Fig. 8.1
Myoblast transplantation therapy (MMT) for DMD. Endogenous skeletal muscle satellite cells are located between the basal lamina and sarcolemma of myofibers in a dormant state. Upon muscle injury, they are activated, proliferate, migrate, and fuse with injured myofibers to regenerate myofibers. Wild-type myogenic cells injected into degenerating muscle fuse with host dystrophin-deficient myofibers in both animal models and clinical trials. Incorporated nuclei transcribe the normal dystrophin gene, and the hybrid myofibers formed by endogenous and grafted cells become dystrophin-positive and resistant to mechanical stress. When conventional protocols for cell transplantation are used, only a small number of injected satellite cells/myoblasts participate in muscle regeneration, and the engrafted cells are found to fuse only with myofibers near the injection site. The results suggest that the distance injected cells can migrate is limited. Of note, grafted myoblasts fuse with regenerating myofibers but not with undamaged myofibers. This may partly explain the low efficiency of MTT
8.3 Myoblast Transplantation Therapy (MTT)
8.3.1 Myoblast Transfer in Animal Models
More than 30 years ago, Partridge et al. first demonstrated that mouse myoblasts intramuscularly transplanted into recipient mice fuse with recipient myofibers [5]. In 1989, Partridge et al. reported that normal muscle precursor cells from wild-type mice intramuscularly transplanted into dystrophin-deficient mdx mice fused with preexisting mdx myofibers and formed dystrophin-positive myofibers [6]. Based on that report, preclinical and clinical trials of myoblast transplantation began.
8.3.2 MTT (1991–1997)
The studies on MTT for DMD conducted between 1991 and 1997 were disappointing [7–9]. Failure of the trials was ascribed mainly to poor survival and insufficient migration of the transplanted myoblasts. Experiments using animal models suggested that a significant fraction of grafted myoblasts die (or are lost) immediately after transplantation [10]. It was also pointed out that satellite cells had been exhausted by expansion in culture and lost their high regenerative potential [11]. However, some researchers argued that current culture protocols might simply be inappropriate for the expansion of satellite cells/myoblasts, because satellite cells in their niche expand robustly and regenerate injured muscle [12]. In fact, culture conditions mimicking in vivo environments might enhance the survival and proliferation of satellite cells/myoblasts after transplantation [13]. Satellite cells/myoblasts are supposed to migrate quite short distances from the site of injection. Therefore, to produce therapeutic effects, the cells need to be injected at multiple sites at quite small intervals.
8.3.3 High-Density Myoblast Transplantation (2004–2007)
It is widely accepted that satellite cells/myoblasts do not migrate long distances but fuse only with the myofibers in close contact with them. To overcome this limitation, Skuk et al. performed high-density multiple injection of myoblasts into muscles of a Duchenne muscular dystrophy patient at 1–2 mm or smaller intervals under immunosuppression by tacrolimus [14]. Their attempt successfully produced numerous dystrophin-positive fibers in a limited area. Interestingly, the same group has recently reported that transplanted myoblasts can migrate more than several millimeters in nonhuman primate skeletal muscle [15]. The migration of satellite cells might be improved under certain conditions.
8.3.4 Local Injection of Autologous Myoblasts for Oculopharyngeal Muscular Dystrophy (OPMD)
MMT is expected to restore muscle function in myopathies that affect specific muscles, such as oculopharyngeal muscular dystrophy (OPMD). A French group performed a Phase I/IIa clinical study of cell therapy for OPMD (clinicalTrials.gov NCT00773227). Twelve patients received cricopharyngeal myotomy and were injected with autologous myoblasts prepared from unaffected quadriceps or sternocleidomastoid muscles. The study verified the feasibility and safety of the procedures and, importantly, reported cell dose-dependent improvement in swallowing: the patients injected with more than 178 million myoblasts showed improvement in swallowing duration, but the patients transplanted with fewer than 178 million myoblasts did not [16]. The observation suggests that the number of transplanted cells for therapeutic effects has a minimum threshold.
8.3.5 Cell Source Other Than Satellite Cells/Myoblasts
Mesoangioblasts were originally identified as multipotent stem cells associated with the embryonic dorsal aorta [17]. In human skeletal muscle, a subset of pericytes possesses similar properties [18]. In contrast to satellite cells, mesoangioblasts were demonstrated to migrate from the blood into the degenerate muscle and to ameliorate disease phenotypes of two animal models of muscular dystrophy (mouse and dog) after intra-arterial delivery [19, 20]. Clinical trials (Phase I/II) of intra-arterial transplantation of HLA-identical allogeneic mesoangioblasts for DMD are now ongoing (EudraCT no. 2011-000176-33). The regime includes immunosuppression of the host.
8.4 Induced Pluripotent Stem Cells (iPS Cells)
Induced pluripotent stem cells (iPS cells), which show properties almost identical to embryonic stem (ES) cells, can be generated from a wide variety of somatic cells by overexpression of a set of reprogramming factors ([21–23]. Since Park et al. reported generation of iPS cells from DMD patients [24], iPS cells have been expected to be a potential cell source of autologous cell transplantation for DMD (Fig. 8.2).
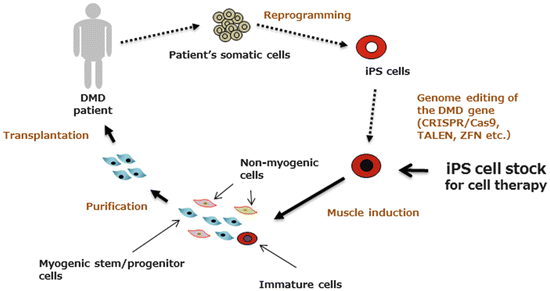
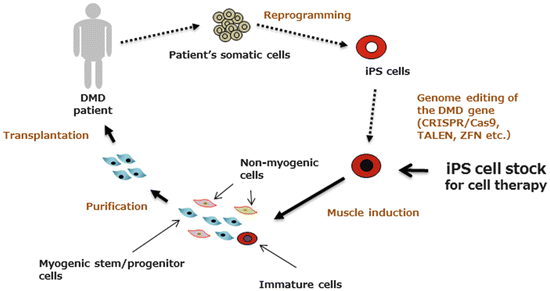
Fig. 8.2
Proposed cell therapy for DMD using patient-specific iPS cells (autologous cell transplantation). Fibroblasts or blood cells from DMD patients are converted into embryonic stem (ES) cell-like pluripotent stem cells by overexpression of reprogramming factors (originally SOX2, OCT3/4, KLF4, and c-MYC). After correction of gene mutations using genome-editing tools such as CRISPR/Cas9, TALEN, or ZFN, iPS cells are induced to differentiate into myogenic cells. Undifferentiated cells are eliminated to avoid tumor formation, and induced myogenic cells are transplanted back into the patient’s muscle, where grafted cells are expected to fuse with dystrophin-deficient myofibers and also migrate into the niche for satellite cells. To circumvent the long process of establishment and evaluation of iPS cells, Yamanaka’s team at Kyoto University is constructing a therapeutic iPS bank, a library of iPS cells with major HLA types. “Ready-made iPS cells” require only immunosuppression of the host
8.4.1 Advances in Methods of Skeletal Muscle Induction
Although it has long been believed that skeletal muscle cannot be easily induced from ES/iPS cells, several groups have recently reported successful derivation protocols.
8.4.1.1 Direct Reprogramming of Human iPS Cells into Skeletal Muscle by Forced Expression of MYOD
More than 25 years ago, MyoD was first isolated as a master gene of myogenesis because ectopically expressed MyoD induced differentiation of non-myogenic cells to skeletal muscle [25]. This was the first report of direct reprogramming by transcription factors. Rao et al. transduced human ES cells with a lentiviral vector encoding a doxycycline (DOX)-inducible MyoD. The protocol takes approximately 10 days to induce multinucleated myotubes in vitro with an induction efficiency of over 90 % [26]. Tanaka et al. constructed a PiggyBac transposon vector to overexpress MYOD in the presence of DOX [27]. After the cells are switched to muscle differentiation medium, MYOD starts to activate the genes involved in terminal differentiation such as contractile proteins and muscle-specific enzymes. This protocol is able to differentiate human iPS cells into multinucleated myotubes in a short period. In some iPS lines, however, the efficiency of muscle differentiation is not 100 %. It remains to be determined whether MyoD-induced cells can be safely applied to cell therapy of DMD.
8.4.1.2 PAX7-Induced Myogenic Progenitor Cells
PAX7 drives myogenesis together with PAX3 during development and plays unique roles in postnatal satellite cells (reviewed in [28]). Forced expression of PAX7 during embryoid body (EB) formation successfully induces transplantable myogenic cells from human ES cells [29]. Transplanted PAX7-induced myogenic cells efficiently fuse with host myofibers and restore dystrophin expression and muscle function in immunodeficient mdx mice. In contrast to the MRF family (MYOD, MYF5, MRF4, and myogenin), PAX7 alone does not induce fibroblasts to differentiate into skeletal muscle. Therefore, the differentiation stage of the cells and timing of the expression of PAX7 in human ES/iPS cells seem critical.
8.4.1.3 Sphere-Based Culture Method Induces Myogenic Stem/Progenitor Cells from ES/iPS Cells
Recently, Hosoyama et al. reported a new protocol named “EZ-sphere culture” for derivation of myogenic stem/progenitor cells from human ES/iPS cells [30]. iPS cells are cultured in low-attachment poly(2-hydroxyethyl methacrylate)-coated flasks and a medium generally used for formation of neurospheres but supplemented with high concentrations of human fibroblast growth factor 2 (FGF2) and human epidermal growth factor (EGF) (100 ng/ml each). In 1 week, human ES/iPS cells form free-floating spheres and robustly expand (Fig. 8.3). It takes roughly 6 weeks for a sphere to become myogenic. When plated onto MatrigelTM-coated dishes, cells forming myospheres start to fuse to form multinucleated myotubes and finally start to twitch. The method is applicable to most human ES and iPS clones including patient-derived iPS cells. We confirmed the myogenic potentials of a variety of disease-specific iPS cells, such as Ullrich congenital muscular dystrophy, Schwartz-Jampel syndrome, congenital myasthenic syndrome, and Duchenne muscular dystrophy (Miyagoe-Suzuki et al. unpublished data). Importantly, some iPS clones fail to form spheres, and some fail to differentiate into the skeletal muscle lineage. This might be correlated to incomplete reprogramming of the iPS cells, but further investigation is needed to determine the cause.
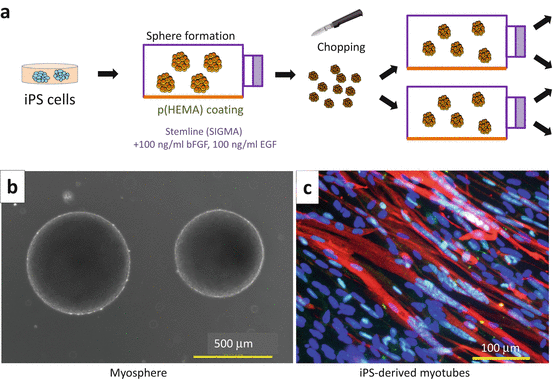
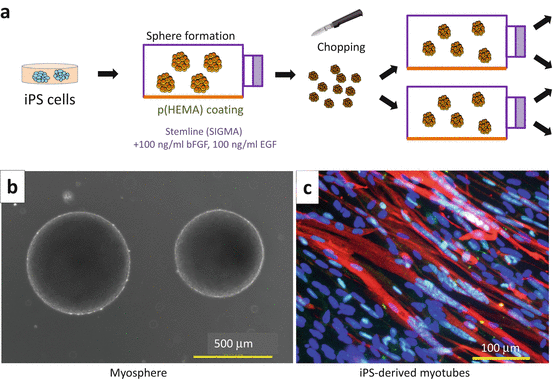
Fig. 8.3
Induction of skeletal muscle from human iPS cells by sphere-based culture (EZ-sphere method). (a) EZ-sphere method. iPS cells are cultured in StemlineTM medium (Sigma-Aldrich S-3194) supplemented with 100 ng/ml human FGF-2 and 100 ng/ml human EGF in p(HEMA)-coated flasks, where iPS cells form spherical aggregations and continue to grow. To keep the size of a sphere between 200 and 500 μm, spheres are cut weekly using a McIlwain tissue chopper. (b) Spheres are derived from human iPS cells. (c) Myosphere cells fuse and form MF20-positive (red) multinucleated myotubes on MatrigelTM-coated dishes in differentiation medium (DMEM containing 2 % horse serum and antibiotics). Nuclei (blue) are detected with Hoechst 33342
The percentage of myogenic cells produced in cultures using the original protocol described by Hosoyama et al. [30] is not 100 %, and they need purification before transplantation. To increase the efficiency of myogenic differentiation, clarification of the molecular mechanisms by which myogenic cells are induced through sphere formation is required. Myogenic cells induced by the EZ-sphere method fuse with cardiotoxin-injured muscle fibers in host NOD/Scid mice (Miyagoe-Suzuki et al. unpublished data).
8.4.2 Genome-Editing Techniques and Autologous Cell Transplantation
Although whether patient-derived iPS cells and their derivatives are immunogenic or not when transplanted into the same patient remains controversial (discussed in [31]), recent tools for genome editing, such as CRISPR/Cas9, TALE nucleases (TALENs), and zinc finger nuclease (ZFN), are encouraging in that they help us to prepare gene-corrected cells from patients for autologous cell transplantation in a relatively short period. For DMD, in addition to gene correction by homologous recombination of the mutated region, restoration of the reading frame by exon skipping at the genomic level or by inserting a small DNA fragment is another option to obtain autologous cells for therapy (reviewed in [32]).
8.4.3 Problems of iPS-Derived Muscle Stem/Progenitor Cells Remaining to Be Solved
8.4.3.1 Evaluation of Myogenic Potentials of Myogenic Cells Induced from Human iPS Cells in Animal Models
So far a limited number of research groups have reported successful engraftment of human iPS cell-derived myogenic cells in animal models. All studies used immunodeficient dystrophin-deficient mdx mice. Recently NSG–mdx 4Cv mice, which have triple mutations, i.e., NOD/Scid, IL2 receptor gamma chain deficiency, and a mutation in the DMD gene, have been successfully used for xenogeneic transplantation [33]. However, xenogeneic transplantation might underestimate the myogenic potency of human muscle stem/progenitor cells. On the other hand, in many studies, the leg muscles of recipient mice are X-irradiated to suppress endogenous satellite cells and injected with a high dose of cardiotoxin to damage the whole TA muscle and evoke synchronized muscle regeneration. X-irradiation and cardiotoxin injection are not physiological and cannot be applied in the clinical setting. Thus, currently, the efficiency of cell transplantation is being tested in conditions quite different from clinical settings. The results need to be interpreted with caution.
8.4.3.2 Heterogeneity of iPS Clones in Differentiation Potential
Human iPS cells are heterogeneous in myogenic differentiation potential. Differences are found even among iPS clones from the same donor. One possible reason for the heterogeneity is that some clones are incompletely reprogrammed and retain epigenetic memory of their original cell, which interferes with the differentiation into the intended lineages. Practically, it would be useful to find characteristic gene expression patterns or specific markers of highly myogenic iPS clones instead of empirically testing each iPS clone for myogenic potential.
8.4.3.3 Tumorigenicity of iPS Cell-Derived Myogenic Cells
Human iPS cells vigorously self-renew. Although this property makes them an attractive source for cell transplantation for devastating diseases, the same property seems to be related to tumorigenesis by transplanted undifferentiated cells. Importantly it is suggested that the risk of tumorigenesis differ greatly among iPS clones. Therefore, primarily, “good” iPS clones should be selected for clinical application. How can we know the quality of iPS cells before transplantation? Koyanagi-Aoi et al. reported the gene expression and methylation patterns of “bad” human iPS clones, which retain a significant number of undifferentiated cells after neural differentiation culture and form teratomas when transplanted into mouse brains [34]. Such a molecular signature would facilitate the selection of therapeutic cells.
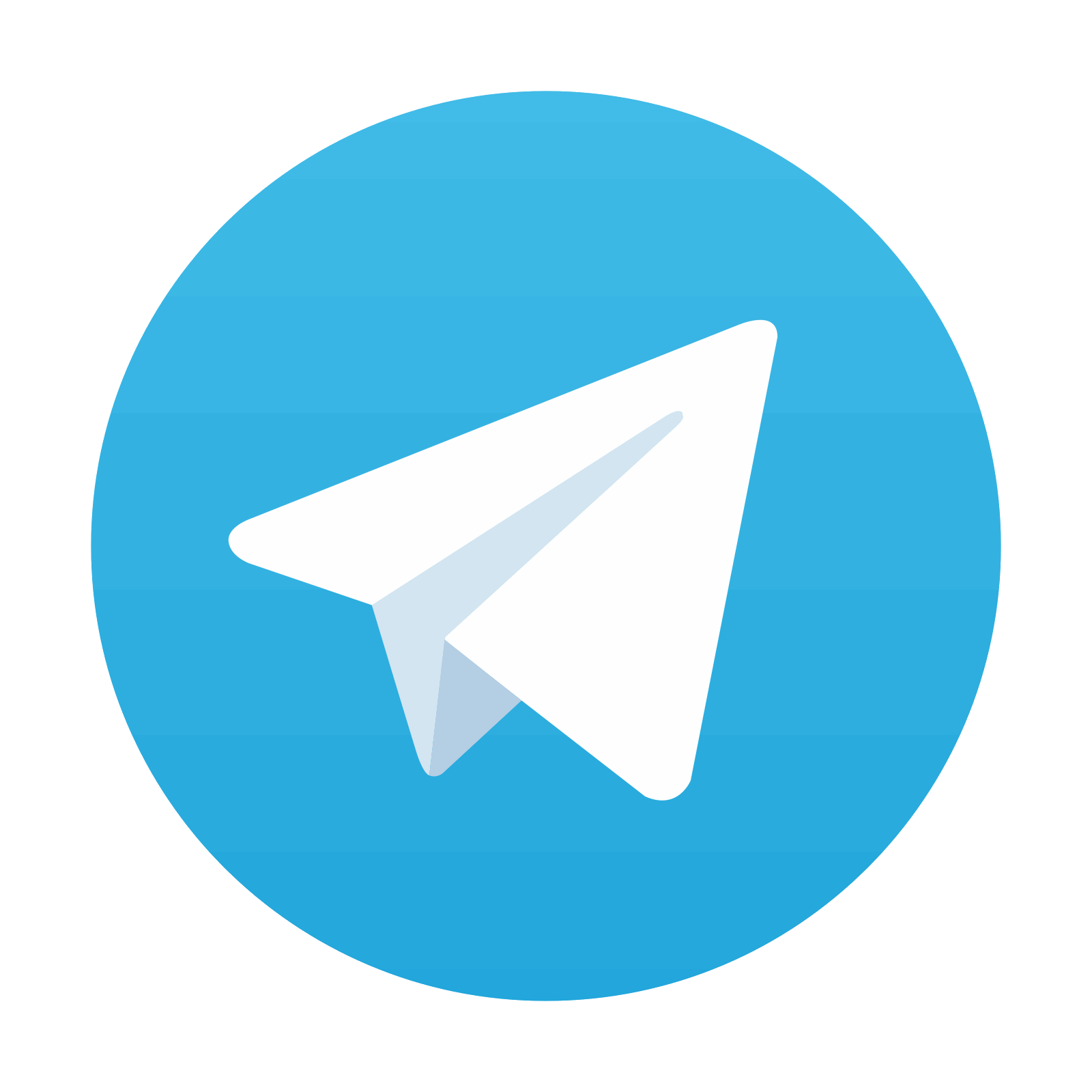
Stay updated, free articles. Join our Telegram channel

Full access? Get Clinical Tree
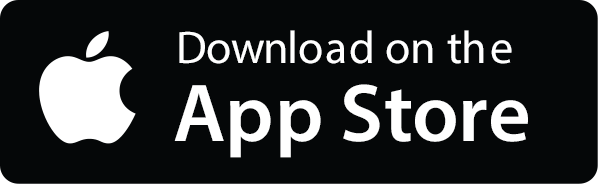
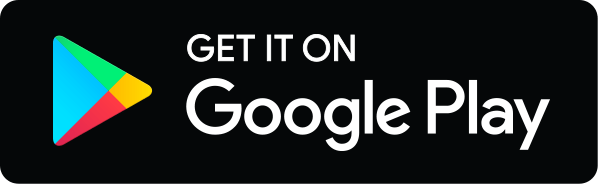