Fig. 2.1
Schematic diagram of the 26S proteasome. The 26S proteasome consists of the 20S core particle (CP) and the 19S regulatory particle (RP). The CP is made up of four heptameric rings: two outer identical α-rings and two identical inner β-rings. Each ring is made of seven distinct homologous subunits. Certain β-subunits contain the protease active sites facing inward into the sequestered proteolytic chamber. The 19S RP is divided into two subcomplexes: the lid and the base. The subunits forming the lid and the base are shown. Rpn10 can interact with either the lid or the base and stabilizes the interaction between the two
2.1.2 The Ubiquitin-Proteasome System
In the ubiquitin-proteasome system (UPS), proteins are targeted for degradation by the 26S proteasome through covalent attachment of a chain of ubiquitin molecules. The ubiquitin ligase enzyme, or E3, binds the protein substrate and catalyzes the movement of the ubiquitin from the E2 enzyme to the substrate. This is the rate-limiting step of the ubiquitination process, which affects the subsequent proteasome-dependent degradation. Once the protein is ubiquitinated, it is docked to the proteasome for degradation, unless the polyubiquitin chain is removed by the deubiquitinating enzymes. Among the different E3s, only a few have been found to regulate the atrophic process and to be transcriptionally induced in atrophying muscle.
The regulation of skeletal muscle mass largely depends on protein synthesis and degradation processes. Two muscle-specific E3 ubiquitin ligases, muscle RING finger 1 (MuRF1) and muscle atrophy F-box (atrogin-1/MAFbx), are believed to be key regulators of proteasomal proteolysis in skeletal muscle, particularly under atrophy-inducing conditions [9–11]. These two E3s are specifically expressed in muscle atrophy [12, 13]. Atrogin-1/MAFbx and MuRF1 knockout mice are resistant to muscle atrophy induced by denervation [12]. Also, knockdown of atrogin-1 spares muscle mass in fasted animals [14]. Thus far, MuRF1 ubiquitinates several muscle structural proteins, including troponin I [15], myosin heavy chains [16], actin [17], myosin-binding protein C, and myosin light chains 1 and 2 [18], whereas the substrates of atrogin-1 that have been identified appear to be involved in growth-related processes or survival pathways. Atrogin-1 promotes degradation of MyoD, a key muscle transcription factor, and of eIF3-f, an important activator of protein synthesis [19, 20].
Another E3 ubiquitin ligase found to play a critical role in atrophy is TNF receptor-associated factor 6 (TRAF6) [21], which mediates the conjugation of Lys63-linked polyubiquitin chains to target proteins. Lys48-linked polyubiquitin chains are a signal for proteasome-dependent degradation, but Lys63-linked polyubiquitin chains play other roles, such as regulating autophagy-dependent cargo recognition by interacting with the scaffold protein p62 (also known as SQSTM1) [22–24]. Notably, muscle-specific TRAF6 knockout mice have a decreased amount of polyubiquitinated proteins, with almost no Lys63-polyubiquitinated proteins in starved muscles [25], and are resistant to muscle loss induced by denervation, cancer, or starvation [21, 25, 26]. The mechanism of this protection involves both direct and indirect effects of TRAF6 on protein breakdown. Inhibition of TRAF6 reduces the induction of atrogin-1 and MuRF1, thereby preserving muscle mass under catabolic conditions. Moreover, TRAF6-mediated ubiquitination may have an additional function on modulating intracellular signaling. In fact, TRAF6 is required for the optimal activation of c-Jun N-terminal kinase (JNK), AMP-activated protein kinase (AMPK), and forkhead box O3 transcription factor (FoxO3) pathways [25]. The effects on FoxO3 and nuclear factor κB (NF-κB) may explain why atrogin-1 and MuRF1 are less induced in TRFAF6 knockout mice.
In skeletal muscle, E3 ligases have important regulatory functions in signaling pathways. For example, it was recently found that anabolic signals were regulated by the ubiquitin ligase F-box protein 40 (Fbxo40) [27]. Fbxo40 ubiquitinates and affects the degradation of insulin receptor substrate 1 (IRS1), a downstream effector of insulin receptor-mediated signaling. Inhibition of Fbxo40 by RNA interference induces hypertrophy in myotubes, and Fbxo40 knockout mice display bigger muscle fibers [27].
2.1.3 Muscle-Specific Proteasome Dysfunction Mice
Proteasomal degradation is mediated by an ATP-dependent protease complex, the 26S proteasome, which is present in both the cytoplasm and nucleus. The 26S proteasome consists of the 20S proteasome and the 19S regulatory particles. The 19S regulatory particles unfold ubiquitin-conjugated proteins to enable their entry into the 20S proteasome. The 19S regulatory particles contain several putative ATPases, such as PSMC1–PSMC6 (proteasome 26S subunit, ATPase, 1–6). These subunits form a large family with a highly conserved ATPase domain [28]. PSMC4, also known as Rpt3 (proteasomal regulatory particle AAA-ATPase-3; Fig. 2.1), is an essential subunit of the 26S proteasome and is required for the degradation of most proteasomal substrates. In particular, Rpt3-deficient mice die before implantation because of a defect in blastocyst development [28]. Interestingly, an insertion/deletion variant in intron 5 of the Rpt3 gene was frequently found in a cohort of patients with Parkinson’s disease [29]. Recently, Tashiro et al. reported that the conditional knockout of the proteasome subunit Rpt3 in motor neurons caused locomotor dysfunction that was accompanied by progressive motor neuron loss and gliosis in mice [30].
We also generated conditional Rpt3-knockout mice to specifically block proteasomal activity in skeletal muscle to clarify the role of the proteasomal system in skeletal muscle tissue (Fig. 2.2). Accordingly, we reported that muscle-specific deletion of a crucial proteasomal gene, Rpt3, resulted in profound muscle growth defects and a decrease in force production in mice with the accumulation of abnormal proteins [31]. In contrast to previous studies using proteasome inhibitors on dystrophic or myostatin-defective mice, in which muscle hypertrophy was demonstrated [32], the specific deletion of the proteasome component Rpt3 resulted in a significant loss of muscle mass with premature death and significantly reduced physical activity. Furthermore, our study suggests that both the UPS and autophagy systems are required to maintain myocellular homeostasis and integrity. The protein and organelle degradation by both autophagy and UPS may provide resources, such as oligopeptides and amino acids, for maintaining cellular integrity in skeletal muscle tissue [33]. Therefore, Rpt3 deficiency may result in a far more serious condition that deprives the cells of two major pathways responsible for pooling resources for cellular maintenance. We hypothesize that the skeletal muscle Rpt3 deficiency may have resulted in blocking the cellular “recycling system” that is essential for maintaining skeletal muscle fibers; this question needs to be further examined.


Fig. 2.2
A schematic of the pathophysiology of muscle-specific proteasome dysfunction mice. Usually, the ubiquitination of misfolded proteins are degraded by both the UPS and autophagy. In Rpt3 deficiency mice, the UPS is impaired, and the autophagy system is upregulated. The dysfunction of the UPS leads to the accumulation of ubiquitinated proteins. Finally, basophilic inclusions and inflammatory cells are found in the myofibers. Our results suggest that appropriate proteasomal activity is important for muscle growth and for maintaining myofiber integrity in collaboration with autophagy pathways [31]. The protein and organelle degradation by both UPS and autophagy may provide resources, such as oligopeptides and amino acids, for maintaining cellular integrity of the skeletal muscle tissue [33]. Therefore, the proteasome dysfunction mice may have resulted in a far more serious condition that deprives the cells of two major pathways responsible for pooling resources for cellular maintenance. We hypothesize that the skeletal muscle proteasome dysfunction may have led to blocking the cellular “recycling system” that is essential to the maintenance of skeletal muscle fibers, and this question needs to be further examined
2.2 Muscle Disease and the Proteasome System
2.2.1 Sporadic Inclusion Body Myositis
Sporadic inclusion body myositis (sIBM) is the most common form of inflammatory myopathy in those older than 50 years of age in Western countries [34–36]. Muscle weakness and atrophy in the quadriceps, wrist flexors, and finger flexors are the typical neurological findings of sIBM. The muscle biopsy typically reveals endomysial inflammation, the invasion of mononuclear cells into non-necrotic fibers, and rimmed vacuoles; these findings suggest that inflammation and degeneration are co-existent in the pathological mechanisms. An abnormal accumulation of intracellular multiprotein inclusions is a characteristic feature of the sIBM phenotype, and, as such, sIBM is considered to be caused by protein unfolding/misfolding combined with the formation of inclusion bodies [35]. The 26S proteasome system is involved in the elimination of various proteins, including ubiquitinated misfolded/unfolded proteins, and its inhibition results in the cellular accumulation of protein aggregates. Ubiquitinated protein aggregates in muscle fibers are characteristic of sIBM muscle fibers.
Two major types of aggregates exist, containing either amyloid beta (Abeta) or phosphorylated tau (ptau). Based on these findings, using cultured human muscle fibers, researchers examined the effect of Abeta precursor protein (AbetaPP) overexpression on proteasome function and the influence of proteasome inhibition on aggresome formation [37]. They reported that in sIBM muscle biopsies, the 26S proteasome subunits were immune detected in the gamma tubulin-associated aggresomes, which also contained Abeta, ptau, ubiquitin, and heat shock protein 70 (HSP70). Additionally, proteasome subunit expression was greatly increased, 20S proteasome subunit was co-immunoprecipitated with AbetaPP/Abeta, and three major proteasomal proteolytic activities were reduced. In the cultured muscle fibers, those that overexpressed AbetaPP displayed diminished proteasomal proteolytic activities, and the addition of a proteasome inhibitor strikingly increased aggresome formation. Accordingly, proteasome dysfunction in sIBM muscle fibers may play a role in the accumulation of misfolded, potentially cytotoxic proteins and may be induced by increased intracellular AbetaPP/Abeta levels. Abnormal intracellular accumulation of unfolded proteins may lead to their aggregation and the formation of inclusion bodies [38].
2.2.2 Cachexic State
Sarcopenia and cachexia are muscle wasting syndromes associated with aging and with many chronic diseases, such as congestive heart failure (CHF), diabetes, cancer, chronic obstructive pulmonary disease, and chronic kidney disease (CKD) [39]. While their mechanisms are complex, these conditions are often accompanied by elevated angiotensin II (Ang II) levels. Patients with advanced CHF or CKD often have increased Ang II levels and cachexia, and angiotensin-converting enzyme inhibitor treatment prevents weight loss [39]. Ang II-induced muscle wasting is caused by alterations in insulin-like growth factor 1 (IGF1) signaling and by enhanced muscle protein breakdown via the UPS. In another study, researchers examined diaphragm muscle biopsies from 22 critically ill patients who had received mechanical ventilation before surgery and compared these with biopsies that were obtained from patients during thoracic surgery for resection of a suspected early lung malignancy. The cross-sectional areas of both the slow and fast twitch diaphragm muscle fibers in the critically ill patients were approximately 25% smaller, and this reduced their contractile force by one-half or more. Markers of the UPS were significantly upregulated in the diaphragm of the critically ill patients. These findings showed that the diaphragm muscle fibers of the critically ill patients displayed atrophy and severe contractile weakness and that the UPS was activated in the diaphragms of critically ill patients [40].
2.2.3 Mutants in the Protein Degradation Systems
Protein aggregate myopathies (PAMs) comprise of muscle disorders characterized by protein accumulation in muscle fibers. Clinicians diagnose new cases of PAM in patients with proximal muscle weakness and hypertrophic cardiomyopathy; these muscle fibers contain inclusions containing myosin and myosin-associated proteins and have an aberrant distribution of microtubules [41]. These lesions can appear as intact A and M bands that lack thin filaments and Z discs. These features are similar to those in mice that are deficient for MuRF1 and MuRF3. Sanger sequencing excluded mutations in the myosin storage myopathy (MSM)-associated gene for β-cardiac myosin heavy chain (MYH7), but identified mutations in tripartite motif-containing 63 (TRIM63) and TRIM54, which encoded MuRF1 and MuRF3, respectively. Both MuRFs are microtubule-associated proteins that are localized to the sarcomeric M bands and Z discs. They are E3 ubiquitin ligases that play a role in the degradation of sarcomeric proteins, the stabilization of microtubules, and myogenesis. A lack of ubiquitin and the 20S proteasome subunit in the inclusions found in the patient suggested impaired turnover of the thick filament proteins. The disruption of microtubules in cultured myotubes was rescued by the transient expression of wild-type MuRF1. The unique features of this novel myopathy point to defects in the homeostasis of A-band proteins in combination with the instability of microtubules as the cause of this disease.
Mutations in the non-lysosomal cysteine protease, calpain 3, cause limb-girdle muscular dystrophy type 2A (LGMD2A) [42]. Calpain 3 may mediate remodeling by the cleavage and release of myofibrillar proteins, targeting them for ubiquitination and proteasomal degradation [43]. The rate of atrophy and particularly the rate of growth are decreased in calpain 3 knockout mouse muscles under conditions that promote sarcomere remodeling by hind limb unloading. In wild-type mice, an elevated level of ubiquitinated proteins was observed during muscle reloading, which was presumably necessary to remove atrophy-specific and damaged proteins [44]. This increase in ubiquitination correlated with an increase in calpain 3 expression. Calpain 3 knockout mouse muscles did not show any increase in ubiquitination at the reloading stage, suggesting that calpain 3 was necessary for ubiquitination and that it acted upstream of the ubiquitination machinery. These studies suggested that the accumulation of aged and damaged proteins can lead to cellular toxicity and a cell stress response in calpain 3 knockout muscles and that these characteristics were pathological features of LGMD2A.
2.2.4 Proteasomal Abnormality in Other Mutants
Mutations in the phosphoinositide phosphatase myotubularin (MTM1) result in X-linked myotubular/centronuclear myopathy (XLMTM), which is characterized by a severe decrease in muscle mass and strength in both patients and murine models [45]. Upregulation of atrogenes has been observed in the presymptomatic phase of the myopathy, which supports over-activation of the UPS.
Oculopharyngeal muscular dystrophy (OPMD) is a late-onset progressive muscle disorder caused by a poly-alanine expansion mutation in the poly-A-binding protein nuclear 1 (PABPN1). UPS is the most consistently and significantly OPMD-deregulated pathway across species [46]. The manipulations of the proteasome and immunoproteasome activity can specifically affect the accumulation and aggregation of mutant PABPN1 in vitro.
Myotonic dystrophy type 1 (DM1) is a neuromuscular disease caused by the expansion of a CTG repeat in the dystrophia myotonica protein kinase (DMPK) gene and is characterized by progressive skeletal muscle weakness and wasting. Using a transgenic mouse model that carried the human DM1 region with 550 expanded CTG repeats [47], a significant increase in trypsin-like proteasome activity and Fbxo32 expression was also measured in the DM1 muscles and indicated that an atrophic process mediated by the ubiquitin-proteasome pathway may contribute to the progressive muscle wasting and weakness in DM1 mice.
2.2.5 Nakajo-Nishimura Syndrome and Myositis
The disease spectrum currently known as the proteasome-associated auto-inflammatory syndromes (PRAAS) was first described in 1939 in patients who presented with recurrent fevers beginning in infancy or early childhood, which were accompanied by nodular erythema, a pernio-like rash, and joint contractures [48]. Since then, several syndromes, such as the chronic atypical neutrophilic dermatosis with lipodystrophy and elevated (CANDLE) temperatures syndrome; the Nakajo-Nishimura syndrome (NNS); the joint contractures, muscle atrophy, microcytic anemia, and panniculitis-induced lipodystrophy (JMP) syndrome; and the Japanese auto-inflammatory syndrome with lipodystrophy (JASL), have been used to categorize the patients that had diseases within the same spectrum, including myositis. Recently, independent studies have identified mutations in the human proteasome subunit β type 8 (PSMB8) gene, which results in a sustained inflammatory response in all syndromes [49, 50]. PSMB8 encodes a catalytic subunit of the 20S immunoproteasomes called β5i. Immunoproteasome-mediated proteolysis generates immunogenic epitopes presented by major histocompatibility complex class I molecules.
Myositis is characterized by severe muscle weakness. Stable isotope labeling with amino acids in cell culture (SILAC) has been used to identify alterations in the skeletal muscle proteome of myositic mice in vivo [51]. Using the SILAC technique, the authors identified significant alterations in the levels of proteins that belonged to the pathway including UPS [51]. There was a significant increase in the ubiquitination of muscle proteins as well as a specific increase in ubiquitin carboxyl-terminal hydrolase isozyme L1 (UCHL-1) in myositis, but there was no increase in the muscles affected by other dystrophies or in normal muscles.
2.2.6 Motor Neuron Disease
Amyotrophic lateral sclerosis (ALS) is a fatal neurodegenerative disorder characterized by the progressive loss of upper and motor neurons. In particular, the accumulation of ubiquitinated inclusions containing these gene products is a common feature in most familial ALS models; it is also a pathologic hallmark of sporadic ALS, indicating that the failure to eliminate detrimental proteins is linked to the pathogenesis of both types of ALS. The presence of ubiquitinated inclusions such as skein-like and round hyaline inclusions strongly suggested dysfunction in the UPS. Indeed, the continuous expression of mutant (superoxide dismutase 1) SOD1 was shown to decrease proteasome activity and that primary cultured embryonic motor neurons were vulnerable to proteasome inhibition by lactacystin [52]. Ubiquilin 2 and p62, which are disease-causing genes in ALS, are mainly related to the protein aggregation and degradation pathways; therefore, mutations of the ubiquilin2 and p62 genes can cause related disorders [53]. On the contrary, other reports have documented an increase in proteasome activity [54]. As described in the previous paragraph, conditional knockout of the proteasome subunit Rpt3 in a motor neuron-specific murine model showed locomotor dysfunction accompanied by progressive motor neuron loss and gliosis [30].
Spinal and bulbar muscular atrophy (SBMA) or Kennedy’s disease is an X-linked disease associated with the expansion of the CAG triplet repeat that is present at exon 1 of the androgen receptor (AR) gene. This results in the production of a mutant AR that contains an elongated poly-glutamine tract (poly-Q) in its N-terminus. Interestingly, the AR poly-Q becomes toxic only after its activation by natural androgenic ligands; this may be because of the aberrant androgen-induced conformational changes of the AR poly-Q, which generates the misfolded species. These misfolded AR poly-Q species must be cleared from the motor neurons and muscle cells, and this process is mediated by proteasomal proteolysis. Molecular chaperones and degradative pathways including the UPS, the autophagy-lysosome pathway, and the unfolded protein response, which activates endoplasmic reticulum-associated degradation, are differentially affected in SBMA [55].
The expansion of the GGGGCC hexanucleotide repeat in the noncoding region of the chromosome 9 open reading frame 72 (C9orf72) gene is the most common cause of frontotemporal dementia (FTD) and ALS (c9FTD/ALS). Recently, it has been reported that an unconventional mechanism of repeat-associated non-ATG (RAN) translation arose from C9orf72 expansion. Sense and antisense transcripts of the expanded C9orf72 repeat, such as the dipeptide repeat protein (DRP), were deposited in the brains of patients with c9FTD/ALS. The evaluation of cytotoxicity revealed that overexpressed poly-GA, poly-GP, and poly-GR increased the substrates in the UPS, including transactive response DNA-binding protein 43 (TDP-43), and enhanced the sensitivity to a proteasome inhibitor, indicating that these DRPs were cytotoxic, possibly via UPS dysfunction [56].
2.3 Therapeutic Strategy Using Intervention into the Proteasomal System
As reviewed above, some diseases present UPS dysfunction, while others represent excessive UPS activity. The suppression of excessive UPS activity is a promising method for some muscle diseases.
The 427 kDa protein dystrophin is expressed in striated muscle where it physically links the interior of the muscle fibers to the extracellular matrix. A range of mutations in the gene encoding dystrophin can lead to a severe muscular dystrophy known as Duchenne muscular dystrophy (DMD) or a typically milder form known as Becker muscular dystrophy. Dystrophin missense mutations, however, cause a wide range of phenotypic severities in patients. When stably expressed in mammalian muscle cells, the mutations caused steady-state decreases in dystrophin protein levels that are inversely proportional to the tertiary stability and are directly caused by proteasomal degradation. Proteasome inhibitors are able to increase the mutant dystrophin to wild-type levels, establishing the new cell lines as a platform to screen for potential therapeutics personalized for patients with destabilized dystrophin [57]. Using systemic treatment with an osmotic pump, the administration of the proteasomal inhibitor MG-132 effectively rescues the expression levels and plasma membrane localization of dystrophin in skeletal muscle fibers from dystrophin-deficient mdx mice. Proteasomal inhibitors reduced muscle membrane damage, as revealed by vital staining (with Evans blue dye) of the diaphragm and gastrocnemius muscle that was isolated from treated mdx mice; it also ameliorated the histopathological signs of muscular dystrophy, as judged by hematoxylin and eosin staining of muscle biopsies taken from treated mdx mice [58]. Bortezomib was systemically administered in mdx mice over a 2-week period. In this system, bortezomib restored the membrane expression of dystrophin and dystrophin-glycoprotein complex members and improved the dystrophic phenotype [59]. Golden retriever muscular dystrophy (GRMD) is a genetic myopathy that corresponds to DMD in humans. Five GRMD dogs were examined; two were treated with the proteasome inhibitor bortezomib, and three were control dogs. The skeletal tissue from the treated dogs had lower levels of connective tissue deposition and inflammatory cell infiltration that in the control animals as determined by histology, collagen morphometry, and ultrastructural analysis. Significant inhibition of 20S proteasome activity was observed 1 h after bortezomib administration. Proteasome inhibitors may thus improve the appearance of GRMD muscle fibers, lessen connective tissue deposition, and reduce the infiltration of inflammatory cells. In addition, proteasome inhibitors may rescue some dystrophin-associated proteins in the muscle fiber membrane [60].
Congenital muscular dystrophy, caused by mutations in LAMA2 (the gene encoding the laminin α2 chain), is a severe and incapacitating disease for which no therapy is yet available. Proteasome activity is increased in laminin α2 chain-deficient muscle and treatment with the proteasome inhibitor MG-132 reduces the muscle pathology in laminin α2 chain-deficient dy(3 K)/dy(3 K) mice. The proteasome inhibitor bortezomib (currently used for the treatment of relapsed multiple myeloma and mantle cell lymphoma) in dy(3 K)/dy(3 K) mice and in congenital muscular dystrophy type 1A muscle cells can also be effective in the improvement of muscle pathology. Bortezomib improved several histological hallmarks of the disease, partially normalized microRNA expression (miR-1 and miR-133a), and enhanced body weight, locomotion, and survival of the dy(3 K)/dy(3 K) mice. In addition, bortezomib reduced proteasome activity in congenital muscular dystrophy type 1A myoblasts and myotubes [61]. The same group explored the use of bortezomib in dy2J/dy2J animals. However, bortezomib neither improved the histological hallmarks of disease nor increased muscle strength or locomotive activity in dy2J/dy2J mice. It is possible that proteasome inhibition could be useful as a supportive therapy in patients with a complete absence of the laminin α2 chain[62].
Dysferlin is a transmembrane protein implicated in the surface membrane repair of muscle cells. Mutations in dysferlin cause the progressive muscular dystrophies Miyoshi myopathy, LGMD2B, and distal anterior compartment myopathy. A marked reduction in dysferlin in patients harboring missense mutations implies that dysferlin is degraded by the cell’s quality control machinery. One study revealed that dysferlin-deficient human myoblast cultures harbored the common R555W missense allele and a dysferlin-null allele and that control human myoblast cultures harbored either two wild-type or two null alleles. Dysferlin protein and messenger RNA levels, resealing kinetics of laser-induced plasmalemmal wounds, myotube formation, and cellular viability were measured after treatment of the human myoblast cultures with the proteasome inhibitors lactacystin or bortezomib (Velcade). Inhibition of the proteasome increased the levels of R555W missense mutated dysferlin. This salvaged protein was functional as it restored the plasma membrane, resealing in the patient-derived myoblasts, and reversed their deficits in myotube formation. These results raised the possibility that inhibition of the degradation pathway of missense mutated dysferlin could be used as a therapeutic strategy for patients harboring certain dysferlin missense mutations [63]. In the other study, the authors described dysferlinopathy due to a homozygous Arg555Trp mutation in dysferlin in patients treated with the proteasome inhibitor bortezomib and monitored dysferlin expression in monocytes and in skeletal muscle by repeated percutaneous muscle biopsy. Expression of the missense mutated dysferlin in the skeletal muscle and monocytes of the three patients increased markedly, and dysferlin was correctly localized to the sarcolemma of the muscle fibers on histological sections. The salvaged missense mutated dysferlin was determined to be functional in a membrane resealing assay in patient-derived muscle cells treated with three different proteasome inhibitors [64].
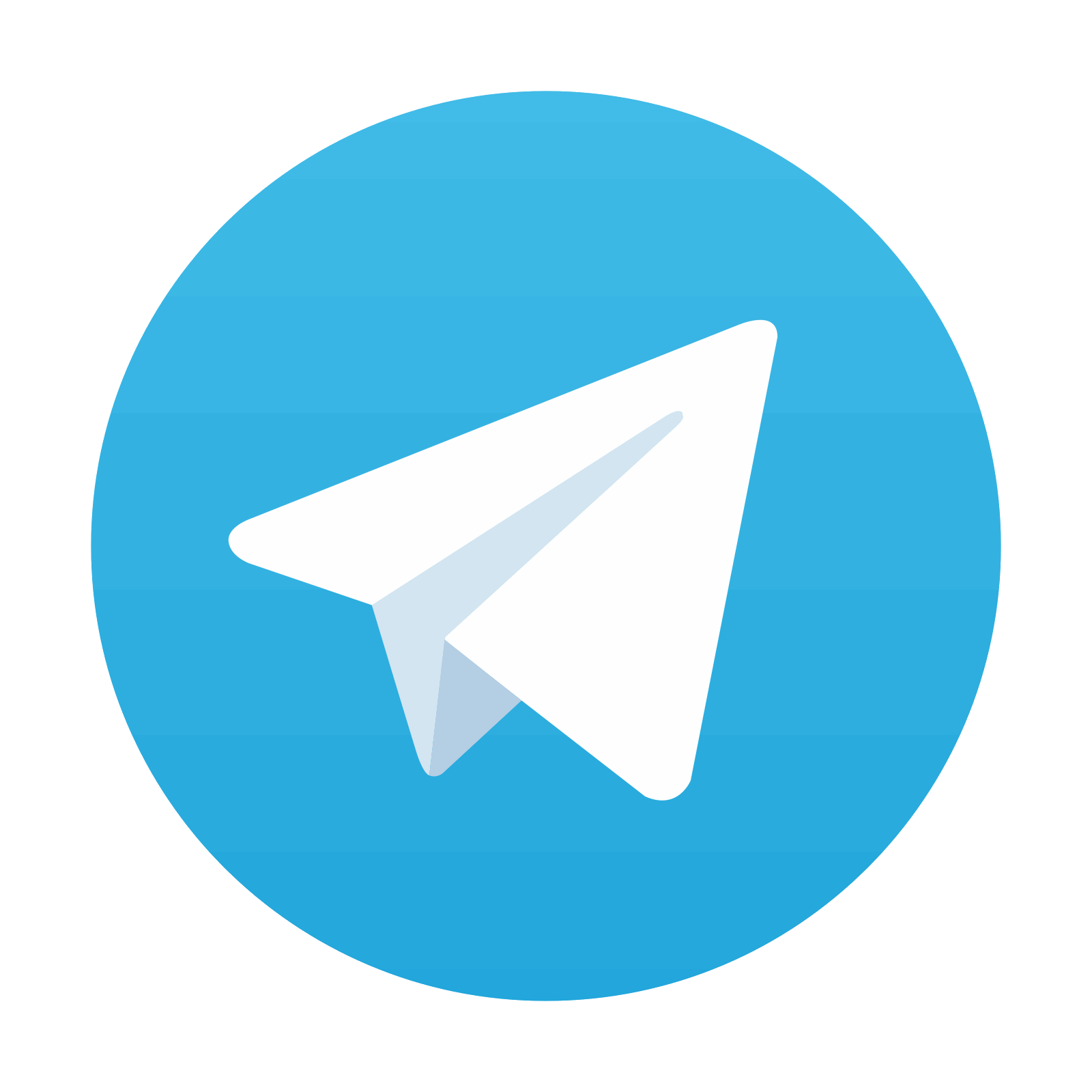
Stay updated, free articles. Join our Telegram channel

Full access? Get Clinical Tree
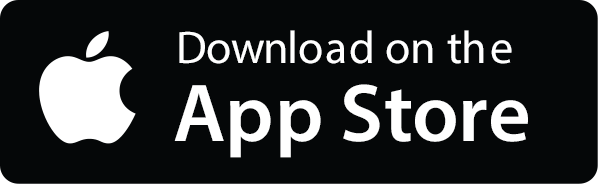
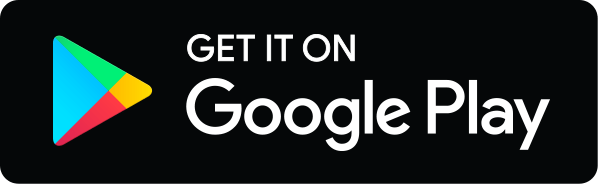