Individuals with cerebral palsy (CP) cannot take a normal activity like walking for granted. CP is the most common pediatric neurologic disorder, with an incidence of 3.6 per 1000 live births. The current trend in the treatment of individuals with CP is to perform a thorough evaluation including a complete patient history from birth to present, a comprehensive physical examination, appropriate radiographs, consultation with other medical specialists, and analysis of gait.
Most of us take walking for granted. Individuals with cerebral palsy (CP) cannot. CP is the most common pediatric neurologic disorder, with an incidence of 3.6 per 1000 live births. There are approximately 9000 newly diagnosed cases annually with a total of 765,000 cases in the United States alone. The pathologic condition that leads to CP produces a central nervous system injury that adversely affects the gait of these individuals. Gait abnormalities are primarily caused by spasticity or abnormal muscle tone, diminished motor control, and impaired balance. Throughout an individual’s growth, gait abnormalities are compounded by progressively developing skeletal deformities, soft tissue contractures of the musculotendinous units and capsular structures, and dynamic deformities caused by abnormal unbalanced muscle forces. As the patient matures, soft tissue contractures, muscle weakness, pain, and compensatory mechanisms result in continued gait abnormalities throughout their lifetime if not treated.
The current trend in the treatment of individuals with CP is to perform a thorough evaluation including a complete patient history from birth to present, a comprehensive physical examination, appropriate radiographs, consultation with other medical specialists, and analysis of gait. Thoughtful consideration of all this information leads to a better understanding of the deformities and pathologies affecting an individual’s characteristic gait at the current stage of development. Once the primary deformities are recognized, the orthopedic surgeon can assimilate this data to formulate a treatment plan with a combination of surgical and nonsurgical interventions. If orthopedic surgery is planned, single-event multilevel surgery (SEMLS) is preferred to performing multiple procedures spread over time. Mercer Rang described this as the birthday syndrome, reflecting a yearly (or every other year) series of hospital admissions, recovery, postoperative therapy, and missed school days. Graham and colleagues have shown improved outcomes using the SEMLS approach, and it is becoming the standard of care for children with CP.
In the twenty-first century, no discussion of gait analysis in individuals with CP would be complete without incorporating the tools and techniques of instrumented gait analysis (IGA). As a diagnostic test, IGA has the unique capability of accurately measuring and providing information that is critical for distinguishing complex gait abnormalities. Knowing the 3-dimensional position of the pelvis, hip, femur, knee, tibia, ankle, and foot at any moment of the gait cycle assists the clinician in recognizing typical pathologic gait patterns. Integrating this information with dynamic electromyography (D-EMG) increases understanding of the pathologic gait patterns and deduces possible treatment options. IGA provides the objective information to assist the orthopedic surgeon in the decision-making process of treatment for these individuals with CP. IGA also measures outcomes during the growing life of the child with CP and helps the clinical provider understand the changing medical needs of the mature adult with the disease.
Information provided by IGA enhances the understanding of gait abnormality by providing real-time objective data that cannot be appreciated visually or measured on a static physical examination. Using advanced technology to improve understanding in this way is similar to localization of a tumor and its surrounding anatomic structures using magnetic resonance imaging rather than relying solely on a 2- or 3-view radiograph when planning a malignant tumor surgical resection or reconstruction procedure.
In addition to the visual gait analysis and static physical examination traditionally used by the orthopedic surgeon to make treatment decisions, the orthopedic surgeon can now take advantage of the latest technology to accurately measure gait pathologies. IGA is not a substitute for clinical judgment and experience, and should be used to enhance clinical decision-making and provide evidence to support a particular treatment plan. The combination of systematic clinical assessment and measurements from IGA provides the basis for an orthopedic surgeon to develop the best treatment plan for a specific child or adult with CP, and represents the strongest outcomes-based approach to address the ambulatory needs of this challenging population.
The central nervous system pathology in CP is extremely variable, but common patterns can be determined. The complexity of the human brain and the interconnections of the various pathways results in complex gait deviations that are a mixture of the individual patterns depending on the exact location of the brain injury or dysfunction. Discussion of all the abnormal gait patterns would be extremely lengthy, so the authors have chosen to present clinical gait patterns that are the most prevalent in individuals with CP. The common gait patterns presented here have been previously described in the literature and include jump knee gait, toe-toe gait pattern in which apparent equinus versus true equinus must be distinguished, crouch knee gait, stiff knee gait, and finally rotational abnormalities affecting gait. Before describing these specific gait patterns in depth, the authors provide some important background information related to classification of gait patterns and also introduce some of the fundamental principles of gait analysis.
Functional classification in CP
As mentioned in the previous section, the walking ability of individuals with CP is as varied as the possible sites of injury to the developing brain, and ranges from the highly functional community ambulators to individuals who are completely nonambulatory and who rely on other assisted methods of mobility. Some have a single-gait abnormality whereas others are more severely affected with multiple deformities, with each deformity interacting with the other resulting in an extremely complex gait pattern. Classification of gait and motor function is challenging because there are many different ways to approach classification, and no 2 individuals with CP are alike. To have a common frame of reference and to promote communication among caregivers, a validated classification scheme known as the Gross Motor Function Classification System (GMFCS) has been used to describe children with CP. Description of GMFCS is well documented in the literature, including the differences associated with age. The GMFCS consists of 5 grades of motor function linked to decreasing levels of ambulatory independence. Level I children walk without assistance in most environments and can run and jump; however, speed, balance, and coordination are limited. Level II children walk in most settings and can climb stairs holding a railing, but may experience difficulty walking long distances or balancing on uneven terrain, and can rarely run or jump. Children at GMFCS level III use handheld assistive devices to walk, and rely on manual wheeled mobility for longer distances. At level IV, walking requires physical assistance; even for short distances and wheeled mobility, using a power wheelchair is required in most settings. At level V, children with CP are nonambulatory and require considerable assistance for most activities, are generally transported in a manual wheelchair, and are limited in their ability to maintain head and trunk postures. Further refinements and details have been established based on the child’s age. Although there are other functional classification schemes, the GMFCS is the most widely used, and is especially important when determining goals of treatment and comparing treatment outcomes for children with CP.
Gait analysis fundamentals
Before describing common gait patterns seen in individuals with CP, it is important to briefly discuss the foundation on which modern clinical gait analysis is based. An understanding of normal gait principles and terminology is basic to being able to communicate abstract concepts related to gait dysfunction. An overview of the gait measurements in common use provides a framework for identifying the causes of an underlying gait problem and a strategy for choosing between different interventions. It is not within the scope of this article to replace the excellent reference material already available on this subject. However, an introduction to the major concepts is warranted to understand the procedures used in gait analysis described in the remainder of this article.
Normal Gait Cycle
The principal goal of locomotion is to propel the body forward. As bipeds, the most natural and efficient way to accomplish this task is to alternate the base of support from one leg to the other while minimizing unnecessary body movements. Typically developing individuals produce a repeatable gait pattern that is both cyclical and symmetric. Because of the cyclic nature of bipedalism, it is useful to consider gait as a repeatable progression with specific events occurring in sequence during each repetition of the cycle. Fig. 1 illustrates one complete gait cycle, or stride, and includes the time periods and temporal events relative to the shaded ipsilateral side and associated with foot-floor contact, which necessarily arise from changing the support limb. Temporal events are specific moments in time that divide the gait cycle into periods of specific duration, and are identified by the skeletal figures along the top of Fig. 1 . Typically, the gait cycle begins when one foot makes contact with the walking surface (initial contact) and ends when that same foot makes contact again. Using such a convention allows a stride to be time normalized, whereby a specific stride location is expressed as a percentage of the total cycle time or stride period. This approach facilitates comparing subjects with different stride lengths, stride periods, and walking speeds on the same scale. The temporal event of foot-off separates the gait cycle into stance and swing periods. In general, stance period occurs from 0% to 60% of the total gait cycle and swing period occurs from 60% to 100%, as seen in Fig. 1 . In individuals with CP, the duration of the initial and final double limb support periods is often different and may vary on a step-to-step basis. The duration of each time period of the gait cycle, the step frequency (cadence), the distance traveled by each foot with each step (step length), and the average walking velocity are collectively known as temporal-spatial parameters, and if measured accurately, they can provide considerable insight into how the child and adult with CP have adapted to their physical limitations while still striving to walk independently.
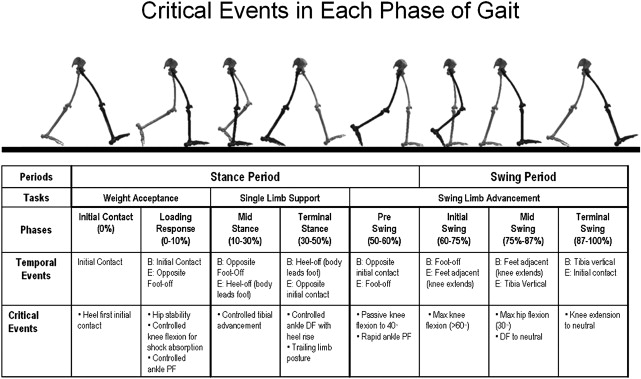
Normal Gait Patterns
The need to alternate one’s base of support and the existence of a repeatable gait cycle forces the segments of the lower limbs to adopt typical patterns of movement at each joint. Graphs of these patterns showing joint angles at different times in the gait cycle are collectively known as gait or motion kinematics. This term refers to the biomechanical description of the limb segments’ motion as if they were a mechanical linkage aligned with the body’s anatomic axes. Fig. 2 shows normal kinematics for a group of typically developing 13-year-old children for the trunk, pelvis, hip, knee, and ankle in the sagittal, coronal, and transverse planes. For lower extremity kinematics, the pelvis marks the top of the kinematic chain and is referred to a fixed coordinate system, while each joint down the chain is (by convention) the angle of the distal segment relative to the more proximal segment. At the end of the chain, foot progression angle is again referred to the fixed coordinate system and is equivalent to the amount of toe-out or toe-in relative to the line of progression. The trunk can be referred either to the pelvis (see Fig. 2 ) or to the laboratory-fixed coordination system. Once oriented to the format of these kinematic graphs, common patterns of joint motion during normal gait are easily recognized. The largest and most easily observed angular displacements occur in the sagittal plane, whereas smaller, less obvious movements are seen in the coronal and transverse plane. The sagittal plane graph at the hip shows a single wave from flexion to extension during stance period and back to full flexion again in midswing, corresponding to the reciprocal motion at the hip necessary for limb advancement. At the knee joint, in the sagittal plane, the first wave is a smaller knee flexion peak occurring during weight acceptance to account for shock absorption at the knee. The second wave is a larger knee flexion peak occurring in midswing to functionally shorten the leg during limb advancement to allow the foot to clear the walking surface. The sagittal plane ankle curve displays the “3 rockers” described by Perry. The first (heel rocker) is associated with controlled plantar flexion (PF) to lower the foot to the floor during weight acceptance. The second (ankle rocker) is associated with controlled tibial advancement and increasing dorsiflexion during mid and terminal stance as the body center of mass advances over the stationary foot. The third (toe rocker) is associated with rapid ankle PF for propulsion during the second double support phase (preswing) and is the source of one of the two primary power generators during normal gait. The ankle sagittal plane curve shows the requirement for dorsiflexion to be near neutral for successful foot clearance during swing period. The remaining planes of motion described in the normal kinematic graphs also show characteristic movements that have been well documented elsewhere. Having a quantitative description of the normal, 3-dimensional orientation of the pelvis and foot and the angular displacement of the joints of the lower limbs during a typical gait cycle provides a precise reference to compare with measurements obtained from the child and adult with CP. This comparison allows for detection of subtle deviations from normal, and provides a framework for a systematic analysis strategy that is the basis for describing many techniques and observations in the remainder of this article.
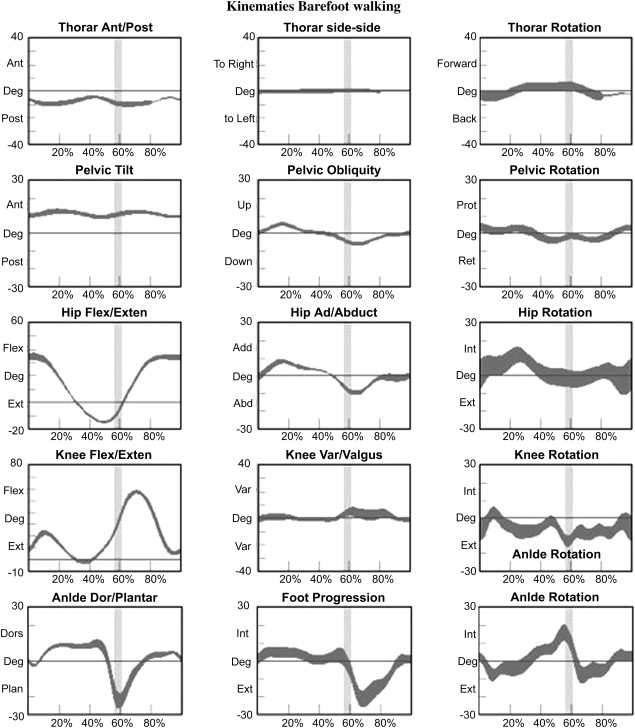
In addition to temporal-spatial parameters and 3-dimensional kinematics, there are also quantitative references for the following during normal walking of typically developing individuals: the forces of motion (kinetics), the timing and activity level of the muscles (D-EMG), foot plantar pressure, oxygen consumption, and more global measures of overall gait performance such as the gait deviation index. The authors introduce some of these measures because they are needed in the remaining sections to clarify the gait patterns commonly seen in individuals with CP and how IGA can provide objective evidence of gait function to plan an appropriate course of treatment.
Functional gait patterns in CP
Every individual with CP presents a unique combination of impairment severity, compensatory movements, and overall ambulatory ability. Experienced clinicians who regularly treat CP attest that most patients display one or more distinct walking patterns. Describing and classifying the gait patterns seen in this population provides a common starting point for further assessment and a connection to other patients with similar gait dysfunction at their current developmental stage. The ability of IGA to precisely measure gait function at the individual joint level lends itself to identifying these functional patterns, and gives much of the information needed to select appropriate treatments. The remaining sections describe the primary gait patterns seen in individuals with CP and a description of how gait analysis can be used by the orthopedist to manage their treatment.
Jump knee gait
Jump knee gait is defined primarily from sagittal plane kinematics, with dynamic changes easily identified using IGA. Jump knee gait is characterized by increased hip and knee flexion, with slight dorsiflexion at initial contact followed by rapid knee extension (KE) and ankle PF during loading response and midstance. These characteristic dynamic findings seen at the ankle begin with forefoot initial contact followed by stretch on the ankle plantar flexor gastrocnemius-soleus complex musculature. On achieving ultimate stretch, the plantar flexors contract rapidly as a stretch response to the commonly spastic triceps surae muscles. The rapid PF of the ankle causes heel rise and prevents the development of a second rocker at the ankle. The resultant proximal joint reactions include rapid knee and hip extension as the plantar flexors contract, giving the clinical appearance of the patient jumping up from one foot to the next. This combination of ankle PF and KE induced by foot position and/or calf spasticity is driven by a shift in the external ground reaction force (GRF) from behind the knee at initial contact and early loading response to a position anterior to the knee joint axis in midstance ( Fig. 3 ). This shift in the GRF produces a force couple or external KE joint moment, commonly called the PF and KE couple, which prevents knee joint collapse without active quadriceps activity.
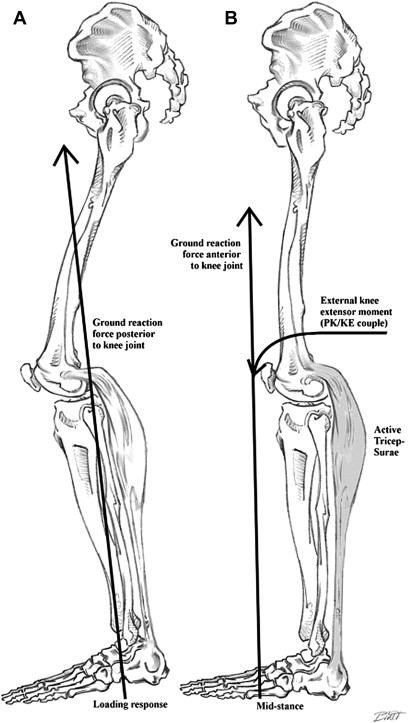
During swing period there is increased knee flexion in mid to terminal swing, leading to the increased flexion seen during initial contact and loading response. This occurrence is believed to be caused by near normal quadriceps and gastrocnemius-soleus strength with some spasticity of the hamstring muscles and hip flexors. Other kinematic changes that are seen with jump knee gait include increased hip flexion throughout the gait cycle in the sagittal plane and increased hip adduction in the frontal plane due to hip adductor contracture and spasticity.
Jump knee gait can be identified in patients with spastic diplegia, hemiplegia, or quadriplegia. It is most often seen in patients with diplegia and hemiplegia and in patients with GMFCS levels II and III. Jump knee gait is not seen in GMFCS level V patients and is rare in patients classified as level IV. It is most commonly seen in younger patients learning to ambulate, and frequently changes as the patient gains weight and gait matures.
Equinus and the important distinction between true and apparent
Equinus deformity is common in individuals with CP and is characterized by excessive PF of the calcaneus relative to the tibia. The simplest form includes normal bony alignment of the midfoot and forefoot, leading to an overall plantar flexed position of the entire foot. More complex deformities such as equinoplanovalgus and equinocavovarus occur when excessive PF of the hindfoot is accompanied by an abnormal midfoot and forefoot alignment. In children with CP, equinus generally results from a dynamic imbalance between the muscles of the lower leg due to spasticity, poor motor control, and/or impaired balance function. This imbalance disrupts the normal ankle motion during walking, often producing a forefoot initial contact that persists throughout the stance period and may result in the emergence of a toe-toe gait.
Although the gastrocnemius and soleus are obvious contributors to this deformity, care should be taken before making either of these plantar flexors the target of surgical intervention. Specifically, special attention should be paid to the subtle difference between 2 distinct gait patterns that can produce the appearance of equinus and toe-toe gait but require completely different interventions. Rodda and Graham have referred to these 2 patterns as true equinus and apparent equinus. In a true equinus gait pattern, calf spasticity or fixed ankle equinus contracture is dominant, resulting in excess ankle PF with hips and knees either extended or displaying relatively little deviation from normal over the gait cycle. With equinus, PF and KE coupling similar to that in the mid to terminal stance phases of jump knee gait is seen, and may result in knee recurvatum with increased hamstring length and gradual stretch of the posterior knee capsule. This pattern is most often seen in younger children with diplegia soon after they begin to walk, and rarely persists throughout childhood unless a fixed ankle contracture develops.
By contrast, apparent equinus is dominated by increased hip and knee flexion throughout the stance period with a relatively normal range of motion at the ankle. Observation reveals that the child is still walking on his or her toes and appears to be in equinus, but in fact the forefoot’s initial contact is the result of the significant knee flexion that is present at the moment of weight acceptance. As previously discussed, this pattern is characteristic of the early stance phase of jump knee gait but can also evolve from a previous true equinus that may have been present when the child was younger. As these children grow older and heavier, they lose much of their original equinus because of weakened plantar flexors, development of foot deformity, and/or the development of an ineffective PF and KE couple, and now have a pattern dominated by increased hip and knee flexion, especially at terminal swing and the initial double support period. For the child with apparent equinus, any procedure that further weakens the calf and allows the tibia to advance earlier and more rapidly during loading response and midstance threatens to produce a crouch gait pattern that inevitably leads to significant functional impairment. For this reason, Goldstein and Harper have stated that failure to recognize apparent equinus is the most common error in observational gait analysis, and is of more than purely academic importance.
While it is sometimes challenging to distinguish between true equinus and apparent equinus using simple observation alone, using techniques from IGA make this task relatively straightforward. Fig. 4 B and Fig. 5 B show a still image of the right side midstance bilateral knee and ankle sagittal plane kinematics for 2 subjects with a toe-toe gait pattern. The subject in Fig. 4 A has apparent equinus and the subject in Fig. 5 A has true equinus. Comparing the ankle curves between the 2 subjects clearly shows that the subject with true equinus has excess PF starting in midstance and continuing for the rest of the gait cycle, whereas the subject with apparent equinus has a near normal ankle range of motion with an average value across the cycle of approximately zero. Notice that both ankle curves do not match the normal reference, because both subjects have a toe-toe gait pattern. Comparing the knee kinematic curves, the subject with true equinus has a more normal knee range of motion with full KE at terminal stance, whereas the subject with apparent equinus has increased knee flexion throughout the stance period, most prominent on the right side. This comparison clearly illustrates the quantitative differences between these 2 similar gait patterns using just the sagittal plane kinematic curves, and demonstrates how IGA can facilitate identification of what Goldstein has described as the most common error in observational gait analysis.
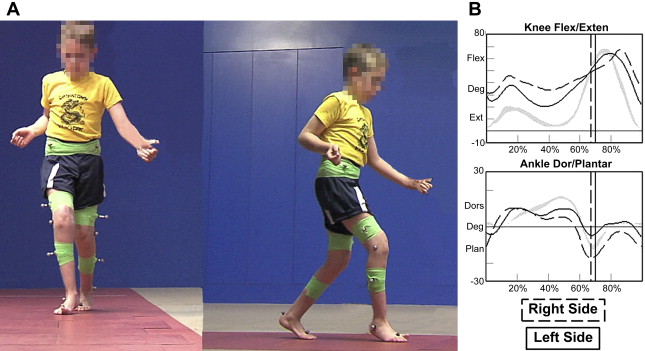
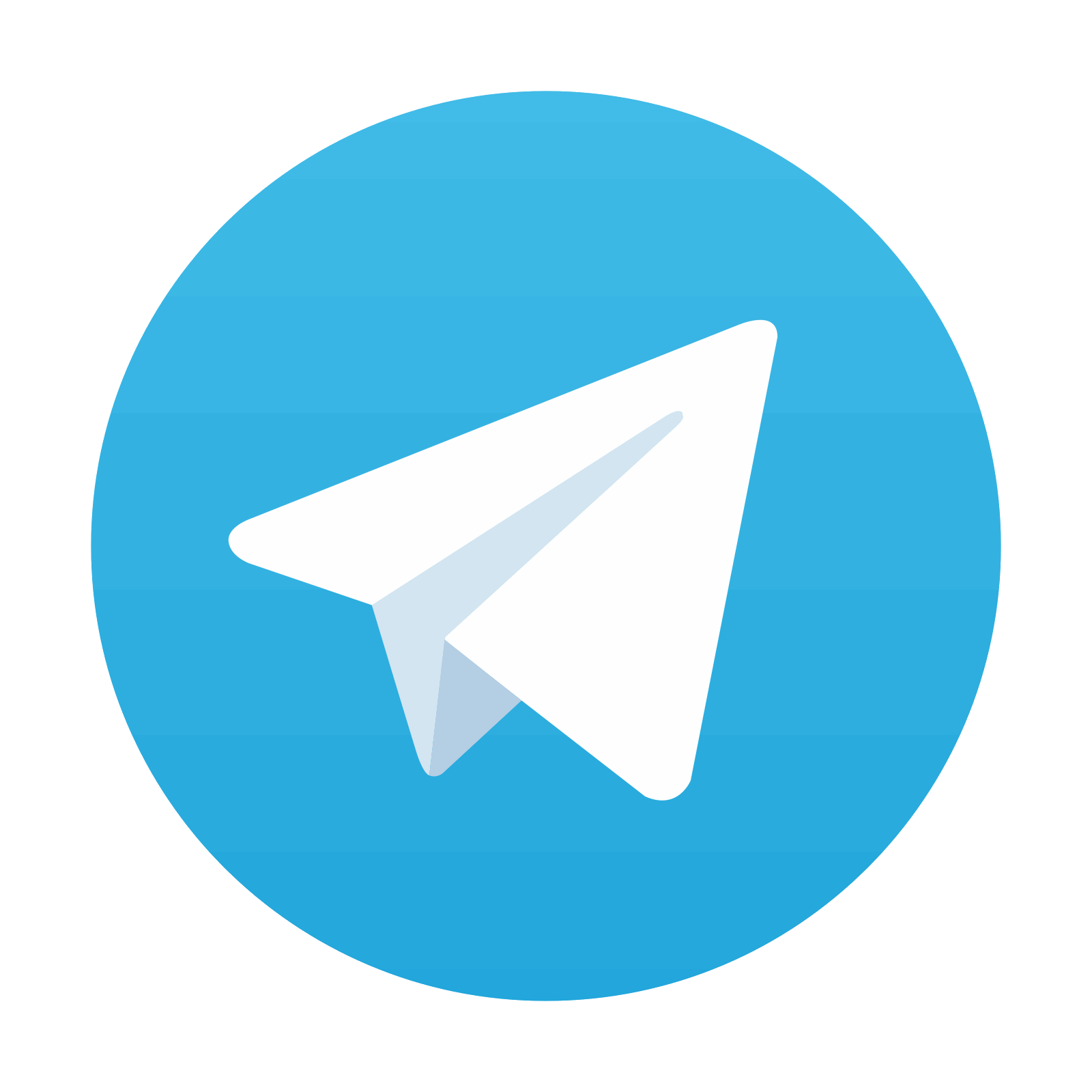
Stay updated, free articles. Join our Telegram channel

Full access? Get Clinical Tree
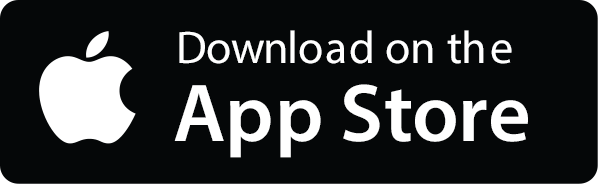
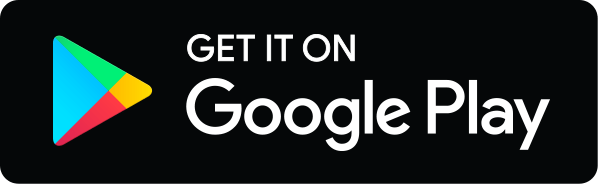
