Active stretch is necessary for regulating muscle fiber length (ie, the number of series sarcomeres). Elevated cytoplasmic calcium is the proposed component of contractile activity required to activate signaling pathways for sarcomere number regulation. Passive stretch reduces muscle tissue stiffness, most likely by signaling connective tissue remodeling via fibroblasts. Passive stretch may induce sarcomere addition if the muscle fibers are lengthened sufficiently to raise cytoplasmic calcium through stretch-activated calcium channels. The magnitude of stretch in vivo is limited by the physiologic range of movement and stretch pain tolerance. The greatest effect of stretching muscle fibers is expected when the lengthening exceeds the optimum fiber length (Lo).
Skeletal muscle properties are dynamic and reflect the history of use over the past few days to weeks. The 4 major properties that determine the functional capacity are contractile strength, structural strength, endurance, and fiber length. This article focuses on length regulation and the effects of stretching. Stretching is widely practiced in clinical medicine and athletic activities. Poor outcomes have led clinicians and athletes to question the value of stretching but not to abandon the practice. Are there physiologic benefits to the muscle tissue? The desired outcomes of length regulation are improvements in the range of motion and performance and the reductions of stiffness and pain.
The International Fitness Association Web site describes 7 stretching techniques, but these techniques can be broadly categorized as either passive, in which the lengthened muscle does not contract, or active, in which the muscle contracts at some point during the procedure. Confusion about the expected outcomes of stretching has arisen in part because active stretch has been misinterpreted as passive stretch in joint immobilization animal studies. The immobilized muscles contract isometrically in the awake animal. In this model, the addition or loss of series sarcomeres altering muscle fiber length definitely occurs in the presence of activity. The assertion that passive stretch alone can change muscle length has not been demonstrated. In this article, the authors discuss evidence that passive tension regulates muscle stiffness and pain tolerance, and muscle fiber length is changed only when components of contractile activity, not yet delineated, are present.
The ability to adjust fiber length (sarcomere number) to meet the demands for force output at an optimal length is one of muscle’s greatest strengths. Under abnormal demands, this adaptability turns into a weakness, because muscle debilitation can occur secondarily to disease, aging, and inactivity. For example, healthy astronauts in superb physical condition were launched for 6-month missions aboard the International Space Station. Comparison of the preflight and postflight biopsies of soleus muscles revealed that, even with daily exercise, significant atrophy of slow fibers occurred. The deconditioning put the astronauts at risk for inadequate mobility during emergency egress and heightened vulnerability to eccentric muscle contraction injury upon return to Earth. Skylab investigations had demonstrated that during the first week of spaceflight, astronauts transitioned from the normal one-gravity standing posture to a microgravity, fetal-like floating posture with persistent plantarflexion foot drop. The chronic plantarflexion posture decreases the working range of soleus. In a 35-day bed rest study in which plantarfexion bias was also present, the altered posture caused the reduction of muscle fiber length. Upon reloading, the soleus fibers are hyperstretched, and the force output is reduced because of less overlap of thick and thin filaments and atrophy in fiber diameter. In this example, the muscle was normal before the event, and the maladaptation occurred secondarily to altered use. Interventions are needed to instruct the muscle to stay pre-event adapted.
What is known about muscle length adaptation?
Growth in muscle fiber length in neonates is required to keep pace with the expanding skeleton. The total number of muscle fibers appears to be present at birth in people and rats. As the neonates increase biomechanical activity, the working muscles grow in diameter. During the same period, elongation of the skeleton necessitates that the attached muscles keep pace in length by adding series sarcomeres. Bone elongation ceases after puberty, but the ability of muscle to adapt fiber length persists. Maladaptation of muscle length is common in the elderly suffering reduced mobility and bad posture.
Is contractile activity necessary to adjust muscle length?
The force output of a muscle cell depends on overlap of contractile filaments so that myosin cross bridges can bind to actin, hydrolyze adenosine triphosphate (ATP), and exert force. The number of sarcomeres in series is regulated to set optimal fiber length (L o ), the length at which the maximum number of cross bridges is possible, within the operating range of the muscle. What signals set L o , and how is the number of series sarcomeres regulated? The results of numerous animal studies of muscle adapting to short and long lengths are consistent with contractile activity being required. Contractile activity continues at 10% to 60% of normal in rat soleus muscles hypershortened following tenotomy (simulating tendon rupture) or when statically shortened or lengthened by immobilization. Contradicting the requirement for activity is the finding that the addition of sarcomeres proceeds in denervated muscles. Nerve transection immediately removes motor neuron impulses and silences the muscle fibers. However, denervated fibers within a day or two begin fibrillation contractions at 2 to 20 Hz. Thus, denervated muscle fibers are contracting, and the stretch is active. When the hindlimb muscles are completely silenced by spinal cord transection, but not denervated (no fibrillation), tenotomy-induced loss of series sarcomeres does not occur. One interpretation, and certainly not the only possibility, is that the depolarization events of endplate-generated contractions and fibrillations raise intracellular free calcium from the sarcoplasmic reticulum. A logical conclusion is that calcium-dependent signaling pathways are required to regulate series sarcomere number.
There may be a direct relationship between the level of contractile activity and the rate of sarcomere turnover. Compared with the slow soleus in the rat, the fast muscles (tibialis anterior, extensor digitorum longus) exhibit much less change in length during immobilization. The contractile activity of fast muscles in both normal and immobilized conditions is very low, about 3% of soleus activity, and remains so after tenotomy or immobilization. The lower activity correlates with the small changes in sarcomere number in fast muscles, whereas the high activity of slow muscles is associated with large changes. Fast and slow muscles were concluded to sense identical immobilization stimuli differently, but the amount of contractile activity may be the explanation. Fewer contractions mean less elevation of cytoplasmic calcium and weaker signaling. Other investigators favor contractile tension as the major factor regulating sarcomere number. While active tension cannot be dismissed, denervated fibers regulate sarcomere number, and the tensions generated during fibrillations are extremely small. Studies in which tension output was dramatically reduced chemically, but the elevation of cytoplasmic calcium remained high, showed that signaling activation persisted. Tension reduction needs to be tested for the effects on series sarcomere regulation. Eliminating all activity halts sarcomere breakdown, and increasing activity increases breakdown. The authors recently reported that passive daily stretch in rats does not prevent the loss of sarcomeres in tenotomized soleus muscles, but stretch plus contractile activity is effective. The failure of passive stretch to prevent sarcomere loss in animal models is consistent with the large number of studies in people reporting that passive stretch produces little or no increase in muscle length (extensibility) based on joint angle excursion after weeks of treatment. Thus, stretch plus an aspect of contractile activity is necessary for inducing series sarcomere turnover.
Some passive stretch studies in people report growth in muscle length. Perhaps, the lengthening elevated cytoplasmic calcium by opening stretch-activated calcium channels. Passive stretch in vivo may not activate these mechanisms when the magnitude of muscle lengthening is limited by the physical range of joint movement and the onset of stretch pain. However, if the L o of the target muscle had been maladapted to a shorter length, then the in vivo range of movement may be sufficient for activation of calcium channels. Two joint muscles, like the hamstrings, can be lengthened by flexing the hip and extending the knee joint at the same time to achieve greater percentage stretches beyond L o than are possible for normal one-joint muscles. Stretch pain may still limit the percentage of lengthening. Fortunately, daily passive stretch can increase stretch pain tolerance and allow greater lengthening. Another factor to consider when evaluating whether stretch can increase muscle length is the position of L o in the operating range. In biceps brachii, L o is near the middle range, whereas in soleus and the wrist flexors/extensors, L o is close to the end of the range. Thus, predicting the effects of passive stretch in vivo requires consideration of the existing L o point and range of motion permitted physiologically as well as stretch pain tolerance.
Is contractile activity necessary to adjust muscle length?
The force output of a muscle cell depends on overlap of contractile filaments so that myosin cross bridges can bind to actin, hydrolyze adenosine triphosphate (ATP), and exert force. The number of sarcomeres in series is regulated to set optimal fiber length (L o ), the length at which the maximum number of cross bridges is possible, within the operating range of the muscle. What signals set L o , and how is the number of series sarcomeres regulated? The results of numerous animal studies of muscle adapting to short and long lengths are consistent with contractile activity being required. Contractile activity continues at 10% to 60% of normal in rat soleus muscles hypershortened following tenotomy (simulating tendon rupture) or when statically shortened or lengthened by immobilization. Contradicting the requirement for activity is the finding that the addition of sarcomeres proceeds in denervated muscles. Nerve transection immediately removes motor neuron impulses and silences the muscle fibers. However, denervated fibers within a day or two begin fibrillation contractions at 2 to 20 Hz. Thus, denervated muscle fibers are contracting, and the stretch is active. When the hindlimb muscles are completely silenced by spinal cord transection, but not denervated (no fibrillation), tenotomy-induced loss of series sarcomeres does not occur. One interpretation, and certainly not the only possibility, is that the depolarization events of endplate-generated contractions and fibrillations raise intracellular free calcium from the sarcoplasmic reticulum. A logical conclusion is that calcium-dependent signaling pathways are required to regulate series sarcomere number.
There may be a direct relationship between the level of contractile activity and the rate of sarcomere turnover. Compared with the slow soleus in the rat, the fast muscles (tibialis anterior, extensor digitorum longus) exhibit much less change in length during immobilization. The contractile activity of fast muscles in both normal and immobilized conditions is very low, about 3% of soleus activity, and remains so after tenotomy or immobilization. The lower activity correlates with the small changes in sarcomere number in fast muscles, whereas the high activity of slow muscles is associated with large changes. Fast and slow muscles were concluded to sense identical immobilization stimuli differently, but the amount of contractile activity may be the explanation. Fewer contractions mean less elevation of cytoplasmic calcium and weaker signaling. Other investigators favor contractile tension as the major factor regulating sarcomere number. While active tension cannot be dismissed, denervated fibers regulate sarcomere number, and the tensions generated during fibrillations are extremely small. Studies in which tension output was dramatically reduced chemically, but the elevation of cytoplasmic calcium remained high, showed that signaling activation persisted. Tension reduction needs to be tested for the effects on series sarcomere regulation. Eliminating all activity halts sarcomere breakdown, and increasing activity increases breakdown. The authors recently reported that passive daily stretch in rats does not prevent the loss of sarcomeres in tenotomized soleus muscles, but stretch plus contractile activity is effective. The failure of passive stretch to prevent sarcomere loss in animal models is consistent with the large number of studies in people reporting that passive stretch produces little or no increase in muscle length (extensibility) based on joint angle excursion after weeks of treatment. Thus, stretch plus an aspect of contractile activity is necessary for inducing series sarcomere turnover.
Some passive stretch studies in people report growth in muscle length. Perhaps, the lengthening elevated cytoplasmic calcium by opening stretch-activated calcium channels. Passive stretch in vivo may not activate these mechanisms when the magnitude of muscle lengthening is limited by the physical range of joint movement and the onset of stretch pain. However, if the L o of the target muscle had been maladapted to a shorter length, then the in vivo range of movement may be sufficient for activation of calcium channels. Two joint muscles, like the hamstrings, can be lengthened by flexing the hip and extending the knee joint at the same time to achieve greater percentage stretches beyond L o than are possible for normal one-joint muscles. Stretch pain may still limit the percentage of lengthening. Fortunately, daily passive stretch can increase stretch pain tolerance and allow greater lengthening. Another factor to consider when evaluating whether stretch can increase muscle length is the position of L o in the operating range. In biceps brachii, L o is near the middle range, whereas in soleus and the wrist flexors/extensors, L o is close to the end of the range. Thus, predicting the effects of passive stretch in vivo requires consideration of the existing L o point and range of motion permitted physiologically as well as stretch pain tolerance.
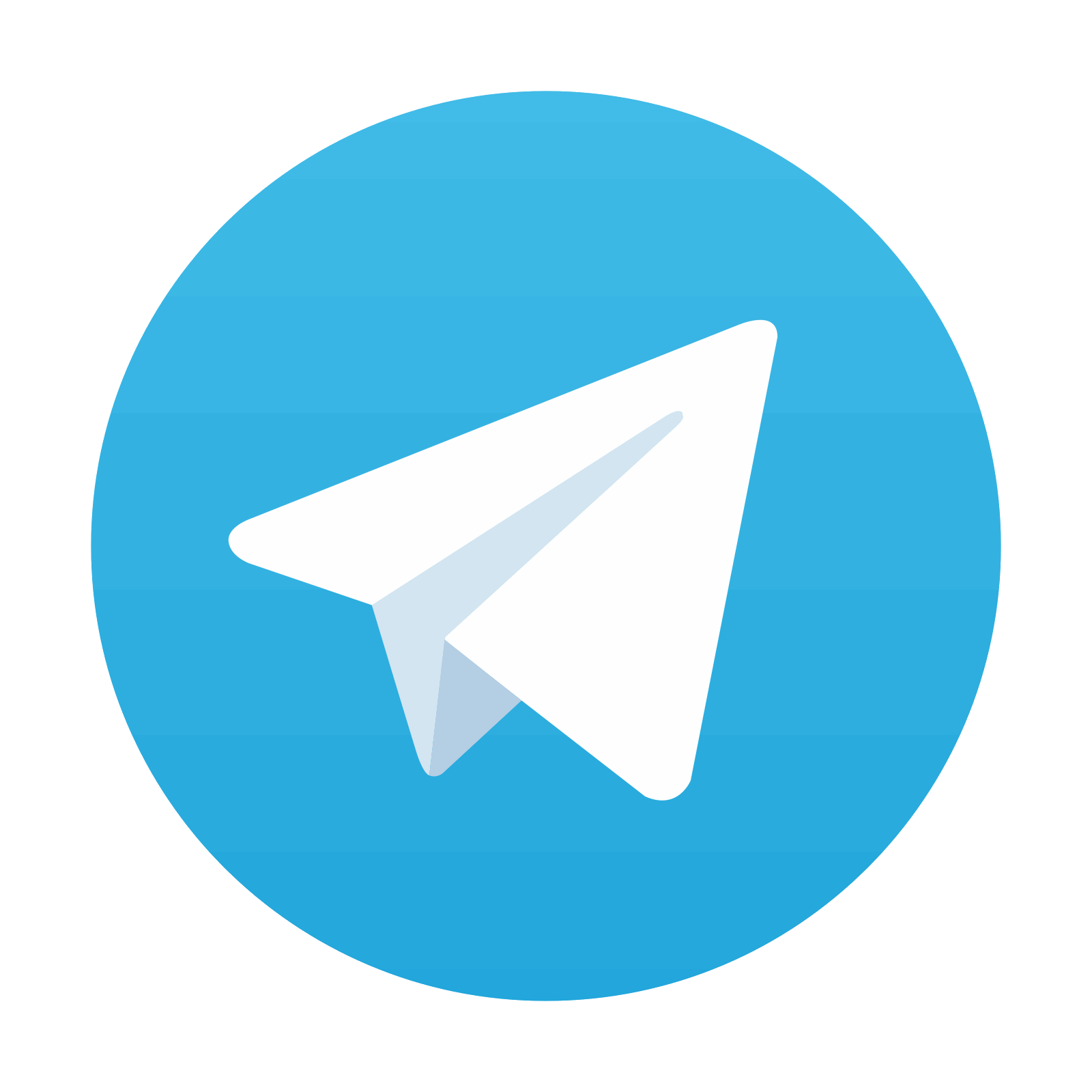
Stay updated, free articles. Join our Telegram channel

Full access? Get Clinical Tree
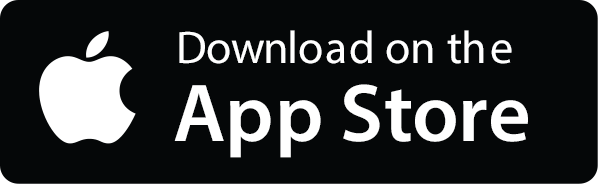
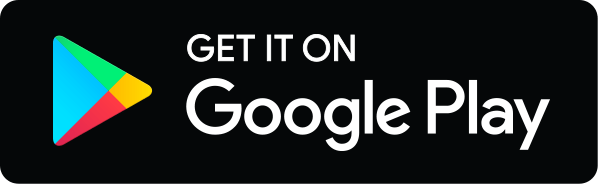