, Francois Lintz2, Cesar de Cesar Netto3, Alexej Barg4, Arne Burssens5 and Scott Ellis6
Keywords
ImagingWeight bearing CTSyndesmotic injuryDeltoid ligament injuryIntroduction
Accurate identification of distal tibiofibular syndesmosis injuries is difficult, especially in the absence of frank bony diastasis [1–4]. Literature suggests that missed injuries may lead to chronic pain and early degeneration of tibiotalar articulation [5, 6]. Currently, the initial diagnosis of syndesmotic disruption is based off several radiographic parameters [2]. Measurement of the medial clear space (MCS), tibiofibular clear space (TFCS), and the tibiofibular overlap (TFO) on external stress ankle radiography has high specificity yet low sensitivity [2, 7]. Magnetic resonance imaging (MRI) has also been shown to aid in diagnosis; however, this non-weight bearing modality fails to illustrate the dynamic relationship between the tibia and fibula when load and torque are applied to the ankle [2, 8–10].
The anatomical relationship within the distal tibiofibular syndesmosis is highly complex and relies on four main ligaments to maintain congruity: the anterior-inferior tibiofibular ligament (AITFL), interosseous membrane (IOM), posterior inferior tibiofibular ligament (PITFL), and transverse tibiofibular ligament (TTFL) [11]. Additionally, medial ankle support via the deltoid ligament is thought to play a pivotal role [12]. The entire complex is subject to acute and chronic structural changes from repeated stresses seen during daily ambulation or secondary to acute injury [13]. An appropriate understanding of how these structures interact and react under stress is essential to our understanding of pathology.
First described in 1998, cone beam computed tomography (CT) utilization has steadily increased since its introduction in the orthopedic literature in 2013 [14, 15]. Weight bearing CT offers a precise representation of bony architecture under physiologic loads as well as the resultant change in biomechanics incurred during injury [16]. The purpose of this cadaver study was to assess whether weight bearing CT scans can be used to identify subtle and also more severe injuries to the distal tibiofibular syndesmosis using three commonly used measurement options.
Methods
Data Source
Ten different male cadavers (tibia plateau to toe-tip; seven left, three right) were included (mean age 63 ± 9 [range 47–70] years; mean weight 83.1 ± 13.5 [range 63.5–104.8] kg; mean BMI 26.3 ± 3.8 [range 20.1–32.3] kg/m2). The cadavers have been provided by United Tissue Network (Norman, OK, USA), and Science Care (Phoenix, AZ, USA). Inclusion criteria were 20–70 years of age and a body mass index (BMI) of less than 35 kg/m2. To ensure a homogeneous cohort, only male cadavers were included. Exclusion criteria were a history of any foot and ankle injuries or a history of surgery of the foot and ankle.
Experiments

Experimental setting. (a) Frame used to hold the cadaver in a plantigrade position. An Ilizarov apparatus was used for fixation of the tibia. (b) The frame fits into the weight bearing CT scanner. Load and torque can be applied following dissection of the distal tibiofibular syndesmosis [1]
Intact ankles (native) were scanned first. Then, each ankle underwent AITFL transection (Condition 1). The deltoid ligament was transected next (Condition 2), followed by the IOM (Condition 3), and the PITFL (Condition 4). Transection of the deltoid ligament included the tibio-navicular and tibio-spring ligament. Then, the tibiocalcaneal ligament was transected. Finally, the anterior and posterior parts of the deep deltoid ligament were transected under visual control. While Conditions 1 and 2 mimic incomplete injuries, Conditions 3 and 4 mimic more complete injuries to the distal tibiofibular syndesmosis.
Weight bearing (85 kg; determined from the average specimen weight) CT scans with and without application of 10 Newton meter (Nm) internal rotation torque applied at the Ilizarov apparatus (corresponding to external torque of the foot and ankle) were performed (PedCAT, CurveBeam LLC, Warrington, USA, medium view, 0.3 mm slice thickness, 0.3 mm slice interval, kVp 120, mAs 22.62) [13]. Before the experiments were performed, preconditioning of the specimens was done by loading the frame with 42.5 kg and 85 kg for 2 minutes each.
Imaging and Measurements

Measurements performed and digitally reconstructed radiographs (DRRs) and single computed tomography (CT) images. (a) Anteroposterior (AP) view showing the medial clear space (MCS), tibiofibular clear space (TFCS), and tibiofibular overlap (TFO). (b) Mortise view of the same cadaver showing symmetrical widening of the ankle mortise. (c) Single CT image 1 cm above of the ankle joint showing the TFCS and TFO. (d) Single CT image 1 cm below of the ankle joint showing the MCS measured at the most anterior part of the joint between the medial malleolus und the talus [1]
CT scans 1 cm above the medial edge of the distal tibial plafond (axial images) were reconstructed at the highest point of the distal tibial plafond on the sagittal view and used for measurements of the TFO and the TFCS (Fig. 14.2) [24]. CT scans 1 cm below the medial edge of the distal tibial plafond (axial images) were additionally reconstructed. Those images were used for measurement of the MCS (Fig. 14.2). The MCS was defined as the distance between the most anterior articular surface of the medial malleolus to the talus.
Statistical Analysis
Intraclass correlation coefficients (ICC) were used to quantify the agreement of measurements between and within observers. Estimates and 95% confidence intervals (CI) were calculated for each type of measurement within each view. Interobserver agreement was modeled with a two-way random effect model of absolute agreement with a single measurement per observation, defined as ICC. Intraobserver agreement was modeled with a two-way mixed effect model of consistency with a single measurement per observation, defined as ICC. Agreement was rated as very good (ICC > 0.80), good (ICC = 0.61–0.80), moderate (ICC = 0.41–0.60), fair (ICC = 0.21–0.40), and poor (ICC < 0.20) [25]. Measurements for interobserver agreement calculation were done by a physician and a research analyst. For calculation of the intraobserver agreement, measurements were performed two times with an interval of 3 weeks by a physician.
Linear mixed effect models were fit for responses. Within DRR and CT measurements, separate models were fit for each measurement (MCS, TFO, and TFCS) and, for DRR measurements, within each view. Cadaver, a random effect, and foot (left or right), a fixed effect, were included in all models in addition to the variables presented. Models were fit for subsets of the data and estimates, and 95% CI were given for differences in measurements in different levels of a specific variable. For each of the models, only the differences in response that were associated with either different torques or different conditions were calculated; the data was subset by the other two variables, and they remained constant within each model. The first set of models compared the differences in response for 10 Nm versus 0 Nm of torque applied with full weight bearing load (condition constant within each model). The second set of models compare the differences in response between Conditions 1 through 4 and the native ankles, with full weight bearing load (torque constant within each model). Coefficients and 95% confidence intervals were reported, and statistical significance (marked by an asterisk in all tables and graphs) was determined based on a P-value of less than 0.05.
Pearson’s correlation was used to assess the relationship between DRR—AP view only—and CT scan. Correlation was graded as very strong (>0.80), strong (0.60–0.80), moderate (0.40–0.60), weak (0.20–0.40), and very weak (<0.20). For each measurement, values were paired by cadaver, foot, condition, and torque, and the correlation estimated between the pairs was computed. Confidence intervals were constructed using the Fisher transformation. All calculations were done in R 3.4.1, specifically using packages psych and lmerTest.
Results
Digitally Reconstructed Radiographs
Reliability of digitally reconstructed radiographs (DRR) and computed tomography (CT) measurements assessed by intraclass correlation coefficient (ICC) [1]
Interobserver: ICC(2,1) Estimate (95% CI) | Intraobserver: ICC(3,1) Estimate (95% CI) | ||
---|---|---|---|
AP (DRR) | MCS | 0.75 | 0.93 |
(0.51, 0.87) | (0.87, 0.96) | ||
TFCS | 0.59∗ | 0.88 | |
(0.35, 0.76) | (0.79, 0.94) | ||
TFO | 0.95 | 0.99 | |
(0.88, 0.98) | (0.97, 0.99) | ||
Mortise (DRR) | MCS | 0.87 | 0.95 |
(0.77, 0.93) | (0.91, 0.98) | ||
TFCS | 0.55∗ | 0.70 | |
(0.29, 0.73) | (0.50, 0.83) | ||
TFO | 0.92 | 0.97 | |
(0.77, 0.97) | (0.94, 0.98) | ||
CT scan | MCS | 0.94 | 0.95 |
(0.89, 0.97) | (0.92, 0.98) | ||
TFCS | 0.93 | 0.94 | |
(0.74, 0.97) | (0.89, 0.97) | ||
TFO | 0.92 | 0.99 | |
(0.53, 0.98) | (0.97, 0.99) |
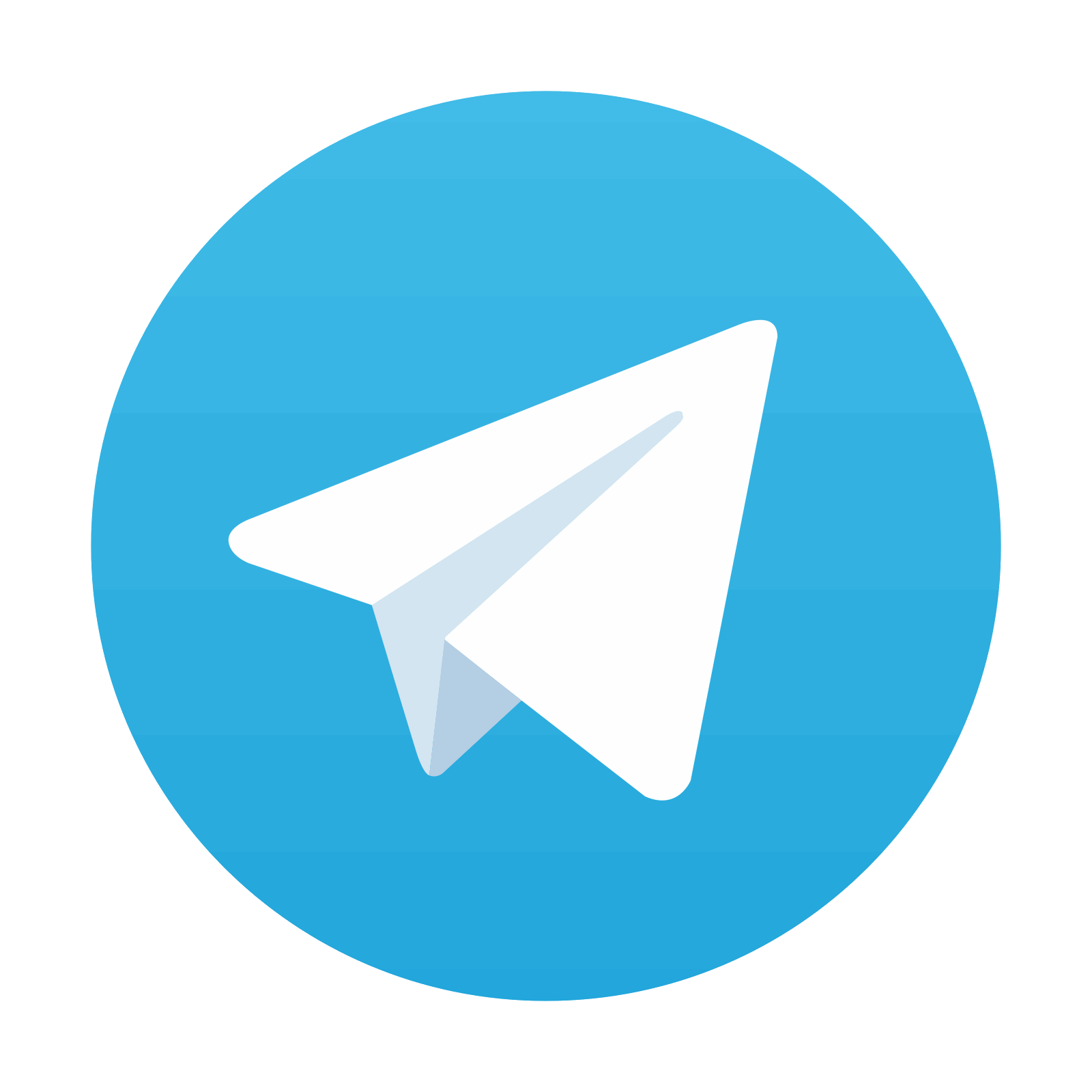
Stay updated, free articles. Join our Telegram channel

Full access? Get Clinical Tree
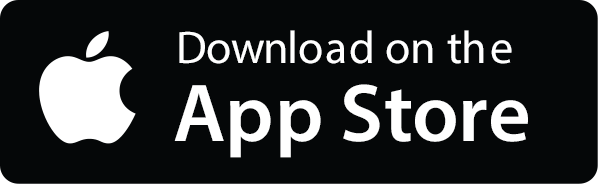
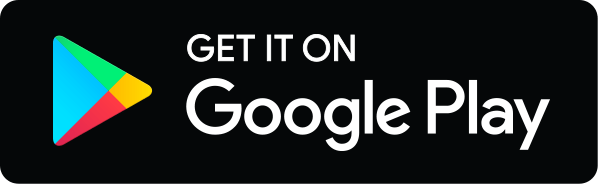