Fig. 1.1
The phases of tissue healing (https://en.wikipedia.org/wiki/File:Wound_healing_phases.png#References)
Altered protease activity is a biomarker of incomplete healing. In the phases of normal wound healing, the production and activity of proteases are tightly regulated, but this regulation appears to be disrupted in chronic injuries. Levels of various MMPs and serine proteases are markedly increased in fluids from chronic wounds. Levels of MMP-1 (collagenase), MMP-2 (gelatinase A), and MMP-9 (gelatinase B) have been shown to be elevated in fluid derived from pressure ulcers [17]. During granulation tissue formation in chronic ulcers, levels of MMPs have been shown to be decreased, while levels of their inhibitors, TIMPs, have been found to be increased [18]. This may explain the mechanism of impaired cellular responses and extracellular matrix reorganization observed in chronic wounds.
Similarly, anabolic and catabolic imbalance in chondrocyte, subchondral bone, and extracellular matrix is seen in osteoarthritis (OA). Degradation and destruction of collagen II caused by MMP-13 are considered a key factor in the development of OA [19]. Indeed, biomarkers indicative of type II collagen (Col II) synthesis such as carboxy peptide of Col II procollagen (CP-II), or cleaved products C2C and CTX-II, are increasingly being expressed in ratios of each other and show great promise in detecting early osteoarthritis [20].
Elevated levels of the pro-inflammatory cytokines TNF-α, interleukin-1 β (IL-1β), and TGF-β1 are found in chronic wounds [21]. Levels of these cytokines decrease as the wound heals [22]. Macrophage activation is suppressed in chronic wounds leading to an impaired inflammatory response [23]. Polymorphonuclear (PMN) cells produce more reactive oxygen species in chronic wounds [24]. The production of catabolic factors involved in both cartilage degradation and nociceptive stimulation includes IL-1, IL-6, TNF-α, PGE2, FGF-2, and PKCδ [25].
Fibroblasts in chronic ulcers may not be capable of responding to growth factors such as PDGF and TGF [26], and fibroblasts isolated from chronic wounds demonstrate reduced motility or migration [27].
Free radicals are short-lived, reactive chemicals containing one or more unpaired electrons. They are classified as reactive oxygen species (ROS), reactive nitrogen species (RNS), or reactive chlorine species (RCS). In low levels, they influence cell differentiation and migration, neurotransmitters, leukocyte adhesion, thrombosis, angiogenesis, and vascular tone. But excess production can induce cellular damage by oxidizing cell components and molecules such as lipids, proteins, or DNA [28].
Free radical production results endogenously from reactions of organic compounds with oxygen or neutrophils stimulated by exposure to microbes. They are produced in the body during phagocytosis, in prostaglandin synthesis, and in the cytochrome P450 system, respiratory chain reactions, and aerobic respiration in the mitochondria [29].
The superoxide (O2 −) radical is one of the main reactive oxygen species in the cell deployed by the immune system to kill invading microorganisms, but is also implicated in cellular damage and aging. The superoxide dismutase enzyme catalyzes the reaction of the superoxide (O2 −) radical to form either molecular oxygen (O2) or hydrogen peroxide (H2O2) efficiently reducing oxidative stress. Modern industrialized society produces numerous exogenous sources of free radicals which include ionizing radiation, lead, pesticides, cadmium, alcohol, cigarette smoke, ultraviolet light, asbestos, automobile exhaust, microwave radiation, and air pollution from fires or volcanic activity [30, 31]. Oxygen-derived free radical reactions have been associated with several diseases including Alzheimer’s disease, Parkinson’s disease, multiple sclerosis, amyotrophic lateral sclerosis, atherosclerosis, hypertension, ischemic heart disease, asthma, chronic obstructive pulmonary disease, rheumatoid arthritis, renal failure, and lung, breast, and hematologic cancers [32, 33].
1.4 Factors That Contribute to Impaired Healing
1.4.1 Obesity
Excess body weight is the primary modifiable factor influencing the development of knee osteoarthritis [34, 35]. Knee pain from osteoarthritis correlates strongly with body mass index (BMI) in studies performed all over the world [36–44]. Knee osteoarthritis occurs about seven times more frequently in obese individuals than in nonobese individuals [45]. Garver et al. found that individuals in the highest BMI class had significantly more pain and poorer function than those with lower BMIs, even after controlling for radiographic osteoarthritis severity [46].
Increasing low-level activity, especially among inactive individuals, alleviates knee pain and reduces the risk of disability from knee osteoarthritis [34]. Randomized controlled trials show that exercise is safe and effective for overweight and obese adults with osteoarthritis [47].
Messier et al. [48] studied the effects of knee pain and body weight reduction by diet, with or without exercise in 399 older adults (>55 years) with knee osteoarthritis and body mass index of 27–41. After 18 months, lean weight loss was 11.4% for the diet/exercise group, 9.5% for the diet-alone group, and 2% for the exercise group. Pain levels and IL-6 serum concentrations were lower in the diet/exercise group when compared with the diet-alone or exercise-alone groups. The diet/exercise group also had better physical health-related quality of life scores and better function scores. Regardless of which etiological factors have the greatest effect on knee osteoarthritis, a reduction in excess body weight is the primary risk factor that can be modified to reduce knee osteoarthritis pain [34, 48].
1.4.2 Drugs
More than one billion people worldwide smoke tobacco products. Smoking is the leading cause of preventable death and causes 480,000 deaths each year in the United States alone [49]. The US Department of Health and Human Services reports that cigarettes contain at least 250 chemicals known to be harmful in humans, including hydrogen cyanide, carbon monoxide, and ammonia. Among the known harmful chemicals, at least 69 can cause cancer [49, 50].
Nicotine, present in all tobacco products, is addictive and reduces cutaneous blood flow by vasoconstriction, stimulates release of proteases that may accelerate tissue destruction, suppresses the immune response, and leads to an increased risk of infection [51]. Almost every clinical study on the effects of smoking in patients undergoing orthopedic surgery for cartilage or ligament injuries demonstrates negative outcomes in patients who smoke [52]. In patients undergoing rotator cuff repair, there is decreased tendon repair quality, decreased biomechanics, poorer clinical outcomes, and impaired healing of small to medium tears as assessed by magnetic resonance imaging in patients who smoke [53]. In cases of fracture healing, smoking tobacco is a contributory factor in delayed unions and prolonged healing times [54].
Smoking cessation is the single most effective intervention to improve healing. Heart rate, blood pressure, carbon monoxide levels, oxygenation, and blood circulation can normalize within a few weeks of quitting. Smoking cessation restores the tissue microenvironment rapidly and the inflammatory cellular functions within 4 weeks, but the proliferative response remains impaired [51]. Smoking cessation just 4 weeks prior to surgery can significantly reduce complication rates [55]. Long-term effects of quitting include decreased risk of cancer, heart disease, and pulmonary disease [56]. Assistance for patients who choose to quit smoking can be found at the National Cancer Institute’s quit line, 1-800-QUIT-NOW (www.smokefree.gov).
Alcohol is one of the most widely used drugs in the world. The Substance Abuse and Mental Health Services Administration reported rates of alcoholism are as high as 10% in the North American population. Alcohol is a central nervous system depressant with wide-ranging and detrimental systemic effects [57]. Increased insulin resistance and higher serum sugar levels with alcohol consumption impair healing [58].
Chronic alcohol abuse is associated with a high risk of malnutrition which results in decreased inflammatory and immune responses to tissue injury, decreased fibroblast migration, angiogenesis, and decreased type I collagen production [59]. Alcohol also reduces bone quality and delays fracture repairs [60, 61].
Glucocorticosteroids, including prednisone and dexamethasone, have been used to treat inflammation in rheumatoid and osteoarthritis since 1951. However, long-term use can delay healing by interfering with inflammation, fibroblast proliferation, collagen synthesis and degradation, deposition of connective tissue ground substances, angiogenesis, wound contraction, and re-epithelialization [62]. Subcutaneous injection of dexamethasone strongly interferes with both the synthesis and degradation of type I collagen and type III collagen [63]. Animal studies show a 30% reduction in wound tensile strength with perioperative corticosteroids at 15–40 mg/kg/day [64].
Local administration of glucocorticoid has significant negative effects on tendon cells in vitro, including reduced cell viability, cell proliferation, and collagen synthesis. The mechanical properties of tendon are also significantly reduced [65]. Corticosteroid injections inhibit collagen tendon repair and are a predictor of future tendon tear [66].
Long-term use or high-dose intra-articular administration of glucocorticosteroids may also induce apoptosis in chondrocytes impairing cartilage homeostasis [67]. Increased chondrocyte autophagy has been described as an adaptive response to protect chondrocytes from short-term glucocorticosteroid exposure, whereas prolonged exposure decreases autophagy and increases apoptosis in vitro [67]. Yet, in vitro dexamethasone has failed to induce chondrotoxicity in low-dose exposures [68].
Clinical trials in humans do not demonstrate adverse effects in patients receiving a single-dose steroid injection following surgery [69]. And a review of the human literature found that high-dose corticosteroid administration for less than 10 days has no clinically important effect on wound healing, but in patients taking chronic corticosteroids for at least 30 days before surgery, wound complication rates are increased up to five times [64]. Further studies are required to determine the effects of a single-dose steroid injection versus prolonged steroid use on healing in patients receiving regenerative medicine therapies.
Nonsteroidal anti-inflammatory drugs (NSAIDs) are commonly used for their analgesic and anti-inflammatory effects. Cyclooxygenases (COX-1 and COX-2) catalyze the conversion of arachidonic acid to prostaglandins which plays a significant role in normal bone healing, osteoclastic activity, bone formation, and angiogenesis [70]. These drugs have been hypothesized to affect the healing process of bone fractures and orthopedic surgical sites. Nonsteroidal anti-inflammatory drugs (NSAIDs) have been shown to have a depressant effect on wound healing while simultaneously decreasing the granulocytic inflammatory reaction [71]. NSAIDs have an antiproliferative effect on blood vessels and skin, thereby delaying wound healing rates [72].
The role of NSAIDs in orthopedics remains controversial because of unclear effects on healing [73]. Delayed fracture healing and spinal fusion inhibition have been described in association with traditional NSAID use [74–77]. Several experimental models of bone, ligament, and tendon repair have assessed the effects of these drugs in animals with variable outcomes [78]. Celecoxib, a COX-2-specific NSAID, and indomethacin significantly inhibit tendon-to-bone healing in a rat model [79]. However, in a recent review of the literature, the short-term use of low-dose NSAIDs and COX-2 inhibitors did not appear to have a detrimental effect following soft tissue injuries [73]. Most studies on the effects of NSAIDs on healing have been completed in animal models making it difficult to translate these results to the clinical setting.
1.5 Factors That Improve Natural Healing
1.5.1 Sleep
Perhaps no activity influences optimal health more profoundly than sleep. In the United States, the average sleep duration has decreased steadily from 9 h per night in 1910 to 6.8 h per night [80]. Only 56% of people surveyed believe they get enough sleep each night [80]. The situation is far worse for patients suffering with orthopedic injuries. More than 70% of patients with lower back pain suffer from sleep disturbance when measured both subjectively and objectively [81]. Sasaki et al. measured the prevalence of sleep disturbance in patients with knee osteoarthritis finding a prevalence of 3.6%, 6.9%, 19.4%, 32.7%, and 75.0% in Kellgren–Lawrence grades 0, 1, 2, 3, and 4, respectively [82].
Sleep disruption is known to cause a range of adverse physiological and psychological effects. Poor sleep is associated with decreased cognitive performance, altered carbohydrate metabolism, and impaired endocrine function [83]. Decreased carbohydrate tolerance and increased sympathetic tone are also associated with sleep deprivation [83]. Over the long term, these effects can lead to serious health conditions such as depression, obesity, type 2 diabetes, hypertension, and coronary artery disease [84, 85].
Sleep deprivation leads to an imbalance between catabolic and anabolic hormone activity [86–88]. In humans, reduced sleep and obstructive sleep apnea adversely impact metabolic and hormonal pathways [83, 89, 90]. Poor sleep also enhances catabolic processes and is associated with increased evening cortisol levels [91, 92]. Chronic insomnia is associated with higher hypothalamic pituitary axis (HPA) activity leading to elevated cortisol and adrenocorticotropic hormone (ACTH) levels [83, 89, 93, 94]. Sleep and exercise may interact with each other by endocrine and inflammatory pathways associated with sarcopenia [95]. Exercise training in older adults possibly interacts with sleep by reducing inflammatory biomarkers, such as IL-6 and TNF-α [96].
Melatonin is a neurohormone secreted by the pineal gland, and it is well known for inducing and regulating sleep. Exposure to light suppresses melatonin synthesis. The primary use of melatonin as a supplement is to normalize abnormal sleep patterns. Exogenous melatonin is one of the most frequently used nonprescription sleep aids due to its role in the internal timing of biological rhythms, including promotion and regulation of sleep [97]. Melatonin may reduce the time it takes for people to fall asleep and resets the body’s sleep–wake cycle [98]. Melatonin also increases total sleep time in individuals suffering from sleep restriction or altered sleep schedules and relieves daytime fatigue associated with jet lag [99].
The National Sleep Foundation recommended dose of melatonin is 0.3–5.0 mg taken 60 min before bedtime. This supplement should only be taken as needed for occasional insomnia. Start with the lowest dose possible because synthetic melatonin is not regulated by the FDA and listed doses may not be accurate. Potential side effects include daytime drowsiness, depressed mood, irritability, abdominal pain, dizziness, and headache.
Although melatonin has important effects on sleep physiology, it may also play a role in the protection against trauma-induced cartilage degeneration. Hong et al. investigated the effects of melatonin combined with treadmill exercise on cartilage damage [100]. Intra-articular injection of collagenase in rats caused cartilage fibrillation and abnormal chondrocytes clustering and downregulated TGF-β1 mRNA levels. However, these changes were rarely seen in the melatonin alone or melatonin combined with exercise subjects. Collagenase injection caused significant serum TNF-α and IL-6 level upregulation. Melatonin, with or without exercise, significantly decreased the expression of catabolic mediators and cellular death markers with lower mineralization indicating that melatonin with exercise may have both preventive and synergistic effects in reducing cartilage degeneration.
Magnesium is an essential mineral that plays a key role in the regulation of central nervous system excitability. Magnesium deficiency is associated with poor quality sleep, increased sleep latency, predominance of light slow-wave sleep, diminution of deep slow-wave sleep, and a reduction in rapid eye movement (REM) sleep [101]. Shorter sleep time is associated with higher levels of the inflammatory markers interleukin 6 (IL-6) and C-reactive protein [102]. The highest elevations are found in patients with sleep apnea and high body mass index (BMI) [103]. Magnesium supplementation has demonstrated improved sleep quality in adults older than 51 years of age [104].
The NIH recommended daily allowance (RDA) of magnesium is 420 and 320 mg for men and women older than 50 years of age, respectively. It is estimated that 38% of adults have daily magnesium intakes less than the recommended allowance [105]. Magnesium deficiency is considered when serum magnesium concentrations are lower than 0.75 mmol/L. Patients with malabsorption (Crohn’s, celiac disease, history of intestine surgery), type 2 diabetes, chronic alcoholism, and renal disease or those taking diuretics are at increased risk of magnesium deficiency. Green leafy vegetables, nuts, legumes, and whole grains are rich sources of magnesium. Dietary supplementation with magnesium glycinate 300 mg is preferred to other forms of supplemental magnesium due to increased bioavailability and reduced risk of side effects. Magnesium overdose can result in diarrhea, hypotension, lethargy, confusion, and cardiac rhythm abnormalities.
Sleep hygiene education is a safe and cost-effective method for managing sleep disturbance. Patients suffering from sleep disturbance should be given the following advices:
- 1.
Get 10–15 min of morning sunlight each day. The circadian system needs bright light to reset itself. More sunlight exposure is required as you age.
- 2.
Get a daily dose of bright sun exposure during the day to optimize melatonin production.
- 3.
Avoid drinking caffeinated beverages several hours before bedtime. Some people do not metabolize caffeine properly and should avoid caffeine altogether.
- 4.
Take a hot bath less than 3 h before bedtime. The core body temperature drops after a hot bath and signals that it is time to sleep.
- 5.
After a hot bath, spend 10 min stretching or massaging muscles to minimize the likelihood of pain that triggers awakenings.
- 6.
Avoid watching TV or using a computer at least an hour before going to bed. These devices emit blue light reducing the production of melatonin and can disrupt circadian rhythms, affecting cell cycle regulation and metabolism. In addition, modern television rarely offers content conducive to a good night’s sleep.
- 7.
Install low-wattage yellow, orange, or red light bulbs for a light source to navigate at night. These wavelengths do not impair melatonin production to the same degree as blue light.
- 8.
Sleep in complete darkness, cover the windows with drapes, or wear an eye mask. Light controls melatonin production in the pineal gland, and even a small amount of light from a clock radio can interfere with sleep.
- 9.
Maintain bedroom temperature to less than 70 °F (21 °C). The optimal room temperature for sleep is between 60 and 68 °F (15.5–20 °C).
- 10.
Move all electronics away from the bed at night. Electromagnetic fields found in electronic devices such as a computer or router can disrupt the pineal gland and its melatonin production.
- 11.
Avoid using loud alarm clocks and, if possible, do not use them at all.
- 12.
If you find that your mind is racing, consider writing your ideas down on a notepad or even spend a few minutes attending to the issue of concern.
1.5.2 Circadian Rhythms
These are essentially endogenous physiological oscillations occurring at 24-h interval. These rhythms are maintained by circadian clocks which are located in every functional cell type, including the immune system. The immune system and its regulation play a vital role in tissue healing, and disorders of immune system have a direct effect on efficacy of tissue healing. Most immune cells through their expression of circadian clock genes display profound impacts on cellular functions, including a daily rhythm in the synthesis and release of cytokines, chemokines, and cytolytic factors [106].
Alteration of circadian rhythms through clock gene mutation in many animal studies has shown altered immune response states [106]. A rat model study has shown that rats with circadian rhythm disorder showed poor collagen synthesis and impaired effect of interleukin (IL)-1, IL-6, transforming growth factor α, and stress hormones on fibroblasts [107]. A recent molecular level study has also shown that cardiac neutrophil oscillations have a direct effect on myocardial infarct size and healing [108]. Melatonin, a key hormone promoting circadian rhythm, has shown to have a direct effect on skin wound healing in a mouse model. This mouse model study found transplantation of umbilical cord blood (UCB)-MSCs pretreated with melatonin-enhanced wound closure, granulation, and re-epithelialization at mouse skin wound sites [109].
In the present era, circadian rhythm disorder influences healing in people who travel great distances across the globe and in athletes or workers who are subjected to work night shifts. Further research in the area of human chronobiology can help in better understanding of the role of circadian rhythm and its influence on tissue healing in humans.
1.5.3 Nutrition
The development of various chronic and degenerative diseases, such as cancer [110], heart disease [111], and neuronal degeneration [112, 113], may be attributed to oxidative stress. Oxidative stress has also been implicated in the process of aging [114] and in the pathogenesis of osteoarthritis [115]. Oxidative stress induces telomere genomic instability, replicative senescence, and dysfunction of chondrocytes in human osteoarthritic cartilage. [115]. In tissue cultures of cartilage explants, nitrotyrosine staining, chondrocyte telomere length, and glycosaminoglycans (GAG) remaining in the cartilage tissue were decreased in cartilage exposed to reactive oxygen species (ROS). However, in cartilage treated with the anti-oxidative, ascorbic acid, a tendency to maintain chondrocyte telomere length and proteoglycan was seen in the cartilage explants, suggesting that oxidative stress induces chondrocyte telomere instability and catabolic changes in cartilage matrix structure and composition [115].
Increased reactive oxygen species (ROS) inhibit mesenchymal stem cell (MSC) proliferation, increase senescence, enhance adipogenic differentiation, reduce osteogenic differentiation, and inhibit MSC immunomodulation [116, 117]. Furthermore, oxidative stress reduces their ex vivo expansion capabilities [117]. MSCs may have low innate antioxidant activity and are more sensitive to oxidative stress compared to more differentiated cell types [118, 119].
Antioxidants are substances capable of scavenging free radicals and preventing cellular damage. Increased consumption of whole foods such as fruits and vegetables containing natural antioxidants may offer health benefits [120, 121].
Nonenzymatic antioxidants are classified as metabolic or nutrient antioxidants. Metabolic antioxidants are produced by endogenous cellular metabolism and include coenzyme Q10, melatonin, uric acid, bilirubin, l-arginine, transferrin, and glutathione.
Nutrient antioxidants are provided in the diet and include selenium, manganese, zinc, flavonoids, vitamins E and C, and omega-3 and omega-6 fatty acids. The oxygen radical absorbance capacity (ORAC) assay measures the degree of inhibition of peroxy-radical-induced oxidation by nutrient antioxidants. A review of the antioxidant activity in 326 selected foods reveals high activity in many fruits, vegetables, herbs, and spices [122]. Foods with higher ORAC values include blueberries, raspberries, strawberries, cranberries, blackberries, apples, pears, citrus fruits, gooseberries, figs, onions, broccoli, artichokes, eggplant, asparagus, garlic, thyme, oregano, basil, peppermint, black pepper, ginger, cinnamon, curry powder, cumin, paprika, peanuts, almonds, pecans, walnuts, pistachios, beans, and peas.
Nutritional deficiencies have been long recognized as an indicator of poor health. Patients with decreased albumin or zinc levels demonstrate delayed healing and greater incidence of complications following orthopedic surgery. Greene reported that patients with albumin level less than 3.5 g/dL showed a sevenfold increase in the risk of major wound complications following hip or knee arthroplasty [123]. Low albumin levels may be due to kidney or liver disease, poor nutrient absorption, or low protein diets.
Decreased protein intake can negatively impact healing due to decreased collagen production, angiogenesis, and fibroblast proliferation. The essential amino acids histidine, isoleucine, leucine, lysine, methionine, phenylalanine, tyrosine, threonine, tryptophan, and valine are not produced in the body and are only supplied by the diet. They serve as enzymes, hormones, cytokines, growth factors, and components of antibodies. Inadequate protein intake impedes both tissue maintenance and healing. Insufficient protein intake can be assessed utilizing serum albumin, pre-albumin, or total lymphocyte count. The recommended daily allowance for protein in healthy adults more than 20 years of age is 80 mg per kg of body weight. Malnourished patients may require up to 1.5 g per kg of body weight [59]. Excellent sources of digestible protein include eggs, milk, cheese, meat, fish, rice, wheat, oatmeal, peanut butter, and beans.
Zorrilla demonstrated that patients with zinc levels less than 95 μg/dL had almost 12-fold increased risk for wound complications in patients undergoing hip hemiarthroplasty for fracture [124]. The daily recommended dose for zinc is 11 and 8 mg/day in men and women older than 50 years, respectively. Good sources of zinc include meats, seafood, milk, cheese, nuts, legumes, and whole grains.
The active form of vitamin D, 1,25-dihydroxy-vitamin D3, is essential for normal bone growth, maintenance, and repair. It is probably essential for the health of most nonskeletal tissues as well as the vitamin D receptor has been identified on almost every cell in the body. Vitamin D supplementation also significantly inhibits human mesenchymal stem cell (MSC) apoptosis and delays the development of replicative senescence in long-term cultures [125].
The optimal serum level is controversial and has been reported from 25 to 80 ng/mL with deficiency defined as less than 30 ng/mL [126]. By this standard, nearly 90% of healthy US adults are vitamin D deficient [127]. The prevalence of vitamin D insufficiency has been measured in 52.3% of sports medicine patients and 66.1% of trauma patients [128].
After total hip arthroplasty, patients with vitamin D deficiency have lower postoperative Harris hip scores [129]. Up to 83% of chronic lower back patients have abnormally low vitamin D levels, and clinical improvement is seen with vitamin D supplementation [130]. Vitamin D insufficiency also plays a critical role in children undergoing hematopoietic stem cell transplantation for malignancy. Hansson et al. demonstrated a more rapid increase in neutrophil granulocyte levels, decreased rejection, and increased survival rates in children who were vitamin D sufficient [131].
Vitamin D is synthesized from 7-dehydrocholesterol in the skin through exposure to sunlight. Many individuals do not get enough sunlight exposure, and those living in colder climates are at even greater risk for vitamin D deficiency. Therefore, vitamin D supplementation is recommended for most adults. Singh et al. studied the effect of vitamin D supplementation on serum vitamin D levels. They noted serum concentrations are influenced by age, body mass index (BMI), and serum albumin concentration [132]. They recommend 5000 IU/day for 3 months to correct vitamin D deficiency. After 3 months, they advise repeating the vitamin D serum concentration test. Once the deficiency has been corrected, a maintenance dose of 2000 IU/day is recommended.
Vitamin C (l-ascorbic acid) is an essential water-soluble vitamin required for the biosynthesis of collagen, protein metabolism, and wound healing. It is also an important antioxidant shown to regenerate antioxidants in the body, including vitamin E (alpha-tocopherol) [133]. Good sources of vitamin C include citrus fruits, tomatoes, potatoes, peppers, broccoli, strawberries, Brussel sprouts, and cantaloupe. When necessary, vitamin C supplementation of 1 g/day is recommended. At doses above 1 g/day, absorption falls to less than 50%, and unmetabolized ascorbic acid is excreted in the urine [133]. Each vitamin and mineral has a specific role to play in health, and once sufficient amounts of that nutrient to perform those roles are obtained, there is no benefit to consume more.
Manganese, vitamin B6 (pyridoxine), and vitamin E (alpha-tocopherol) are also linked to good tendon health; however, deficiency of these nutrients in well-nourished individuals is exceedingly rare. These essential nutrients are found in seafoods, nuts, whole wheat, seeds, tofu, chicken, turkey, and green leafy vegetables.
1.5.4 Dietary Supplements
The term “nutraceutical” was derived from the words “nutrition” and “pharmaceutical” and is defined as a food that offers health benefits [134]. The term, however, is not recognized by the US Food and Drug Administration (FDA) which uses the term “dietary supplement” instead. The FDA states that supplements are not intended to treat, diagnose, prevent, or cure diseases. Therefore, companies that produce them cannot make health claims.
Despite strong evidence of the health benefits of consuming a diet rich in whole fruits, vegetables, whole grains, and lean protein, there is little evidence for the multitude of dietary supplements offered to patients with orthopedic injuries. Despite this fact, approximately 62.5% of adults with arthritis reported taking at least one dietary supplement. Glucosamine and chondroitin sulfate are the most common supplements used by patients with osteoarthritis [135].
Eriksen et al. performed a systematic review and stratified meta-analysis of randomized placebo-controlled trials on the efficacy of glucosamine in the treatment of painful arthritis [136]. Glucosamine was shown to moderately reduce pain although a high level of between trial inconsistency was observed. The single most important explanation was product brand. Twelve trials using the Rottapharm/Madaus product resulted in significant pain reduction although a sensitivity analysis showed less promising results.
A large National Institutes of Health (NIH) study compared glucosamine hydrochloride, chondroitin, both supplements together, celecoxib, or a placebo in patients with mild knee osteoarthritis. Those who received the celecoxib had better short-term pain relief at 6 months than those who received the placebo. Those who received glucosamine and chondroitin supplements did not receive significant improvement in knee pain or function, although the investigators saw evidence of improvement in a small subgroup of patients with moderate-to-severe pain who took glucosamine and chondroitin together [137, 138]. The American College of Rheumatology indicates that there is insufficient evidence of efficacy and does not recommend glucosamine or chondroitin sulfate for patients with hand, hip, or knee osteoarthritis [139].
Fusini et al. performed a critical review of nutraceutical supplements in the management of tendinopathy [140]. They concluded that results were encouraging but were unable to confirm a benefit from glucosamine, chondroitin sulfate, vitamin C, type I collagen, arginine alpha-ketoglutarate, bromelain, curcumin, boswellic acid, or methylsulfonyl-methane (MSM). Numerous supplements have been proposed to reduce chronic inflammation associated with joint diseases such as osteoarthritis and rheumatoid arthritis. In a review by Ghasemian et al., ginger, rosemary, borage, Boswellia serrata, and urtica dioica (stinging nettle) have demonstrated preclinical or clinical evidence of efficacy and safety [141].
Osteoarthritis is a multifactorial process that includes articular cartilage catabolism, synovial tissue inflammation, and subchondral bone resorption. These pathological changes are associated with an excessive production of pro-inflammatory molecules such as interleukin 1-β (IL-β) and tumor necrosis factor alpha (TNF-α). A review of current nutraceuticals in the management of osteoarthritis summarized the biologic effects of several nutraceuticals consumed by patients [142].
Pomegranate juice (Punica granatum) has strong antioxidant properties due to their high content of hydrolyzable tannin and anthocyanins, a polyphenolic compound that exhibits antioxidant and anti-inflammatory capabilities. In osteoarthritic chondrocytes, pomegranate fruit extract (PFE) has been shown to suppress COX-2 enzyme activity and IL-1β-induced prostaglandin E2 (PGE2) and nitric oxide (NO) production [143]. Interleukin 1-β (IL-1β)-induced expression of matrix metalloproteinase (MMP)-1, MMP-3, and MMP-13 has been reduced by PFE in osteoarthritic chondrocytes as well [144]. Clinical studies of PFE efficacy in treating OA inflammation have not yet been performed.
Green tea (Camellia sinensis) contains catechins which have demonstrated anticancer, anti-inflammatory, antibacterial, and neuroprotective effects [142]. In particular, epigallocatechin-3-gallate (EGCG) decreases reactive oxygen species (ROS)-mediated cytotoxicity in human chondrocytes [145]. Several inflammatory mediators of inflammatory joint disease are inhibited by EGCG as well including IL-1β, TNF-α, IL-6, nitric oxide, and PGE2 in human chondrocytes [146, 147]. Encouraging evidence from in vitro and animal studies demonstrates the potential anti-inflammatory and antiarthritic effects of green tea; however, controlled clinical trials have not yet been completed.
Frankincense oil is an aromatic resin obtained from trees of the genus Boswellia. Boswellia serrata (B. serrata) in vitro has been reported to inhibit leukotriene biosynthesis [148], collagen IV and elastin hydrolysis [149], IL-1β-induced cartilage matrix breakdown, and nuclear factor kappa β (NFKB) in human chondrocytes [150]. Pain reduction, swelling, and disability have been demonstrated in human clinical trials using a herbomineral formulation containing B. serrata [151, 152], B. serrata extract with Aflapin or Laxin [153], or B. serrata 1000 mg daily [154] when compared with placebo.
Bromelain is derived from the pineapple plant stems and immature fruit. Bromelain influences inflammation by decreasing PGE2 and thromboxane A2 and modulation of immune cell surface adhesion molecules [155]. Phlogenzym (which contains 90 mg bromelain, trypsin, and rutin) is compared favorably to diclofenac 100–150 mg by reducing pain 80% in patients with knee osteoarthritis during a 4-week trial [156]. No serious adverse events were reported. Phlogenzym (which contains 540 mg bromelain) showed significantly better pain relief in patients with moderate-to-severe knee osteoarthritis than diclofenac 100–150 mg during a 12-week trial [157]. Again, no serious adverse events were noted. Although bromelain has demonstrated clinical efficacy, the optimum dosage remains unclear.
Cat’s claw (Uncaria tomentosa and Uncaria guianensis) is a vine found near the Amazon River with anti-inflammatory properties. U. tomentosa inhibits COX-1 and COX-2, TNF-α, PGE2, nitric oxide production, and lipopolysaccharide (LPS)-induced iNOS gene expression [158, 159]. Pain with daily activities due to Kellgren–Lawrence grade 2–3 knee osteoarthritis was significantly reduced in a 4-week placebo-controlled trial of cat’s claw bark extract; however, night pain was not improved [160].
Devil’s claw (Harpagophytum procumbens) is a desert plant found in southern Africa which claims benefits for digestion and joint and lower back pain. A dose-dependent analgesic and anti-inflammatory effect have been demonstrated in rats [161]. Devil’s claw inhibits PGE2 production, TNF-α, IL-1β, IL-6, MMPs, and nitric oxide [162–164]. Significant pain relief has been achieved with harpagoside 360 mg daily [165], harpagoside 57 mg daily [166], and harpagoside 100 mg daily [167] when compared to placebo in patients with osteoarthritis or nonspecific lower back pain.
Turmeric (Curcuma longa) comes from the C. longa root and contains curcuminoids (curcumin, demethoxycurcumin, bis-demethoxycurcumin) which are natural polyphenols with strong antioxidant capacity. A randomized double-blind placebo-controlled trial investigated the ability of curcuminoids to reduce systemic oxidative burden in patients suffering from knee osteoarthritis [168]. Forty mild-to-moderate knee osteoarthritis patients were given curcuminoid capsules 1500 mg/day in three divided doses or placebo for 6 weeks. Curcuminoids were coadministered with piperine (15 mg/day black pepper) in order to improve the bioavailability. Investigators measured the levels of superoxide dismutase (SOD) and malondialdehyde (MDA). Malondialdehyde results from lipid peroxidation of polyunsaturated fatty acids and is a product of thromboxane A2 synthesis. Serum superoxide dismutase (SOD) activity was significantly elevated, and malondialdehyde (MDA) concentration was significantly reduced in the treatment group when compared with the placebo group suggesting that short-term supplementation with curcuminoids attenuates systemic oxidative stress in patients with mild-to-moderate osteoarthritis.
Comblain [169] performed a review of dietary supplementation in dogs with osteoarthritis. They included studies of chondroitin sulfate, glucosamine sulfate, collagen hydrolysate, avocado–soybean unsaponifiables (ASU), curcumin, and polyunsaturated fatty acids (PUFAs). They conclude that dietary supplements are promising and associated with only minor adverse effects; however, the majority of the existing studies suffered from a small sample size and low statistical power.
Nitric oxide is a small free radical generated by nitric oxide synthase (NOS). In a series of experiments performed over 15 years, Murrell et al. [170] showed that nitric oxide is induced by all three isoforms of NOS during tendon healing and that it may restore tendon function by enhancing collagen and matrix synthesis.
Oxygen-derived free radicals have been implicated in the development of venous ulcers. Removing free radicals with antioxidant therapy expedites healing in such lesions [171]. Nitric oxide (NO) combines with hydroxyl free radicals to form peroxynitrate, a potent free radical that causes tissue injury. Nitric oxide overexpression may be involved in delayed wound healing through the production of peroxynitrate and its effects on vasculature, inflammation, and collagen deposition.
Three randomized, double-blind clinical trials have evaluated the efficacy of a nitric oxide patch in the management of tendinopathy. In all three trials, there was a significantly positive effect in clinical symptoms and function in patients with Achilles tendinopathy [172], tennis elbow [173], and supraspinatus tendinopathy [174]. In all three studies, patients were randomly assigned to receive either glyceryl trinitrate (GTN) patches delivering 1.25 mg GTN every 24 h or a placebo patch. In the tennis elbow study, 81% of the treatment group was asymptomatic compared with 60% of the control group. The Achilles tendinopathy study showed 78% of the treatment group asymptomatic at 6 months versus 49% of the control group. Lastly, 46% of patients with supraspinatus tendinopathy in the treatment group were asymptomatic compared with 24% of the control subjects. In addition to decreased pain, patients demonstrated increased power and improved function.
Some physicians recommend consumption of various bovine collagen hydrolysate preparations to improve joint collagen and proteoglycan metabolism. Schadow et al. have demonstrated that various collagen hydrolysates differ with respect to their chemical composition of collagen fragments but do not stimulate biosynthesis of collagen in humans [175]. There is evidence, however, that a leucine-rich diet combined with physical exercise stimulates collagen synthesis in tendons [176].
The best evidence to date suggests that no single nutrient or supplement is sufficient to improve tendon or joint healing. Rather a nutrient-rich diet that includes fruits, vegetables, essential fats, and proteins is recommended for optimal health.
1.5.5 Physical Activity
Optimal healing is facilitated by normalized cardiovascular health, muscle strength, and joint flexibility. According to the American College of Sports Medicine (ACSM), a program of regular exercise beyond activities of daily living is essential for most adults [177]. Adults should perform at least 150 min of moderate intensity exercise per week. Exercise recommendations can be met through 30–60 min of moderate intensity exercise 5 days per week or 20–60 min of vigorous intensity exercise 3 days per week in order to maximize cardiovascular health. Gradual progression of exercise time, frequency, and intensity is recommended for best adherence and lowest risk of injury. People unable to meet these minimum requirements can still benefit from some physical activity.
Adults are also advised to engage in resistance strengthening exercises 2–3 days each week. Two to four sets of each exercise will help improve strength and power. For each exercise, 8–12 repetitions per set improve strength and power, 10–15 repetitions improve strength in middle age and older people starting to exercise, and 15–20 repetitions improve muscular endurance. Adults should wait at least 48 h between resistance training sessions to allow for adequate recovery [177].
Static, dynamic, and ballistic stretches are all effective to maintain muscle and joint flexibility when performed 2–3 days a week. Flexibility exercises are most effective when performed after light aerobic activities to warm the muscles. Stretches should be held for 10–30 s to the point of slight discomfort for maximum effect.
Sedentary behavior, such as sitting at a computer or watching television for prolonged periods of time, has been shown to be an independent health risk. Meeting the recommended physical activity guidelines does not make up for a sedentary lifestyle.
It has been suggested that eccentric exercise programs promote collagen fiber cross-link formation and facilitate tendon remodeling [178]. The efficacy of eccentric exercise programs has been demonstrated in athletes and nonathletes with chronic Achilles tendinopathy [178, 179]. Pushing eccentric exercises through pain in patients with chronic Achilles tendinopathy was popularized in the 1990s with success rates as high as 100% [180]. Imaging of the Achilles tendon after a 12-week eccentric training protocol has demonstrated tendon structure normalization on ultrasound and MRI [181, 182]. Better results are noted with midsubstance Achilles tendinopathy when compared with insertional tendinopathy [183]. Rotator cuff tendinopathy and lateral epicondylitis also respond to strength training [184, 185].
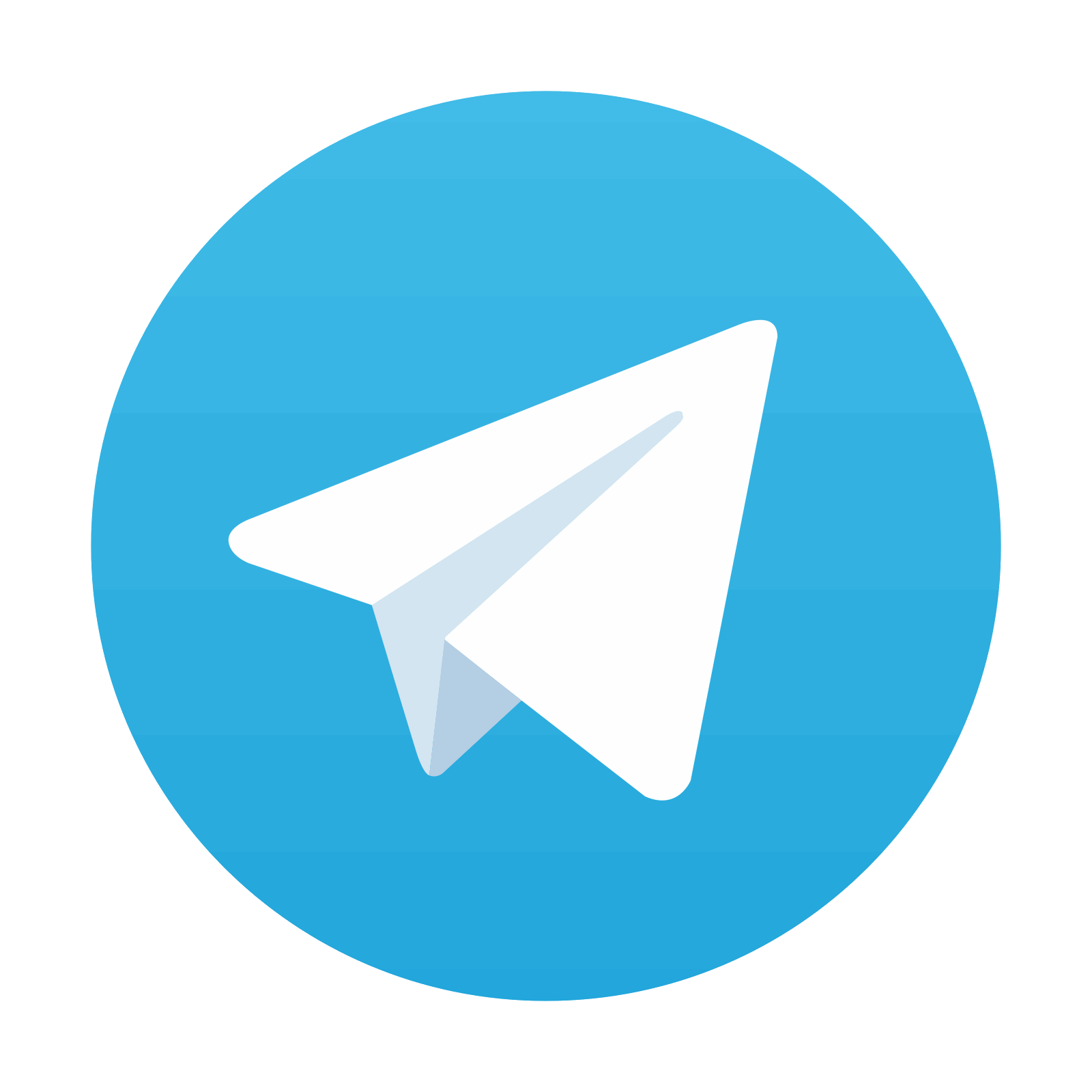
Stay updated, free articles. Join our Telegram channel

Full access? Get Clinical Tree
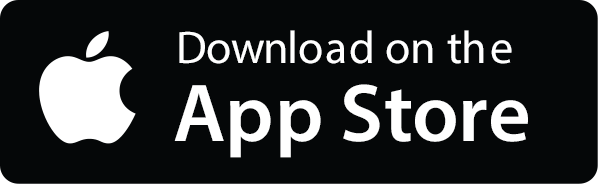
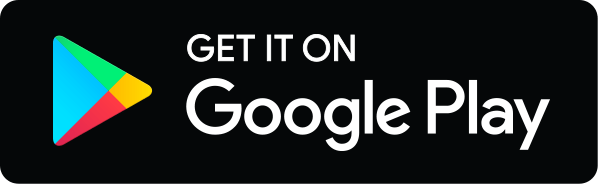