This article introduces the utility of electromyography (EMG) and mechanomyography (MMG) for the assessment of neuromuscular function, and discusses the interpretation of the EMG and MMG signals for various exercise perturbations. The results of these studies suggest that the use of EMG and MMG to determine muscle fatigue is robust. Future studies with clinical populations are needed, however, to determine the optimal use of EMG and/or MMG for assessing muscle function in rehabilitative settings.
Electromyography
Electromyography (EMG) has been used to study muscle function for decades. During voluntary contraction, action potentials are sent from the brain to the motor unit, ultimately leading to the contraction of muscle fibers. EMG, therefore, allows investigators to measure muscle activation. EMG can be divided into invasive and noninvasive methods. For invasive methods, fine-wire or needle EMG is used, with recording wires directly inserted into the muscle, whereas with noninvasive EMG, electrodes are placed on the skin over the muscle of interest ( Fig. 1 ). This latter approach is the focus of the first half of the paper. The second half of the paper introduces a complementary method of examining muscle function called mechanomyography (MMG). MMG measures the sound produced by activated muscle, and thus represents the mechanical counterpart to EMG. Therefore, this article introduces and discusses two noninvasive methods of examining muscle function that may be potentially useful in clinical settings ( Fig. 2 ).
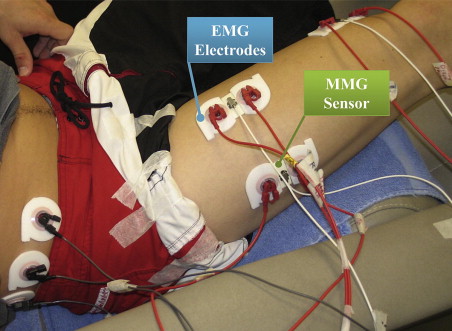
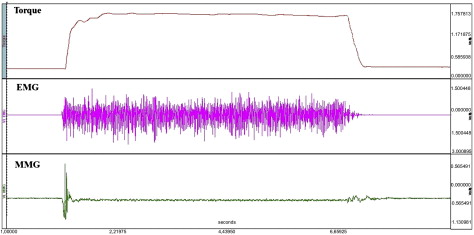
The EMG signal derived from muscle contraction is characterized into two domains called time and frequency . The time domain of the EMG signal is a measure of EMG amplitude (voltage) and reflects the number of motor units activated and the frequency of activation. The frequency domain of the EMG signal is a measure of how frequently the waveform crosses the baseline and is measured in hertz (Hz). It is related to the conduction velocity of the action potentials, at least for isometric muscle actions. The EMG time and frequency domains have been examined under various perturbations, such as isometric, isokinetic, eccentric, and dynamic muscle actions.
EMG responses to isometric and dynamic submaximal to maximal muscle actions
A variety of muscle action types (concentric, eccentric, and isometric) are typical of activities of daily living. Methodologies such as EMG suggest that the nervous system uses unique strategies to control muscle force production during each of these different types of muscle actions. This concept may have important implications for clinicians who are responsible for assessing muscle function and conducting strength training programs designed to assist with movement tasks that require the use of different muscle actions.
Isometric muscle actions are typically characterized by linear or curvilinear increases in EMG amplitude with force caused by concurrent increases in motor unit recruitment and firing rates to 50% to 80% of maximal voluntary contraction (MVC), and then increases in firing rates only between 50% to 80% and 100% of MVC. Investigators have also suggested that a curvilinear EMG amplitude versus isometric torque relationship may be caused by increases in the firing rates of motor units with fused twitches. Theoretically, increases in the firing rates of these motor units (with fused twitches) could cause an increase in EMG amplitude without a corresponding increase in torque production. This response would result in curvilinear EMG amplitude versus isometric torque relationships.
During isometric muscle actions, the frequency content of the EMG signal is believed to reflect the average conduction velocity of the action potential. This response is related to the number and type of the activated motor units. Theoretically, the progressive recruitment of fast-twitch motor units, with higher conduction velocities of action potentials, would lead to increases in EMG frequency with increases in torque muscle, although this is a debated concept.
For concentric muscle actions, the amplitude of the EMG signal increases linearly with increases in force. The limited number of studies that have examined eccentric muscle actions have found that they involve unique motor control strategies compared with isometric or concentric muscle actions. For example, muscle activation is lower during eccentric than concentric or isometric muscle actions, and preferential recruitment of fast-twitch motor units may occur during eccentric, as opposed to concentric, muscle actions.
EMG responses to isometric and dynamic submaximal to maximal muscle actions
A variety of muscle action types (concentric, eccentric, and isometric) are typical of activities of daily living. Methodologies such as EMG suggest that the nervous system uses unique strategies to control muscle force production during each of these different types of muscle actions. This concept may have important implications for clinicians who are responsible for assessing muscle function and conducting strength training programs designed to assist with movement tasks that require the use of different muscle actions.
Isometric muscle actions are typically characterized by linear or curvilinear increases in EMG amplitude with force caused by concurrent increases in motor unit recruitment and firing rates to 50% to 80% of maximal voluntary contraction (MVC), and then increases in firing rates only between 50% to 80% and 100% of MVC. Investigators have also suggested that a curvilinear EMG amplitude versus isometric torque relationship may be caused by increases in the firing rates of motor units with fused twitches. Theoretically, increases in the firing rates of these motor units (with fused twitches) could cause an increase in EMG amplitude without a corresponding increase in torque production. This response would result in curvilinear EMG amplitude versus isometric torque relationships.
During isometric muscle actions, the frequency content of the EMG signal is believed to reflect the average conduction velocity of the action potential. This response is related to the number and type of the activated motor units. Theoretically, the progressive recruitment of fast-twitch motor units, with higher conduction velocities of action potentials, would lead to increases in EMG frequency with increases in torque muscle, although this is a debated concept.
For concentric muscle actions, the amplitude of the EMG signal increases linearly with increases in force. The limited number of studies that have examined eccentric muscle actions have found that they involve unique motor control strategies compared with isometric or concentric muscle actions. For example, muscle activation is lower during eccentric than concentric or isometric muscle actions, and preferential recruitment of fast-twitch motor units may occur during eccentric, as opposed to concentric, muscle actions.
Using EMG to identity fatigue threshold
EMG Amplitude
Although several methods are available to identify fatigue threshold using cardiorespiratory indices, deVries and colleagues introduced the use of EMG to identify neuromuscular fatigue using cycle ergometry ( Fig. 3 ). The fatigue threshold or physical working capacity (PWC) uses mathematical modeling and physiologic indices such as the EMG amplitude or frequency to identify a workload that an individual can theoretically perform indefinitely. Briefly, the EMG fatigue threshold is identified from a series of visits during which the subject performs constant power output exercise on a cycle ergometer. The power outputs are selected to elicit a continuous increase in muscle activity during the ride. The duration of each ride, therefore, may be until voluntary exhaustion occurs or for a set period, such as 8 minutes ( Fig. 4 ). In their original work, deVries and colleagues focused on EMG amplitude, and therefore plotted EMG amplitude versus time for each power output. Thereafter, the investigators used linear regression to determine the slope of the relationship between EMG amplitude and time. Subsequently, the slope coefficients were plotted for each power output and linear regression was performed. The intersection between the regression line and the y- intercept is identified as the fatigue threshold for that individual, and is theoretically the maximal workload that the individual could continue indefinitely.
Recently, Camic and colleagues proposed a method of determining EMG fatigue threshold from a single incremental cycle ergometry test. Briefly, the investigators recorded EMG amplitude from the vastus lateralis at each workload. Thereafter, linear regression was performed to determine if the slope of the regression line was significantly different from zero. The investigators then selected the highest workload that had a nonsignificant ( P >.05) slope and the first workload that had a significant ( P <.05) slope. These two workloads were then averaged to provide an estimate of the EMG fatigue threshold from a single incremental test to voluntary exhaustion. This new approach may have applications in a clinical setting if future studies validate this method and show that it is sensitive for detecting changes in the muscle with various interventions, such as endurance training.
EMG Frequency
Several studies have examined measures of EMG frequency during various fatiguing exercise tasks, including those performed by clinical populations. Typically, these studies have found that fatigue is associated with decreases in EMG frequency measures. During incremental cycle ergometry to exhaustion, however, there is a lack of consistency in the patterns of responses for EMG frequency. To determine whether the consistencies in EMG frequency were from the mode of exercise (multijoint vs single-joint), Malek and colleagues had the same subjects perform incremental cycle ergometry and double-legged knee-extensor ergometry. Briefly, the knee-extensor ergometry is a model of isolating the exercise workout to the quadriceps femoris muscles with little to no neuromuscular activity in other lower limb muscles. The investigators found that the patterns of responses for EMG frequency were still inconsistent during incremental exercise regardless of whether the mode was multijoint or single-joint. Several recent studies have successfully attempted to use the EMG frequency domain as an index of neuromuscular fatigue during isometric muscle action of the biceps brachii or the vastus lateralis during cycle ergometry. Future studies, however, are needed to validate these new methods and determine their application in rehabilitation settings.
MMG
The previous section discussed the use of EMG for examining motor control strategies and determining contributors to neuromuscular fatigue under various perturbations. This section introduces MMG, which can be used in conjunction with EMG to examine neuromuscular function. MMG is the recording and quantification of sounds produced by low-frequency lateral oscillation of muscle fibers. Although in more recent years the term MMG has been more routinely used, initial studies used various terms such as vibromyography , sound myogram , or acoustic myography to describe this phenomenon of the muscle. Regardless of terminology, investigators have suggested that MMG reflects the mechanical counterpart of motor unit activity measured by EMG. In addition, several investigators have found that the lateral oscillations reflected in the MMG signal are generated by (1) a gross lateral movement of the muscle at the initiation of a contraction that is generated by nonsimultaneous activation of muscle fibers, (2) smaller sequential lateral oscillations occurring at the resonant frequency of the muscle, and (3) dimensional changes of the active muscle fibers. Similar to the EMG signal, the MMG signal has a time and frequency domain, with the time domain related to motor unit recruitment and the frequency domain being unique in that it provides information regarding motor unit firing rates.
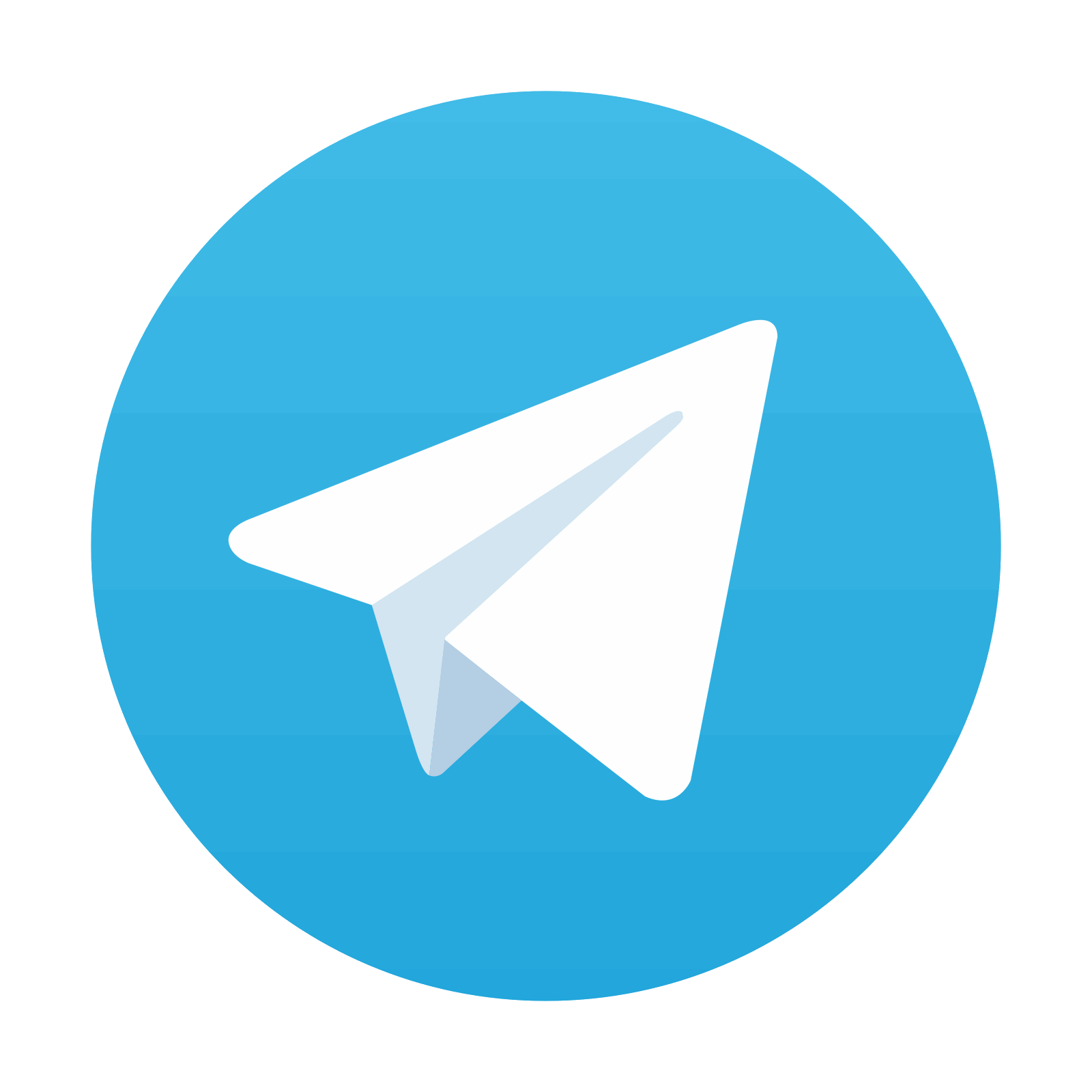
Stay updated, free articles. Join our Telegram channel

Full access? Get Clinical Tree
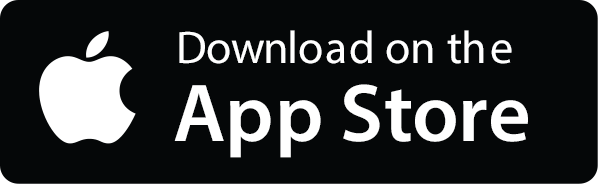
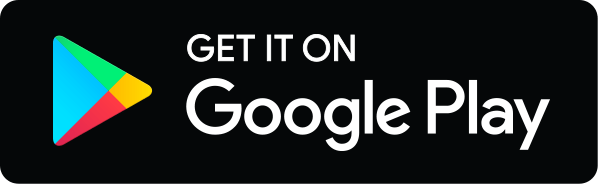