Fig. 6.1
Overview on the synthesis of the large (60S) and small (40S) subunits of the ribosome. RPs ribosomal proteins
Ribosome biogenesis is intimately linked to cell growth and proliferation in organisms from yeast to higher eukaryotes. Accordingly, the rate of cell growth in both yeast and cancer cells is highly correlated to the level of ribosome biogenesis [8, 65]. Given that ribosome biogenesis is critical for the viability of the organism or cell, the production of ribosomes is highly conserved, thus allowing the process to be extensively studied in a more tractable model system such as yeast [65]. Although the relationship between cell growth and translational capacity is well established in yeast, much less is known regarding the role of ribosome biogenesis in multinucleated cells such as skeletal muscle.
6.3.1 rDNA Transcription
The rDNA gene is organized into tandem repeats that are spread throughout the mammalian genome with somatic cells containing approximately 200 copies of the rDNA gene (Fig. 6.1); however, not all rDNA copies are actively transcribed [30]. The regulation of the rDNA repeats’ conformation is important for ribosome biogenesis [19]. The nucleolus is an extremely prominent region within the nucleus where rDNA transcription occurs and is heavily packed with proteins responsible for synthesis of the 45S pre-rRNA and ribosome assembly (Fig. 6.2).


Fig. 6.2
Organization of the ribosomal DNA (rDNA) gene. Ribosomal DNA gene is arranged in approximately 200 tandem repeats. The intergenic spacer (IGS) regions are non-transcribed regions separating rDNA gene copies and where the promoter regions – core promoter region (Core) and the upstream promoter element (UPE) – are located. The pre-rRNA is transcribed as a single transcript containing the mature rRNAs in addition to both internal and external transcribed spacers (ITS, ETS)
The Pol I enzyme requires the formation of a protein complex – the preinitiation complex (PIC) – at the rDNA promoter region. The upstream binding factor (UBF) is the first protein attaching to the rDNA. UBF binds physically to the promoter region (both UPE and the core promoters) via high mobility group (HMG) box domains, which confers DNA-binding activity of UBF. This association is the basic mechanism for the formation of the enhanceosome at the rRNA gene. The ribosomal enhanceosome is an almost 360° loop on the promoter elements allowing PIC formation for subsequent Pol I transcription [53]. Following enhanceosome formation, selectivity factor 1 (SL1) complex (formed by several TATA-binding proteins) associates with UBF and the rDNA [13]. The SL1 complex binds to the promoter region alongside UBF and recruits TIF-IA (also known as RRN3) and Pol I to initiate transcription [45]. TIF-IA, via protein-protein interactions, associates with SL1 and Pol I enzyme complex [5, 6], serving as a bridge between PIC and PolI[8, 18, 38].
Following synthesis, the 45S pre-rRNA will be processed into the mature rRNAs for maturation with ribosomal protein into the 40S and 60S subunits. First, the ETS and ITS regions are removed by nucleases and then degraded [20]. The majority of the processing occurs while pre-rRNA is still in the nucleolus, although some processing also occurs in the nucleoplasm and cytoplasm [20]. In addition to the processing of the 45S, the 5S rRNA transcribed by the Pol III and the ribosomal proteins translated in the cytoplasm will also join the rRNA processing and maturation to form the ribosomal subunits [29].
6.3.2 Upstream Pathways
The majority of total cellular RNA is by far rRNA. Depending on the cell, as much as 80% of extracted RNA in tissue or cells can be rRNAs [61]. In proliferating cells, the majority of new transcripts are rRNA to make new ribosomes and support growth [61]. Due to the high cost of production, the process of making ribosomes is tightly regulated at many levels by nutritional, hormonal, and energy-sensing cues. It is widely known that many growth-related pathways, such as PI3K-Akt-mTOR axis and MAPK/ERK, trigger cell growth via increased rates of translation, especially at initiation and elongation steps. Those same pathways can also alter the rate of pre-rRNA synthesis. The transcription of rDNA is the central process governing ribosome biogenesis, in which the formation of the preinitiation complex (PIC) at the rDNA promoter is regulated by those pathways, such as mTOR and MAPK (Fig.6.3).


Fig. 6.3
Assembly of PIC at the rDNA promoter and pathways affecting components of PIC formation
The mammalian target of rapamycin (mTOR) is a master kinase controlling ribosome activity and its biogenesis. mTOR complex 1 (mTORC1) controls protein synthesis by phosphorylating many targets, especially the 4E-BP1 and p70S6K at the TOS motif sequence [47]. Hyperphosphorylation of 4E-BP1 releases the inhibitory effect of 4E-BP1 on translation initiation of 5’TOP mRNAs. Members of this selected group of mRNAs encode proteins involved in cellular growth. The majority of mRNA translation that 4E-BP protein inhibits encodes ribosomal proteins, mitochondrial ribosomal proteins, and proteins that associate with ribosomes to drive translation, the eukaryotic initiation, and elongation factors (eIFs and eEfs) [55]. mTORC1 also regulates the transcription of the RNA polymerases I, II, and III (Pol I, II, and III) [34, 56]. In addition to these effects, mTOR also promote the phosphorylation of TIF-IA [35]. Therefore, activation of mTORC1 increases translation efficiency and transcription of the RNA polymerases toward augmentation of translational capacity, supporting cell growth in addition to modulating PIC formation at the rDNA promoter (Fig. 6.4).


Fig. 6.4
mTORC1 promotes ribosome biogenesis and increase in translational capacity at multiple levels
In addition to mTORC1, the MAPK pathway also promotes ribosome biogenesis. Different proteins of MAPK pathway also directly phosphorylate key proteins promoting assembly of the PIC components at the rDNA promoter. ERK and RSK proteins can phosphorylate TIF-IA and UBF proteins and promote rDNA transcription [52, 54, 66]. In addition, as ribosome biogenesis is also important in cell proliferation, cell cycle regulators, such as the cyclin-dependent kinases (CDK), have also been shown to modulate ribosome biogenesis. CDKs can phosphorylate UBF directly [57, 58] and TIF-IA [35]. In particular, CDK4, when complexed with cyclin D1, can directly phosphorylate UBF protein [58]. Moreover, the pro-oncogene protein c-Myc, known for its role in cell cycle and proliferation, also promotes ribosome biogenesis. The c-Myc protein has been shown to directly interact with PIC components at the rDNA promoter [1].
Ribosome biogenesis is a highly metabolic demanding cellular process, and as such, AMPK has been found to be an important factor linking energy balance with rDNA transcription. Not only can AMPK interfere with ribosome biogenesis via its regulation at mTORC1 and translation initiation level [31], but AMPK has also been found to interact with PIC components. AMPK can directly phosphorylate and destabilize TIF-IA association with SL1 [21] impacting negatively in the rate of pre-rRNA synthesis (Fig. 6.3).
6.4 Ribosome Biogenesis in Skeletal Muscle Hypertrophy and Atrophy
6.4.1 Hypertrophy
In animal models of muscle growth, ribosome biogenesis has been shown to precede muscle growth. Mechanical load of the plantaris muscle, via synergistic ablation of the plantar flexor muscles, markedly increases the expression of UBF, c-Myc, TIF-IA, and SL-1 components in addition to promoting the association of UBF, c-Myc, and Pol I with the rDNA promoter [59]. These events lead to a robust increase in rDNA transcription and accumulation of mature rRNAs [27, 28, 59]. Importantly, the degree of changes in mature rRNA has been shown to correlate with changes in muscle mass using this model of hypertrophy [43].
Following these key studies in muscle ribosome in animal models, a few studies have also investigated muscle ribosome biogenesis in humans. Specifically, the response to resistance exercise has been evaluated in recent years. Consistent with the animal studies, a single bout of resistance exercise is capable of increasing expression of 45S pre-rRNA [11, 42, 51]. Chronically, the repeated increases in ribosome biogenesis induced by resistance exercise lead to accretion in mature rRNA [9, 50]. Moreover, recent independent investigations using resistance exercise in rodents have also clearly demonstrated that a single bout and chronic training leads to pre-rRNA synthesis and accumulation of mature rRNAs [44, 63].
The mechanism leading to increased rDNA transcription following a bout of resistance exercise has been not fully understood; however, some recent studies have shed light on the pathways involved in promoting ribosome biogenesis in response to resistance exercise. Given the importance of both MAPK and mTORC1 signaling pathways in the regulation of ribosome biogenesis, it is not surprising these pathways are activated following resistance exercise. For instance, UBF, as a central regulator of rDNA transcription, is subjected to phosphorylation via both MAPK and mTORC1 pathways. Recent studies have shown that resistance exercise promotes a strong activation leading to UBF phosphorylation at multiple residues [11, 63]. In addition to this, another component of PIC formation, TIF-IA, has also been shown to rapidly increase in phosphorylation levels shortly after resistance exercise at an MAPK-pathway-dependent site [9, 11].
Overall, these studies support the notion that muscle hypertrophy in adults observed with mechanical stimulation is a result of greater muscle translational capacity, achieved via increased ribosome biogenesis.
6.4.2 Atrophy
Muscle atrophy is usually associated with an increase in muscle protein degradation rates [7, 46]. This is especially true for a highly atrophic condition in which significant muscle loss is observed in a short period of time, such as in sepsis, cachexia, immobilization/bed rest, and denervation. However, the contribution of protein synthesis also has been brought to attention, as recent studies have shown that, for instance, disuse can also decrease protein synthesis rates at basal and postprandial state [60]. Moreover, smaller basal protein synthesis but potentially undetectable by current methods could contribute to the loss of muscle mass in cancer patient [22]. Therefore, impairment in muscle ribosome biogenesis could also contribute to both acute and chronic muscle wasting conditions. A few studies so far have tried to elucidate the roles of muscle ribosome biogenesis in muscle atrophy.
Machida et al. [32] studied the effects of muscle denervation on muscle atrophy. Both 45S pre-rRNA and mature rRNAs were markedly reduced following denervation. Similarly, a rodent model of chronic inflammation also caused an impairment in muscle ribosome biogenesis, as 45S, 28S, 18S, and 5.8S rRNAs were decreased following the onset of muscle inflammation, leading to decreased muscle mass and size [10].
Moreover the inability to mount a significant increase in pre-rRNA and mature rRNA expression in response to resistance exercise in humans [51] and mechanical loading in mice [27] has also been shown recently in aged in comparison with young cohorts. Thus, impaired ribosome biogenesis may also contribute to the blunted response to resistance exercise in older individuals.
Combined, these data suggest that muscle disuse and other muscle atrophic conditions and decreased translational capacity due to impaired ribosome biogenesis can be a major contributor to muscle wasting. Moreover, these data also highlight the potential links between ribosome biogenesis and sarcopenia.
6.5 Conclusion and Future Directions
Many recent studies have shown that changes in ribosome biogenesis and translational capacity correlate well with changes in muscle mass in both growth and wasting conditions [9, 10, 43, 50]. Thus, muscle ribosome biogenesis appears to be necessary for muscle hypertrophy [62]. However, more mechanistic and proof-of-principle evidences are necessary to stablish a causal relationship between ribosome biogenesis and muscle growth.
The regulation of ribosome biogenesis and translational capacity is an open venue for muscle biology researchers with interest in both the fundamental understanding of the molecular mechanisms of muscle growth and also of great applicability for clinical research. Novel aspects of regulation of muscle ribosome biogenesis may help elucidate the mechanism of muscle adaptation to different stimuli, providing tools to better design interventions of clinical interest. In addition to resistance exercise, endurance training studies will also further our understanding regarding the different exercise stimuli on ribosome biogenesis and the potential influence on skeletal muscle phenotypes. Moreover, of clinical relevance, novel therapeutic approaches to ameliorate the loss of muscle mass during muscle disuse, chronic inflammatory conditions, cancer cachexia, and sarcopenia can be developed through the elucidation of the role of ribosome biogenesis in skeletal muscle size regulation.
References
1.
2.
Atherton PJ, Miller BF, Burd NA, Macnaughton LS, Murton AJ, Camera DM, Pancheva AV, Wang X, Vagula MC, Phillips BE, Brook MS, Wilkinson DJ, Smith K, Etheridge TE, Hamilton KL, Beals JW, van Vliet S, van Loon LJC, Wardle SL, Tipton KD, Stephens FB, Billeter R, Wall BT, Hawley JA, Panchev VS, Pancheva MV (2015) Commentaries on viewpoint: what is the relationship between acute measure of muscle protein synthesis and changes in muscle mass? J Appl Physiol 118:498–503CrossRef
3.
Atherton PJ, Smith K (2012) Muscle protein synthesis in response to nutrition and exercise. J Physiol 590:1049–1057CrossRefPubMedPubMedCentral
4.
Balagopal P, Schimke JC, Ades P, Adey D, Nair KS (2001) Age effect on transcript levels and synthesis rate of muscle MHC and response to resistance exercise. Am J Physiol Endocrinol Metab 280:E203–E208PubMed
5.
Blattner C, Jennebach S, Herzog F, Mayer A, Cheung ACM, Witte G, Lorenzen K, Hopfner K-P, Heck AJR, Aebersold R, Cramer P (2011) Molecular basis of Rrn3-regulated RNA polymerase I initiation and cell growth. Genes Dev 25:2093–2105CrossRefPubMedPubMedCentral
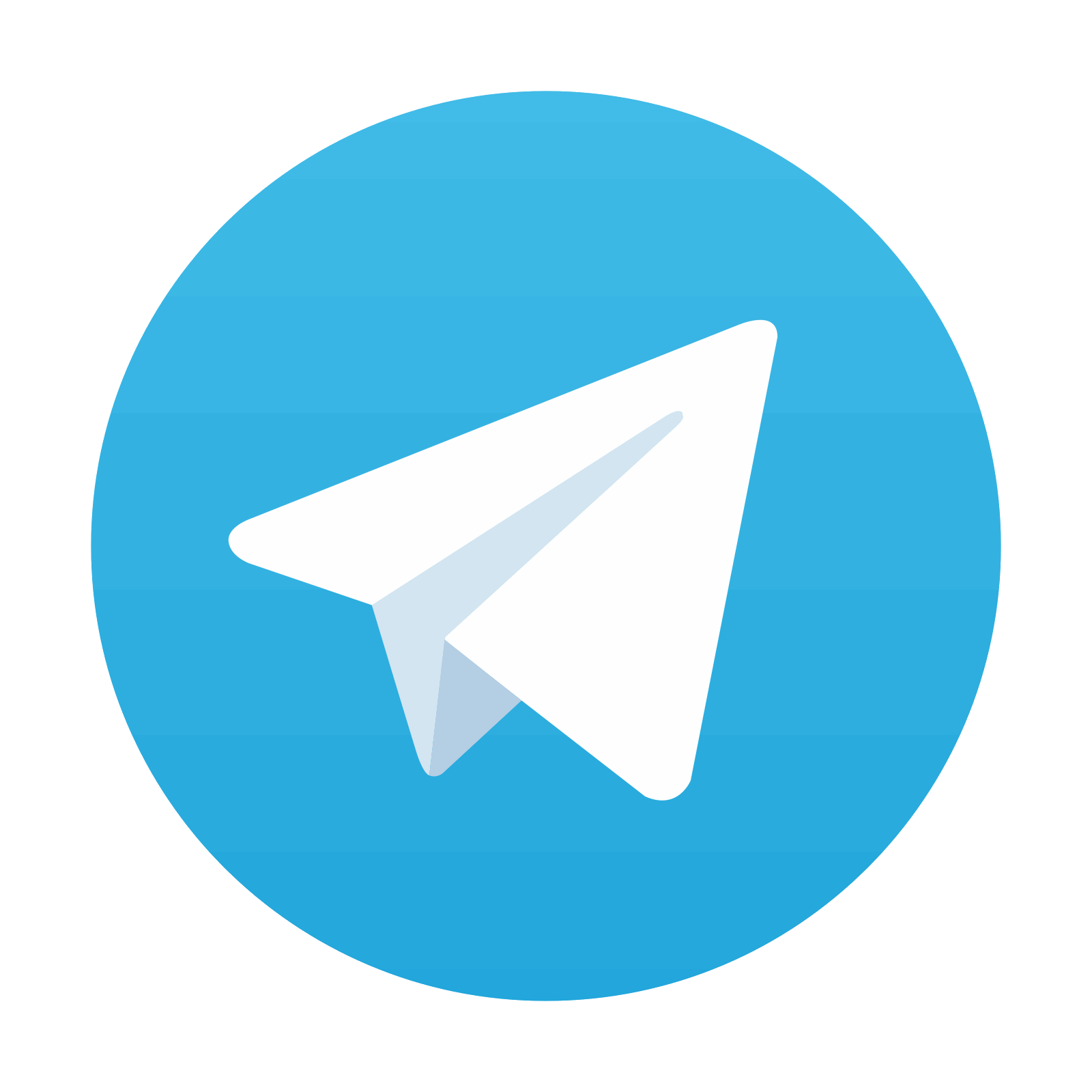
Stay updated, free articles. Join our Telegram channel

Full access? Get Clinical Tree
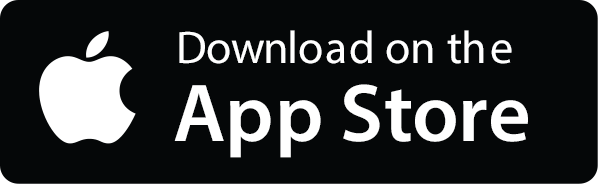
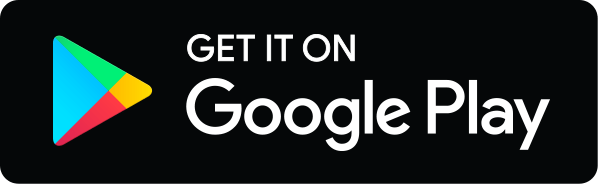