Thermal Treatment, Sutures, Knots, and Bone Anchors
Robert A. Pedowitz MD, PhD

Arthroscopic methods for shoulder surgery offer superior visualization and open the door for complex procedures with minimal surgical morbidity, but technological advances do not necessarily guarantee better clinical results.
The selection of a device for supplying heat to the shoulder should be driven by safety, ease, and cost-effectiveness. Depth of penetration of heat can affect risk of injury to adjacent neurologic structures and probably also plays a role in the ultimate healing and remodeling of the tissue.
The outcome of thermal modification surgery depends upon tissue remodeling. The biological response requires either initial fibroblast viability or repopulation with viable cells and in either case, tissue vascularity is essential for ultimate biomechanical durability.
Destruction of the capsule by thermal treatment can be extremely difficult to treat, and this condition may require tissue transfer or grafting procedures in order to reconstruct the deficient capsule.
Axillary nerve injury is an infrequent but important complication that has been associated with thermal capsular shrinkage. The axillary nerve is vulnerable due to its proximity to the capsule in the axillary recess, and heat application should be well-controlled in this region to minimize the risk.
The rotator cuff must be stabilized with suture material that is strong enough and lasts long enough to provide mechanical stability until adequate tissue healing is achieved.
At a minimum, the arthroscopic surgeon must master one sliding knot and one non-sliding knot. Much of the security of arthroscopic knots is achieved by placement of at least three reversed throws on reversed posts to back up the initial knot.
Bio-absorbable anchors remain hard for many months or even years after surgery, making concerns about anchor migration paramount regardless of anchor material. Magnetic reasonance imaging (MRI) can be useful for radiolucent anchor localization and may help with surgical planning if a broken anchor is suspected.
Recent decades have seen tremendous advances in surgical options for shoulder disorders. Arthroscopic methods for shoulder stabilization, labral repair, biceps tendon management, and rotator cuff treatment offer superior visualization and open the door for complex procedures with minimal surgical morbidity; however, technological advances do not necessarily guarantee better clinical results. The purpose of this chapter is to review some of the recent information regarding fixation and stabilization technology for shoulder arthroscopy. This section will present basic biomechanical information that may be used by the arthroscopic surgeon for clinical application in the burgeoning field of minimally invasive shoulder surgery.

Thermal capsulorrhaphy went through a phase of rapid clinical adoption based upon early reports of clinical success, but subsequent clinical experience led to a marked decrease in clinical enthusiasm. This early excitement was fueled, in
part, by frustration with existing surgical techniques and clinical results, the obvious ease and speed of arthroscopic application of thermal methods, and perhaps by premature commercial hype for general application of the technique. This initial enthusiasm, however, was followed by an equally rapid period of surgeon rejection, as reports of clinical failures and significant complications began to surface. At the moment, it is not clear whether thermal tissue modification will retain a specific niche in our orthopaedic armamentarium. Therefore, it makes sense to review some of the relevant basic science information regarding thermal tissue treatment.
part, by frustration with existing surgical techniques and clinical results, the obvious ease and speed of arthroscopic application of thermal methods, and perhaps by premature commercial hype for general application of the technique. This initial enthusiasm, however, was followed by an equally rapid period of surgeon rejection, as reports of clinical failures and significant complications began to surface. At the moment, it is not clear whether thermal tissue modification will retain a specific niche in our orthopaedic armamentarium. Therefore, it makes sense to review some of the relevant basic science information regarding thermal tissue treatment.

Heat can be applied in various ways to the shoulder joint, including the brutal though perhaps effective “hot poker” technique described many centuries ago. Current methods are more refined (we think); however, the basic mechanisms of thermal shrinkage are much more dependent upon the magnitude and speed of tissue temperature change than they are upon the method of inducing that change. Lasers devices and monopolar and bipolar radiofrequency probes are available for clinical application, but selection of a specific method should be driven by the safety, ease, and cost-effectiveness of the device in question. For example, the depth of penetration of heat can affect risk of injury to adjacent neurologic structures, and probably also plays a role in the ultimate healing and remodeling of the tissue because full-thickness tissue necrosis may obliterate the opportunity for revascularization and a fibroblastic response. Ciccone et al. (1) noted that human cadaveric glenohumeral capsular thickness varied from an average of 2.4 mm anteriorly to 2.8 mm in the inferior capsular pouch and to 2.2 mm posteriorly. Global shoulder capsule thickness ranged from 1.3 to 4.5 mm. Thinning of the capsule occurred from the labrum to the humerus, with a mean capsular thickness of 3.0 mm at the glenoid and 2.2 mm at the humeral insertion, and capsular thickness ranged from 2.8 to 3.2 mm in the regions in closest proximity to the axillary nerve.
Arnoczky and Aksan (2) and Gerber and Warner (3) have presented excellent reviews of the important basic science issues pertaining to tissue thermal modification. When collagenous tissue is heated, cross-links are broken and protein denaturation leads to a random, gel-like state. Upon cooling, the tissue shrinks along an axis parallel to the dominant direction of fiber orientation. Tissue shortening increases with increased temperature and time exposure to increased temperature; however, greater tissue heating is also associated with a greater loss of tissue stiffness. At tissue temperatures that are sufficient to induce collagen shortening, all cellular elements at the zone of thermal application are killed, with varying depth of necrosis based upon the magnitude and degree of thermal penetration. After thermal treatment, there is a phase of tissue fibroplasia; however, there are no accepted opinions regarding the time frame for equilibration of mechanical properties or the ultimate degree of mechanical strength that is recovered by the treated tissues. It is also not clear whether the initial degree of shrinking has a major bearing on the long-term biologic and biomechanical status of the capsule. Associated changes in the afferent neurologic pathways may affect shoulder function after thermal treatment.
Naseef et al. (4) noted that bovine knee capsular shortening was both temperature and time dependent. No shrinkage occurred until temperature reached at least 60°C, and above that temperature, heat initiated a phase transition in the collagen triple helix leading to collagen fibril denaturation and gel formation. At or above 65°C, there was complete loss of the native fine fibrillar collagen structure and complete loss of birefringence. The loss of birefringence signifies unwinding of the collagen triple helix with loss of collagen fiber organization. The maximal observed shrinkage was about 50% of the original tissue length. Hayashi et al. (5) studied human cadaveric shoulder capsule and also found that shrinkage occurred at temperatures of 65°C and above. Shrinkage was time-dependent. Hyalinization of collagen and ultrastructural changes were observed when the tissue was subjected to temperatures of 65°C or greater (6) (Fig 20-1).
Wall et al. (7) examined the early mechanical properties of bovine tendon as a function of tissue shrinkage. They found that with greater thermal shrinkage, a higher proportion of collagen fibrils underwent a conformational change from an extended, crystalline, inextendible state to that of a contracted, random coil, extendible state. Baseline collagen packing and fiber orientation probably play a role in the amount of shrinkage that is possible. Of importance, greater tendon shrinkage was associated with a more extendible structure upon subsequent testing, and after about 15% to 20% shrinkage, extendibility may be so great that the tissue may stretch beyond its original length.
Vangsness et al. (8) found that collagen shortening in cadaveric human tendons started at a temperature just below
70°C, but at temperatures greater than 80°C, the collagen physically fell apart. Histology revealed that the typical collagen striations and crimp patterns were not present in the heated tissue. Ten percent shortening of the patellar tendon was associated with a 70% loss of tensile load; however, these experiments did not address potential recovery of mechanical properties after tissue healing. In a separate study, Selecky et al. (9) used laser thermal treatment to induce 10% shortening of cadaveric glenohumeral ligaments. Early mechanical evaluation demonstrated higher ultimate strain and yield strain in the lased ligaments compared to control specimens, but no significant difference in ultimate stress, yield stress, or elastic modulus.
70°C, but at temperatures greater than 80°C, the collagen physically fell apart. Histology revealed that the typical collagen striations and crimp patterns were not present in the heated tissue. Ten percent shortening of the patellar tendon was associated with a 70% loss of tensile load; however, these experiments did not address potential recovery of mechanical properties after tissue healing. In a separate study, Selecky et al. (9) used laser thermal treatment to induce 10% shortening of cadaveric glenohumeral ligaments. Early mechanical evaluation demonstrated higher ultimate strain and yield strain in the lased ligaments compared to control specimens, but no significant difference in ultimate stress, yield stress, or elastic modulus.
Several groups have examined acute changes in glenohumeral joint mechanics following thermal capsulloraphy, and it is clear that an acuter change in joint translation for a given load can be achieved by thermal treatment (10,11,12,13,14,15,16,17). It is less clear that these changes are maintained after a relevant period of biological remodeling and subsequent high-demand activity. Tibone et al. (16) found that thermal shrinkage of cadaveric anterior capsuloligamentous structures reduced anterior translation by 41% and 43% with 15 N and 20 N applied loads, respectively. Shrinkage reduced posterior translation of the shoulder by 35% and 36% with 15 N and 20 N loads, respectively.
Victoroff et al. (17) applied pancapsular radiofrequency heat to human cadaveric shoulders and noted a 37% reduction of joint volume. This amount of shrinkage was associated with significantly reduced anterior, posterior, and inferior translation at 45 degrees and 90 degrees of abduction with an applied 30 N load, with some effect of humeral rotation upon observed translation changes. Luke et al. (12) compared thermal shrinkage to standard open capsulorrhaphy, and found 30% and 50% decreases in joint volume, respectively. Karas et al. (11) found that intracapsular volume was reduced 19% by four capsulolabral tucks, compared to 33% by “paintbrush” thermal shrinkage and 41% reduction by a combination of both methods. The authors note that these acute volume reductions in a cadaveric model do not necessarily correlate with ultimate volume alterations after surgery and rehabilitation in patients.
Selecky and Tibone (14) demonstrated that thermal treatment of the rotator interval alone can significantly decrease both anterior and posterior translation with the arm abducted 30 degrees and in neutral rotation. The same author (13), however, found that arthroscopic thermal capsuloplasty of the posterior capsule alone did not significantly decrease posterior glenohumeral translation, suggesting that thermal treatment is probably not a reliable method of treatment for posterior shoulder instability.
The outcome of thermal modification surgery depends upon tissue remodeling after the thermal insult. This biological response requires either initial fibroblast viability or repopulation with viable cells and in either case, tissue vascularity is essential for ultimate biomechanical durability. Schaefer et al. (18) examined rabbit patellar tendon after laser-induced thermal shrinkage. There was a significant positive correlation between the depth of laser penetration and the percentage of tissue shrinkage. After 4 weeks, the laser treated tendon was significantly longer than the immediate post-laser length. After 8 weeks, the laser treated tendons were significantly longer than both the post-laser and the baseline, pre-laser length. At this time point, the tendons were less stiff, had an increase in cross sectional area and demonstrated fibroblastic responses that were all significantly different than the bilateral control tendon. Based upon these data, the authors recommended immobilization for a period of time following thermal shrinkage in order to prevent the collagen from “stretching out.”
Schulz et al. (19) studied rabbit knee capsule up to 12 weeks after laser thermal treatment. They found decreased biomechanical properties immediately after treatment with recovery that was close to normal 12 weeks after treatment. Ultimate load, ultimate stiffness, and ultimate stress were all significantly decreased at 0 and 6 weeks post treatment but recovered by 12 weeks after thermal modification. Based upon these data, the authors recommended activity precautions particularly for the first 12 weeks after thermal tissue modification; however, these experiments were performed in rabbit knee capsule, and the pace and magnitude of biomechanical recovery may not extrapolate directly to human shoulder treatment. Lu et al. (20) compared a grid pattern (stripes of treatment separated by untouched tissue) to a paintbrush pattern (treatment of the entire surface area) to induce shrinkage in sheep knee joint capsule using a monopolar radiofrequency device, and followed the response up to 12 weeks after surgery. Although initial tissue shrinkage was similar, the grid pattern was associated with faster tissue healing and improved biomechanical recovery. These differences may be due to better preservation of cellular viability with the grid method, with associated improvement in biologic remodeling.
It is unclear from a review of the literature how much load can be tolerated by thermally modified tissue in the immediate postoperative period, and how that load changes during the healing phase. These basic questions are critical for establishment of postoperative rehabilitation protocols. Lack of optimal postoperative activity control (however that is defined), may explain some of our disappointing clinical results.
Clinical Results
Abrams (21) presented a thorough review of the important concerns related to clinical application of shoulder thermal capsulorraphy. Major issues include inconsistent tissue shrinkage, capsular ablation, over constraint, and neurologic complications. Anderson et al. (22) reported a 14% failure rate after thermal shoulder stabilization, with failure noted on average of 6 months after surgery. Failure was associated with multiple dislocations and prior surgery, with a tendency (inadequate statistical power) for decreased results with multidirectional instability and contact sports.
Early reports of thermal capsulorrhaphy tended to describe encouraging results. Lyons et al. (23) described a 12% unsatisfactory rate in 27 shoulders treated with laser shrinkage for multidirectional instability with a minimum follow-up of 2 years. Fitzgerald et al. (24) followed 30 of 33 consecutive multidirectional instability patients treated with radiofrequency thermal capsulorrhaphy in a military setting, with an average follow-up of 36 months. There were 3 excellent, 20 good, and 7 poor results, and 76% returned to active duty.
More recent reports tend to be more pessimistic about the clinical outcomes of thermal shrinkage. D’Alessandro et al. (25) followed 84 shoulders after thermal capsulorrhaphy performed with a monopolar radiofrequency device; most surgeries were done with the capsular “painting” technique. With an average follow-up of 38 months, the overall success rate was 63%, and the most favorable outcomes were seen in the patients with a traumatic dislocation. The worst outcomes were in patients with multidirectional instability with only a 55% success rate. Of note, these patients did well for the first year after which the clinical results declined. The most common complication was axillary nerve dysaethesias, seen in 14% of the patients. Patients who underwent open revision had a success rate of only 50% with the secondary procedure. In contrast, Savoie and Field (26) reported satisfactory results in 28 of 30 multidirectional instability patients treated with thermal capsulorrhaphy and adjunctive interval closure followed for an average of 26 months.
Levy et al. (27) noted a 36.1% failure rate at a mean follow-up of 40 months after laser capsular shrinkage, compared to a 23.7% failure rate at a mean follow-up of 23 months after shrinkage with a radiofrequency device. Noonan et al. (28) followed 59 patients after laser thermal treatment (some patients also had a suture anchor repair of a labral lesion); they observed a 4% failure rate after treatment of unidirectional instability compared to a 39% failure rate with multidirectional laxity. This report is concerning because unidirectional laxity is the best condition for treatment with current suture anchor and plication methods, whereas multidirectional laxity continues to be associated with the least predictable outcomes. In a very discouraging report, Miniaci and McBirnie (29) describe clinical failure in 9/19 instability patients treated by thermal techniques, with stiffness observed in 5 patients and neurologic symptoms noted in 4 patients.
Overhead athletes may be a specific subset of patients who may benefit from limited capsular shrinkage. Dugas and Andrews (30) reported on 170 overhead athletes treated by standard shoulder arthroscopy and debridement with addition of capsular shrinkage and a well-defined postoperative rehabilitation protocol. They noted that 81% returned to competition, and felt that, in their hands, addition of thermal capsular shrinkage resulted in about a 20% improvement in the rate of return to play. Follow-up averaged 30 months in this study. In a prior report by the same authors (31), however, heat shrinkage was not felt to improve the rate of return to play in baseball players, although the authors thought that treatment of internal impingement with thermal capsulorrhaphy might decrease the re-injury rate after return to sports.
Another clinical situation that may be reasonable for application of thermal tissue modification involves augmentation in cases of labral repair, such as SLAP repair or Bankart repair (32,33,34) utilized thermal capsular treatment as an adjunct to suture anchor stabilization in 42 patients with recurrent traumatic anterior instability. Recurrent instability occurred in 3 patients (7%). Although these authors report that thermal treatment was an important adjunct, it should be emphasized that comparable results are achievable with suture anchor placation methods alone. The selection criteria for adjunctive thermal treatment are not well-defined, and use of this approach as during suture anchor stabilization is at best left to the subjective judgment of the surgeon at the time of arthroscopic evaluation of capsular laxity.
Complications
Failure of treatment is typically thought of as recurrent instability, with a subset of patients requiring a subsequent stabilization procedure. Destruction of the capsule by thermal treatment can be extremely difficult to treat, and this condition may require tissue transfer or grafting procedures to reconstruct the deficient capsule (35,36). McFarland et al. (37) did not observe a specific histologic feature that could explain capsular deficiency in 7 patients who underwent biopsy after failed thermal treatment. Denuded synovium was observed in all of these patients, and 71% had changes in the collagen including “hyalinization.”
However, postoperative stiffness can also occur, and this complication may require additional surgical intervention as well (36). Gerber and Warner (3) believe that the major complication of thermal capsulorrhaphy is postoperative stiffness, with an incidence between 1% and 10% in their review.
Axillary nerve injury is an infrequent but important complication that has been associated with thermal capsular shrinkage (3,38,36). The axillary nerve is vulnerable due to its proximity to the capsule in the axillary recess, and heat application should be well-controlled in this region to minimize the risk of neurologic injury (39,40). McCarty et al. (41) noted a significant increase in temperature along the course of the axillary nerve in 11 of 15 cadaveric specimens during thermal application to the capsule; the branch to the teres minor appeared to be most vulnerable during this procedure. In a clinical follow-up study, however, shoulder proprioception returned to baseline when evaluated 6 months to 24 months after thermal treatment in twenty patients (42). These results suggest that normal neurologic function can be anticipated unless there is a catastrophic injury along the axillary nerve’s ramifications.

The overall strength of a repair construct, either arthroscopic or open, is defined by the “weakest link” in the chain. In the case of rotator cuff repair with suture anchors, the weak link may be the bone, anchor, eyelet, suture, knot, or rotator cuff tissue itself (Table 20-1). A variety of suture materials is available for clinical application; some are resorbable, others permanent, some are monofilament and others braided. Endoscopic knot tying is technically demanding and has a relatively steep learning curve (43,44), and the surgeon should select the appropriate knot for a given situation based upon the suture to be used, the mechanical requirements of the repair in question, and the surgeon’s experience and personal preference (45). Suture materials have unique handling characteristics and knot tying behavior (46), which the surgeon must understand to create the strongest possible repair. In addition, super high strength sutures have been introduced recently and these materials have been very helpful for minimizing suture breakage during surgery. Extraordinarily high tensile load to failure does not, in and of itself, guarantee secure surgical knots (Table 20-1).
TABLE 20-1 Variables That Affect Construct Stability, Healing, and Recovery After Rotator Cuff Repair | ||||||||||||||||||||||||||
---|---|---|---|---|---|---|---|---|---|---|---|---|---|---|---|---|---|---|---|---|---|---|---|---|---|---|
|
|
There are multiple basic science and clinical reports of suture anchor fixation for shoulder instability (47,48,49,50,51,52,53,54,55,56,57,58,59,60,61), and detailed clinical outcomes for these procedures will be discussed elsewhere in this book. The mechanical aspects of suture anchor stabilization tend to be more challenging with rotator cuff repair than with capsulolabral repair, due to obvious anatomic and age-related differences in bone and soft tissue quality. Although this section will focus upon issues specific to sutures and knots for arthroscopic rotator cuff repair, the principles are relevant to other surgical procedures as well.
Suture Materials
Rotator cuff repair sutures can be conveniently separated into monofilament or braided sutures and absorbable or nonabsorbable sutures (Table 20-2). An advantage of monofilament suture is the relative ease of tissue passing, particularly with devices that utilize hollow needles, because the inherent stiffness of the suture facilitates “pushing” or “wheeling” the suture within the tool; however, that stiffness comes with a price in terms of knot tying, because knots don’t lay
down easily with monofilaments, and they have a greater tendency for knot slippage (62). In contrast, braided sutures tend to be quite pliable and are generally relatively strong compared to similar size monofilaments. The introduction of antegrade suture passing tools has made management of braided sutures a bit more convenient (these instruments grab the suture on a needle prior to tendon puncture).
down easily with monofilaments, and they have a greater tendency for knot slippage (62). In contrast, braided sutures tend to be quite pliable and are generally relatively strong compared to similar size monofilaments. The introduction of antegrade suture passing tools has made management of braided sutures a bit more convenient (these instruments grab the suture on a needle prior to tendon puncture).
Absorbable sutures are advantageous in the long term because they minimize residual knots that can cause mechanical impingement/irritation in the subacromial space. However, mechanical integrity must be maintained at least until the strength of the healing response is sufficient to withstand physiologic loads. Rapid hydrolysis can lead to an inflammatory response, which in some cases can be confused with an early postoperative infection. In the case of a rotator cuff repair, it is unlikely that the bone-tendon interface is particularly strong before 6 weeks, and it probably takes 12 weeks or more for the bone tendon junction to partially mature. Bourne et al. (63) noted significant mechanical degradation between 2 and 6 weeks after in-vivo placement with various resorbable suture materials (Table 20-2). Similar findings were noted by Greenwald et al. (64), with the important observation the PDS suture was only about 10% to 15% as strong after 6 weeks compared to time zero in-vivo. Boileau et al. (65) recently reported a 71% healing rate with arthroscopic cuff repair using predominantly PDS sutures, suggesting that sufficient early mechanical integrity was maintained for cuff healing using this resorbable suture construct. In another recent study, Boehm et al. (66) found no significant difference in clinical outcome or re-tear rate (about 20%) after cuff repair with either Ethibond or PDS suture. At this time, there is no clearly optimal suture material for rotator cuff repair. The basic principle, however, holds that we must stabilize the cuff with suture material that is strong enough and lasts long enough to provide mechanical stability until adequate tissue healing is achieved.
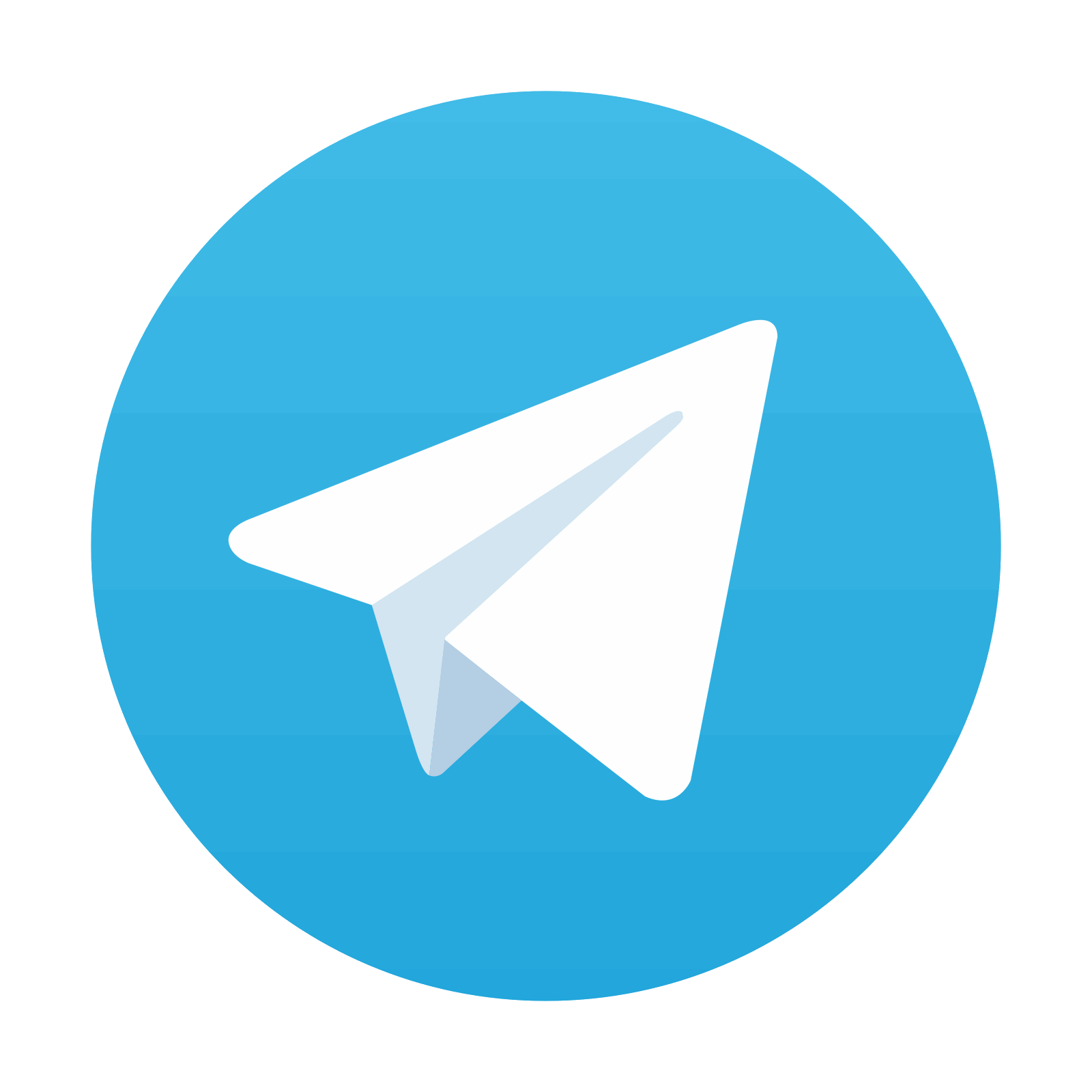
Stay updated, free articles. Join our Telegram channel

Full access? Get Clinical Tree
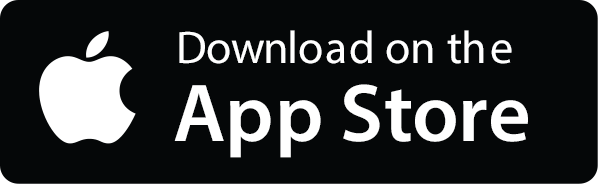
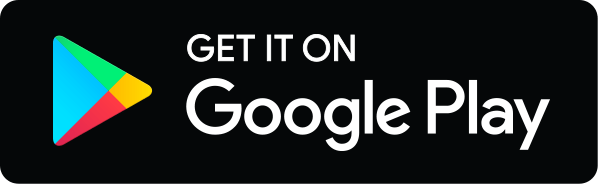