, Pax7 was used for generating satellite cell-like cells from human iPS cells [52], demonstrating that Pax7 is one of the important discoveries in satellite cell biology that promotes the achievement of cell therapy.
Another molecule essential for regulating satellite cells in adult skeletal muscle is the Notch signaling pathway, which is an evolutionarily conserved intracellular signaling system. When Notch signaling is activated by its ligand (Dll1, Dll3, Dll4, Jag1, or Jag2), the intracellular domain of Notch is cleaved by a protease like γ-secretase and transported to the nucleus [53]. Then, the target genes are transcribed through interaction with Rbpj. Rbpj-dependent Notch signaling is known as canonical Notch signaling, and the representative target genes of canonical Notch signaling are the Hes (Hairy and enhancer of split) and Hesr (Hes related, also known as Hey/Herp/Hrt/Ghf/Gridlock) families of bHLH transcriptional repressor genes [54]. Using conditional ablation of Rbpj in Pax7-CreERT2 mice, two independent groups have indicated the essential roles of Rbpj for maintaining adult satellite cells in an undifferentiated and quiescent state [55, 56]. Intriguingly, when satellite cells in adult skeletal muscle lack Rbpj, they start to show precocious myogenic differentiation following protein expressions of MyoD and myogenin. They eventually fuse with myofibers and the satellite cell pool is diminished. When C2C12 cells or primary myoblasts are stimulated by Dll1 (Delta-like 1) or Dll4, Hesr1 and Hesr3 are upregulated [10, 57]. In addition, we observed premature differentiation and loss of satellite cell pools in Hesr1/3-double knockout mice [10]. Because each single knockout mouse did not show any significant defect in skeletal muscle including satellite cells, these results suggest that Hesr1 and Hesr3 have a redundant effect in maintaining satellite cells downstream of the canonical Notch signal [32]. Like Pax7, Notch signaling might contribute to the realization of cell therapy. Parker et al. compared the in vivo reconstitution potential of transplanted cells stimulated with Notch ligand and control cells and found that the former cells can engraft in vivo more actively than the latter [58]. The mechanism of Pax7 expression induction and the type of ligand/receptor for canonical Notch signaling in adult satellite cells have not been fully determined. Therefore, elucidation of these regulatory mechanisms will lead to the identification of the in vitro maintenance conditions for preparing cells for regenerative medicine.
Except for Pax7 and Notch-related genes knockout mice, decreased numbers of satellite cells are observed in some genetic knockout mice [59–63]. Most gene knockout mice show a loss of satellite cell pools at a relatively young age [31]. However, the phenotypes of Sprouty 1 (an inhibitor of receptor tyrosine kinase signaling) knockout mice are quite different. The loss of Sprouty 1 did not affect the number of satellite cells in uninjured muscle of young mice, but in aged mice (22 months), the satellite cell pool was diminished by half [64]. They also showed that this diminishment of satellite cells resulted from accelerated fibroblast growth factor (FGF) signaling. Therefore, maintenance genes can be divided to two categories: one is a gene for autonomous regulation, and the other is a gene affected by a change of environment. The elucidation of both mechanisms is important for the development of an ideal cell source for muscular disorders.
In addition to coding RNA, noncoding RNA is also essential for maintaining the satellite cell pool in uninjured muscle. Dicer is an enzyme for generating microRNA. When Dicer is conditionally and specifically deleted in adult satellite cells, they start to express proliferative markers and eventually undergo apoptosis [65]. Among quiescent stage-specific microRNA, Cheung et al. demonstrated that mir-489 is critical for a quiescent state of satellite cells through suppressing its target gene, Dek. Notably, mir-489 is located in the intron of the calcitonin receptor expressed specifically in quiescent satellite cells in skeletal muscle [9]. The expressions of both mir-489 and calcitonin receptor are downregulated after activation of satellite cells. During regeneration, re-expression of calcitonin receptors is restricted to the area where regeneration is mostly completed [9]. Although the physiological role of calcitonin receptor is unknown, the common expression mechanism of both mir-489 and calcitonin receptor might be extremely important to unravel the mechanism for establishment of quiescent satellite cells during developmental and regenerative processes.
7.2.1.3 Self-Renewal Mechanism of Satellite Cells
As described above, adult satellite cells are maintained in a dormant and undifferentiated state, but when skeletal muscle is injured, these cells start to enter an activated state. Before proliferating, they begin to express MyoD protein and then enter the cell cycle. Proliferating satellite cells that express both Pax7 and MyoD are called myoblasts. When they become more committed to myogenic differentiation, they start to express myogenin and lose Pax7. Myogenin-expressing cells that can fuse with each other or myotubes and make multinucleated myotubes are called myocytes. After myotubes mature, myofibers are innervated and rebuilt. During the regeneration processes, satellite cells produce self-renewed satellite cells that are indispensable due to their repeated regenerative potentials. The critical mechanism of satellite cell self-renewal is unknown, but two basic models have been proposed. Using cultured single myofibers, Zammit et al. indicated that almost all satellite cells become Pax7+MyoD+ cells, and then a part of them return to a Pax7+MyoD− state. On the other hand, Kuang et al. found that satellite cells can be divided into two populations based on YFP expression driven by Myf5-Cre and that the Pax7+Myf5−(YFP−) population has more stem cell-like characteristics than the Pax7+Myf5+(YFP+) population. Intriguingly, when Pax7+Myf5− cells divide on a plane, most cells generated are Pax7+Myf5− cells. On the other hand, when Pax7+Myf5− cells do apical-basal division, both Pax7+Myf5− and Pax7+Myf5+ cells (asymmetric division) are generated. However, these cells were observed in regenerating myotubes/myofibers having central nuclei. Furthermore, Wnt7s promote the number of Pax7+Myf5− cells [66], and the effect of Wnt7a was accelerated by fibronectin, which is specifically expressed during myotube/myofiber formation [67]. Therefore, there is a possibility that symmetric and asymmetric division in Pax7+Myf5− might occur after several cell divisions of Pax7+MyoD+ cells. The self-renewal mechanism of satellite cells is one of the important issues to be explained.
7.2.2 Mesenchymal Progenitors
In 2010, we identified muscle-resident mesenchymal progenitors [3]. Originally, mesenchymal progenitors were identified as cells contributing to pathological changes in skeletal muscle, but, at the same time, we speculated on their support functions in muscle repair because many mesenchymal progenitors proliferate actively even during a successful regeneration process. Intriguingly, recent studies revealed their support functions in muscle regeneration and homeostasis. In this part, we introduce several pathophysiological roles of mesenchymal progenitors on the basis of recent reports.
7.2.2.1 Mesenchymal Progenitors as Villains
Fibrosis is a hallmark of pathological conditions with impaired regeneration in many tissues. In skeletal muscle, accumulation of adipocytes is also observed in progressed muscular dystrophy patients. In order to identify the origin of the cell populations involved in fat accumulation and fibrosis in skeletal muscle, we performed comprehensive FACS-based cell analyses. Although satellite cells were considered the original source of fibrosis and adipogenesis, we found that a non-satellite cell population exclusively contributed to both fat accumulation and fibrosis in skeletal muscle, and this cell population specifically expressed PDGFRα [3, 4]. Besides the potentials to produce adipocytes and fibrogenic cells, they can also differentiate into osteogenic cells [3, 68]. Therefore, we called these cells mesenchymal progenitors.
Our study clearly demonstrated that only PDGFRα+ mesenchymal progenitors differentiate into adipocytes and form ectopic fat tissue in skeletal muscle [3]. This conflicts with the adipogenic potentials of satellite cells proposed by experiments using a myogenic cell line and single isolated myofiber. Myogenic cell lines are extremely useful for investigation of many aspects of myogenic differentiation, but they do not reflect some aspects of characteristics of in vivo satellite cells. Isolated single myofibers are also widely used for studies of satellite cell biology, but we have to pay attention to the risk of contamination by non-satellite cells (including mesenchymal progenitors) attached to the myofiber. Satellite cells were also proposed as a potent cellular source of collagen-producing cells by a study using a myogenic cell line [69]. In fact, fibrosis-related genes are detected even in highly purified primary myogenic cells, but their expression levels in mesenchymal progenitors are much higher than those of myoblasts, and myogenic cells do not contribute to or differentiate into collagen-producing cells in the dystrophic condition [4]. Brack et al. showed that satellite cells in aged mice transdifferentiate into fibroblastic cells in response to canonical Wnt signaling [28]. Therefore, we cannot exclude the potential of satellite cells to become fibroblastic cells. However, given their highly fibrogenic nature, it seems highly plausible that mesenchymal progenitors are the best candidate for fibrosis-initiating cells in skeletal muscle. In addition, we could identify a human counterpart to these progenitors by the expression of PDGFRα [34, 70]. Therefore, their pathological relevance to muscular disorders is further convinced. Taken together, the mesenchymal progenitor is the most potent origin of adipogenesis and fibrosis in skeletal muscle.
7.2.2.2 Mesenchymal Progenitors and Pericytes
Mesenchymal progenitors reside in the muscle interstitium, and thus, their location is also distinct from that of satellite cells. Mesenchymal progenitors are more frequently observed in the perimysium than in the endomysium, particularly in the perivascular space, but they are distinct from pericytes because they locate outside the vessel wall and basement membrane of capillaries [3]. The definition of a pericyte is based exclusively on its anatomic location, where it is bounded by a common basement membrane with endothelial cells [71, 72]; there is no specific pericyte marker. Mesenchymal progenitors account for about 5–10 % of whole mononucleated cells isolated from skeletal muscle [3], but skeletal muscle is known to have an extremely low percentage of pericytes. In skeletal muscle, there is only one pericyte per 100 endothelial cells [71, 73]. This is quite a contrast to pericyte-rich tissues such as the retina or central nervous system, where the pericyte to endothelial cell ratio is 1:1 [71, 73]. Because endothelial cells account for 60–70 % of total mononuclear cells in skeletal muscle, as described earlier, pericytes should constitute only 0.7 % of all mononuclear cells at most. Although pericytes have been reported to possess myogenic differentiative potential [74], mesenchymal progenitors cannot differentiate into myogenic cells. Where do mesenchymal progenitors arise developmentally? We do not know, but we are sure that they do not originate in bone marrow and that they reside in skeletal muscle before the beginning of inflammation.
7.2.2.3 Mesenchymal Progenitors and Fibroblasts
The morphology of mesenchymal progenitors is similar to that of fibroblasts, and there is no marker that distinguishes fibroblasts and mesenchymal progenitors. Unlike fibroblasts, mesenchymal progenitors have the potential to differentiate into mesenchymal lineage cells including adipocytes, chondrocytes, and osteoblasts. Therefore, the ability to differentiate is the only way to characterize mesenchymal progenitors. Sudo et al. showed that primary fibroblasts derived from various human tissues can differentiate into at least one mesenchymal lineage cell [75], and mesenchymal progenitors and so-called fibroblasts have much more similarity than previously recognized [76]. Therefore, many tissues seem to have their own tissue-resident mesenchymal progenitors which have been considered as fibroblasts.
7.3 Muscular Dystrophy
Duchenne muscular dystrophy (DMD) is a representative inherited muscular disorder that is caused by a mutation in the DYSTROPHIN genes [77]. Besides DMD, more than 30 different types and subtypes of muscular dystrophies are known, and most of them lack cell structure-related molecules for sustaining the cell membrane stability of myofibers. DMD patients exhibit progressive muscle weakness, and eventually myofibers are replaced by fibrosis and adipocytes. Although the mechanism of progressive muscle weakness is still unknown, it has been believed that a decline of the ability of muscle regenerative potential results in an increase in the size of pathogenic areas. Therefore, the loss of satellite cell numbers and function is considered to be one reason why many muscular dystrophies exhibit progressive symptoms. In this section, we introduce the relationship between satellite cells and the progression of muscular dystrophies. In addition, we discuss mesenchymal progenitors as therapeutic targets for muscular disorders.
7.3.1 Satellite Cells and Progression of Muscular Dystrophies
In 2010, we found impairment of the repeated regenerative ability in the DBA/2, but not C57BL/6, BALB/c, and C3H/HeN strains, and showed that mdx mice having a DBA/2 background exhibit much severe dystrophic phenotypes than C57BL/10-mdx, a conventional DMD model mice [78]. The proliferative ability of DBA/2-derived satellite cells is inferior to that of C57BL/6 satellite cells. This phenomenon is not limited to mdx mice. Heydemann also observed sever phenotypes in gamma sarcoglycan-deficient mice having a DBA/2 strain background compared to 129SV/J and C57BL/6 J strain mice [79]. The same group employed an unbiased mapping approach and identified a latent TGF-beta-binding protein 4 gene (Ltbp4) as one explanation of the phenotype of DBA/2 J background mice [80]. Ltbp is considered to be a negative factor for TGF-β activity; therefore, accelerated TGF-β signaling likely contributes to the pathological progression in DBA/2-J dystrophic mice. In fact, mutations of LTBP4 are found in humans and result in increased TGF-β activity in cultured human DMD patient-derived fibroblasts [81]. Until now, the phenotype of DAB/2 satellite cells and LTBP4 was unclear because both anti- and pro-proliferative effects of TGF-β signaling have been reported during proliferation of myogenic cells [82]. If we can determine the gene responsible for the low repeated regenerative potential in DBA/2 satellite cells, the progression of muscular dystrophies might be controlled.
Lamin A/C and emerin are known as causal genes of autosomal-Emery-Dreifuss muscular dystrophy (A-EDMD) [83] and X-EDMD [84], respectively. Notably, both molecules are expressed in quiescent satellite cells. In addition, our microarray analyses reveal that satellite cells express dystroglycan and dystrophin genes [9]. The roles of lamin A/C and emerin in satellite cells are still unknown, but some causative genes of muscular dystrophy may affect the function and survival of satellite cells directly, which might determine the severity of symptoms [85]. The relationship between the causative genes and satellite cell biology is one of the important issues in understanding each muscular dystrophy and development of therapeutic approaches.
7.3.2 Mesenchymal Progenitors as Therapeutic Target
Some studies have indicated or implied that PDGFRα+ mesenchymal progenitors are also positive for Sca-1, ADAM12, Tcf4, CD90, and Tie2 [3, 86, 87]. Among them, PDGFRα is not only a specific marker for mesenchymal progenitors but also can be a molecular target for many disorders including muscular dystrophy. Olson and Soriano generated constitutively active PDGFRα mutant mice and observed systemic fibrosis including skeletal muscle in these mice [88]. We showed that PDGF-AA (one ligand of PDGFRα) promotes cell proliferation and expression of fibrosis-related genes in PDGFRα+ mesenchymal progenitors [4]. Furthermore, we showed that a tryrosine kinase inhibitor, imatinib, inhibits the effect of PDGF-AA on PDGFRα+ mesenchymal progenitors, and we and others indicated that imatinib ameliorates dystrophic symptoms in the DMD model mice [89–91].
Besides imatinib, many small molecules ameliorate the dystrophic symptoms of mdx mice. Intriguingly, mesenchymal progenitors might be a direct target of some of them. Losartan is a blocker of the angiotensin II type I receptor and is widely used for treatment of hypertension. Cohn et al. showed that losartan improves skeletal muscle regeneration in mdx and fibrillin-null mice [92]. Fibrillin-1 is a structural component of extracellular matrix microfibrils that is known to be a negative regulator of TGF-β activation and signaling. The effect of losartan is attributed to reduced expression of thrombospondin-1 (TSP-1), which is a downstream target of angiotensin II and has the potential to activate latent TGF-β. In our analyses, mesenchymal progenitors also express TSP-1, and TGF-β induces the expression of fibrosis-related genes (Col1a1, Col3a1, a-SMA, CTNF) in mesenchymal progenitors. Therefore, mesenchymal progenitors might be a direct target of losartan in the dystrophic condition.
Histone deacetylase (HDAC) is a component of the epigenetic regulator. Trichostatin A (TSA) is a chemical compound that selectively inhibits mammalian classes I and II but not class III HDACs. Iezzi et al demonstrated that the expression of follistatin was upregulated in TSA-treated C2C12 cells compared with control C2C12 cells [93]. Follistatin inhibits myostatin, a well-known negative regulator of muscle mass, because myostatin-null mice, cows, and humans exhibit a noticeable increase in skeletal muscle mass. Follistatin was induced by TSA and then promoted C2C12 recruitment and fusion. The same group also showed that TSA induced follistatin in myotubes and ameliorated dystrophic symptoms of mdx mice in vivo [94]. Importantly, TSA enhances the ability of mesenchymal progenitors to promote myogenic activity of myoblasts in vitro and in vivo. Mesenchymal progenitors express a much higher level of follistatin than myogenic cells, and the positive effect of mesenchymal progenitors on myogenesis is mediated by follistatin [95]. Therefore, the beneficial effect of an HDAC inhibitor on muscular dystrophy is largely mediated by mesenchymal progenitors. Taken together, PDGFRα+ mesenchymal progenitors are or will be potent direct targets for the treatment of muscle diseases.
Muscle CD31−CD45− SP cells [24] are only a small portion of the cells present during regeneration, but they have characteristics similar to those of mesenchymal progenitors; therefore, we consider that muscle CD31−CD45− SP cells are a type of mesenchymal progenitor. In 2008, we showed that co-transplantation of satellite cells and muscle CD31−CD45− SP cells results in an increased number of satellite cell-derived myofibers in vivo [96]. Mozzetta et al. reported that co-transplantation of satellite cells with mesenchymal progenitors resulted in an increased number of donor-derived myofibers [95]; therefore, co-transplantation of mesenchymal progenitors might be a promising cell therapy to improve the results of satellite cell transplantation. However, when mesenchymal progenitors are transplanted into a severely dystrophic environment, they differentiate into adipocytes or collagen-producing cells. Therefore, one of the most important issues is the mechanism that controls the behavior of mesenchymal progenitors and affects the myogenic reconstitution of satellite cells. Regulation of the fate of mesenchymal progenitors will be a promising therapeutic strategy to treat muscle dystrophy.
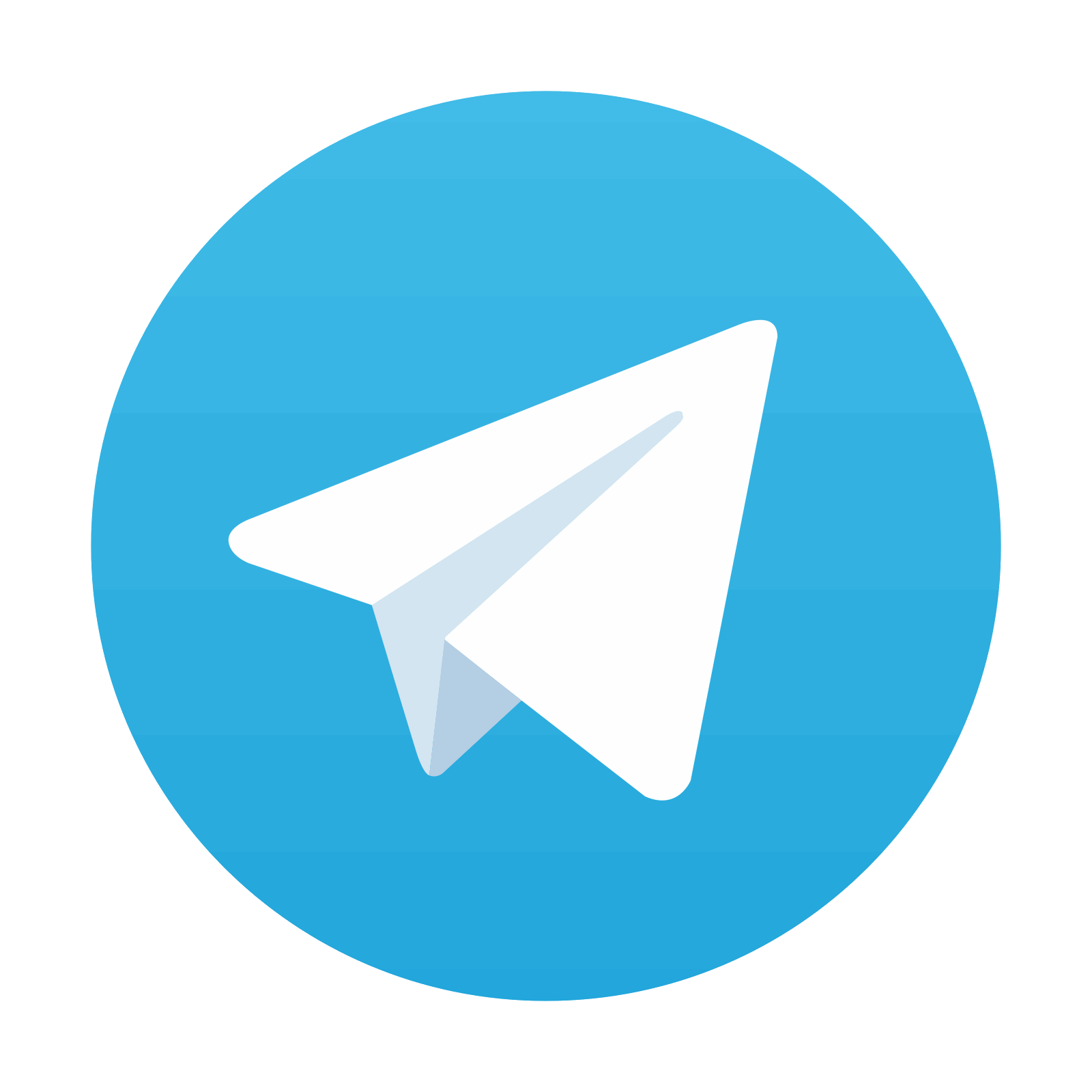
Stay updated, free articles. Join our Telegram channel

Full access? Get Clinical Tree
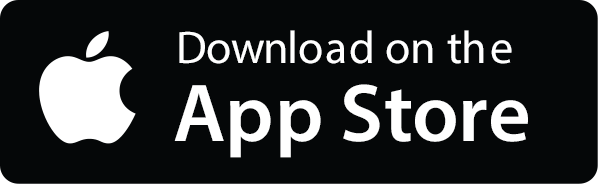
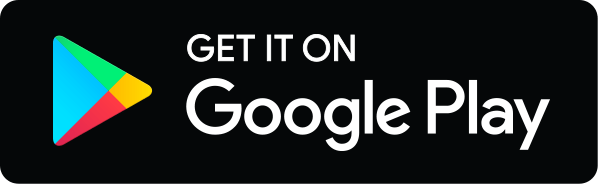