Cell type
Associated growth factors
Leukocytes
PDGF, VEGF
Neutrophils
MMP-9, IL-1B
Monocytes
IL-1B
Platelets
PDGF-AA, PDGF-AB, PDGF-BB, VEGF, TGF-B1
Up to 70% of growth factor content from activated PRP can be released over 10 min [16].
Since the earliest reports of its clinical use in the 1980s and 1990s, PRP therapy has gained popularity in regenerative medicine. In the beginning, it was used to manage dermatologic and oromaxillofacial conditions, in fact Whitman et al. described the use of a platelet gel in 1997 for oral and maxillofacial surgery [17].
More recently the interest has grown exponentially in the potential use of PRP in orthopedic applications such as bone and soft tissue regeneration (tendon and muscle tissue) or as an adjunct in surgical reconstruction procedures (ACL reconstruction, cuff tears repair) especially in high-level sport traumatology [18–24].
Generally PRP has been defined as an autologous plasma derivative in which the concentration of platelets is higher above the baseline. In humans, the normal platelet count in whole blood ranges from approximately 150,000 to 350,000/μL [25], whereas platelet-rich plasma is often defined as at least 1,000,000 platelet/μL suspended in plasma [26].
However, recent articles have indicated different ranges. Giusti et al., for example, prepared platelet concentrates between 300,000/μL and 7.5 million/μL and found that the optimal platelets concentration for cultured endothelial cell proliferation was 1.5 million/mL (5–7 baseline) [27].
In order to ensure that the platelets are suspended and do not form a clot, the platelet-rich plasma must be made from anticoagulated blood. However, its preparations have significant variability, which has led to the proposal of several PRP classification systems [28, 29].
It is well known that platelet-rich plasma preparations are not the same and that not all these include white blood cells [30].
Dohan Ehrenfest et al. suggested a classification that takes into consideration the different characteristics of the preparations: leukocyte-poor PRP, pure PRP (PPRP), leukocyte-rich PRP (LPRP), pure platelet-rich fibrin, and leukocyte- and platelet-rich fibrin [31].
All these preparation techniques have several things in common. Blood is first collected from the patient with anticoagulant and immediately centrifuged within the hour. This initial centrifugation separates red blood cells (RBCs) from acellular, platelet-poor plasma (PPP) and the “buffy coat,” which contains concentrated platelets and ± white blood cells (WBCs). Through various other steps, the RBC and PPP layers are discarded and the platelet concentrate remains [32, 33].
The role of the leukocytes has not been yet well defined: they could dispatch an antimicrobial effect, but no significant correlation between the leukocyte concentration in platelet-rich plasma and antimicrobial activity has been found [34].
Other classifications were defined: one of the most recent was proposed by Mautner K. et al. in 2015: the PLRA (platelet count, leukocyte presence, red blood cell presence, and use of activation) classification system. Authors believe that this system reflects clinically important PRP characteristics based on contemporary literature, and it can be easily adopted for research and communication [35].
Other studies have investigated the presence of growth factors in PRP: substantial differences have been observed in all the preparation methods [36–38].
The most important cytokines contained within platelets include insulin-like growth factor (IGF-1), transforming growth factor-β (TGF-β), platelet-derived growth factor (PDGF), fibroblast growth factor (FGF), epidermal growth factor (EGF), and vascular endothelial growth factor (VEGF) [25] [Table 20.2].
Table 20.2
Growth factors and their functions
Growth factors | Functions |
---|---|
PDGF | Stimulates cell proliferation, chemotaxis, and differentiation |
Stimulates angiogenesis | |
TGF-b | Stimulates production of collagen type I and type III, angiogenesis, reepithelialization, and synthesis of protease inhibitors to inhibit collagen breakdown |
VEGF | Stimulates angiogenesis by regulating endothelial cell proliferation and migration |
EGF | Influences cell proliferation and cytoprotection |
Accelerates reepithelialization | |
Increases tensile strength in wounds | |
Facilitates organization of granulation tissue | |
IGF-1 | Regulates cell proliferation and differentiation |
Influences matrix secretion from osteoblasts and production of proteoglycan, collagen, and other noncollagen proteins | |
FGF | Stimulates angiogenesis |
Promotes stem cell differentiation and cell proliferation | |
Promotes collagen production and tissue repair |
The precise relationship between platelet count and growth factors concentrations remains unclear. Some studies have shown that platelet count is correlated with the type and quantity of growth factors released following PRP treatment. For example, Sundman et al. recently found positive correlations between platelet counts and both TGF-β 1 and PDGF-AB concentrations within PRP preparations [39].
Despite this, Eppley et al. showed little correlation between platelet number and growth factors concentrations, with high variation from patient to patient [40].
Instead, Zimmerman et al. have demonstrated that storage conditions such as temperature and time from initial preparation influence the total growth factors’ content [41].
The relationship between platelet count and cellular response is ambiguous. A comparison of the effects of different platelet concentrations on fibroblast expression of type I collagen levels did not show a dose-response relationship to increasing platelet concentrations [42].
Today many commercial platelet separation systems are available, many of which may vary significantly in the relative amounts of platelets, leukocytes, erythrocytes, and anabolic and catabolic growth factors.
This multitude of systems has an important implication that is difficult to generalize results from clinical trials using different commercial PRP preparations in heterogeneous pools of muscle injuries, in terms of type and grade. A most recent 2014 Cochrane Review of single-center, randomized controlled trials (RCTs) of PRP in the literature reveals that evidence for the primary outcomes of function and pain are of low quality and at high risk of bias [43].
On the other hand, investigations are needed to determine the ideal PRP composition, while large clinical trials with standardized reporting of formulations used are needed to determine the real PRP efficacy.
20.3 Platelet-Rich Plasma and Muscle Injuries: What Is the Evidence?
20.3.1 The Healing Process of Muscle Injuries
The cellular environment in which platelet-rich preparations are placed strongly influences the direction and magnitude of tissue response. In orthopedics, the majority of PRP research has focused on its effects on connective tissues such as the tendon, ligament, and muscle. Healing is classically defined as a complex and dynamic process resulting in the restoration of anatomic continuity and function. In general, it can be separated into three phases: inflammation, proliferation, and remodeling [44].
The initial inflammatory phase can last up to 72 h, involves a number of inflammatory responses, and is usually characterized by pain, swelling, redness, and increased local temperature. It is also defined by hemostasis, with platelets establishing clot formation, and the release of growth factors that aid in activating and attracting inflammatory cells like neutrophils and macrophages to the site of injury. The proliferation phase, which lasts from 48 h up to 6 weeks, is characterized by the construction of an extracellular matrix associated with granulation, contraction, and epithelialization. Finally, the remodeling phase, lasts from 3 weeks up to 12 months, is defined by collagen remodeling, leading to an increase in the functional capabilities of the injured tissues. The physiologic progression through these phases of wound healing is orchestrated by growth factors and cytokines, many of which are released and modulated by blood components in PRP [45–47].
20.3.2 The Use of Platelet-Rich Plasma in Muscle Injuries
With the exception of muscle complete ruptures/avulsions, complications (like myositis ossificans), and the persistence of uncomfortable symptoms in chronic injuries, almost all the acute muscle damages are usually treated nonsurgically. Experimental and clinical studies demonstrate that myogenesis is not restricted only to the prenatal period but may also occur during the healing after a muscle tissue damages [48].
In the literature, many studies have shown beneficial effect with the use of PRP in traumatic muscle pathologies. The proposed mechanisms, with in vitro and in vivo assessments, include an increase of activation of satellite cells improving the diameter of regenerating fibers and the enhancement of the myogenesis and healing response.
For example, in an experimental in vitro model conducted on the gastrocnemius muscle of rats, Wright-Carpenter et al. observed accelerated activation of satellite cells, 30–48 h after injury, and an increase in the diameter of the muscle fibers undergoing regeneration, during the first week after injury [49].
The authors also observed an increased concentration of FGF-2 (460%) and TGF-beta1 (82%) through ELISA testing and suggested that these could have been responsible for the accelerated regeneration, due to the proliferative and chemotactic characteristics. In the same way, Hammond et al., analyzing major eccentric injuries of the anterior tibial muscle of rats, observed that the time taken for muscle regeneration was significantly shorter in the group treated with PRP. This paper suggested that acceleration of myogenesis was probably the mechanism responsible for this effect from platelet-rich plasma injections [50].
Harris et al. therefore reported, with histological evaluation, the persistence (but with a decreased amount) for a prolonged time of inflammatory infiltrated cells in muscle tissues of healthy rabbits that received PRP, but not the same result as those that received only saline solution [51].
In contrast, Gigante et al. did not note any difference regarding the inflammatory process between muscle injuries in rats treated with PRP and those that were not treated. Despite this, the authors showed an improvement of muscle regeneration and long-term vascularization in the first group [52].
However, in literature there are conflicting opinions about the use of PRP in the human muscle injuries. Wright-Carpenter et al., for example, with a retrospective, nonrandomized, and non-blinded study using autologous conditioned serum (ACS) of 18 professional sportsmen (with a variety of muscle strains), reported shorter recovery time from injury than a control group (treated with Actovegin; Nycomed, Zurich, Switzerland). ACS contains essentially activated PRP, which produces a lower yield of growth factors than most PRP [53].
In the same way, Bubnov et al. evaluated the efficacy of ultrasound-guided PRP treatment, comparing them with the traditional conservative therapy. In this randomized study, 30 patients with 34 lesions were enrolled; the authors reported faster pain relief and a greater range of motion recovery in the group of patients treated using PRP than the control group [9].
Also Zanon et al. in 2016 reported, in a case series of level IV, the advantages of the use of platelet-rich plasma injections in hamstring lesions in a large series of professional football players. With a mean follow-up of 36.6 months (range 22–42), 25 hamstring injuries (grade 2 according to MRI classification) were studied. The authors showed good/excellent results if this technique was applied not before than 24 h from the traumatic event?? meaning and using an ultrasound-guided procedure. PRP-treated lesions did not heal more quickly than untreated lesions described in the literature, but they showed a smaller scar and excellent repair tissue in a midterm follow-up [6].
Despite this, completely different results have been also published. For example, Guillodo et al. published a cohort study evaluating the time to return to play (TTRTP) in athletes with grade III acute hamstring injuries treated with one PRP injection. In this paper, only 15 of 34 patients underwent this procedure. The remaining 19 athletes were considered a control group and treated with a conservative strategy. The same standardized rehabilitation program was then used in both groups. The mean TTRTP at the pre-injury level was 50.9 ± 10.7 days in the PRP group and 52.8 ± 15.7 days in the control group. The authors did not find statistically significant differences [54].
The same conclusion was found in a double-blind placebo-controlled trial conducted in three study centers. Reurink et al. demonstrated no benefit, in patients with acute hamstring injuries, using two intramuscular PRP injections compared with placebo. In this study, the authors randomly assigned patients to receive intramuscular injections of PRP or isotonic saline (as a placebo) in 80 competitive and recreational athletes with acute hamstring muscle damage [55]. The median time until the resumption of sports activity was 42 days (interquartile range, 30 to 58) in the PRP group and 42 days (interquartile range, 37 to 56) in the placebo group. The reinjury rate was 16% in the PRP group and 14% in the placebo group.
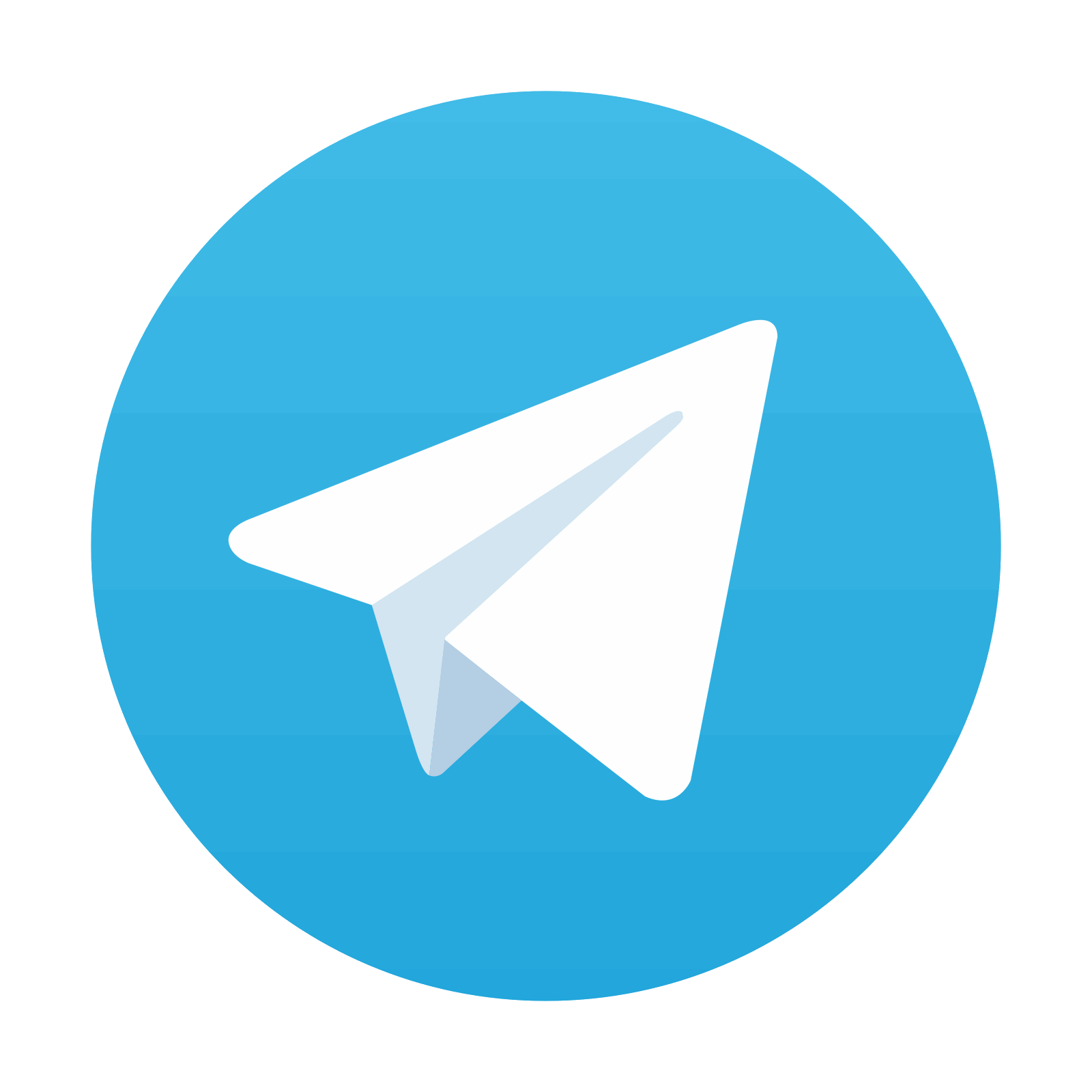
Stay updated, free articles. Join our Telegram channel

Full access? Get Clinical Tree
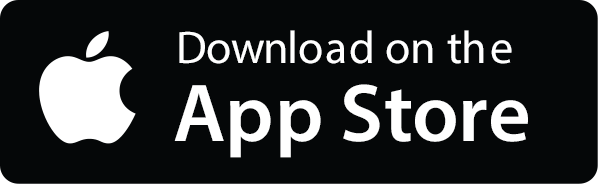
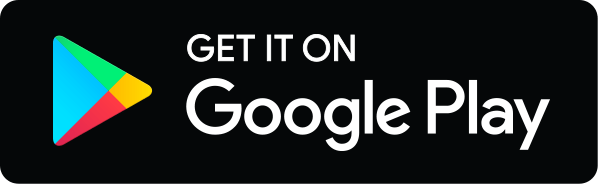