Tendon Disorders in the Lower Extremity
Mark A. Glazebrook MD, MSC, PhD, DIP SPORTS MED, FRCS(C)

Achilles tendon disorders represent a small portion of tendon disorders in general, but are cited to be increasing in frequency in the modern world.
The most common patient profile for Achilles tendon rupture is a male in his third or fourth decade of life that plays sports occasionally.
Magnetic resonance imaging (MRI) is the gold standard for imaging musculoskeletal soft tissues, yielding the most detailed information about the midsubstance of the tendons and surrounding structures.
Only short periods of immobilization are recommended in severe cases because prolonged immobilization is detrimental to tendon and muscle strength and worsens joint stiffness.
The goal of treatment for tibial posterior tendon injuries is relief of symptoms, restoration of function, and prevention of progression of the disease. Early stages are usually treated nonoperatively. Late stages may require surgical treatment with debridement, tendon transfer, or various forms of arthrodesis.
The primary function of the peroneal tendons is that of eversion and plantarflexion of the foot. The peroneus longus is also responsible for plantar flexion of the first metatarsal.
Peroneal tendon instability often coexists with peroneal tendon disease and partial tears. Instability may be viewed as a continuum with frank traumatic dislocation at one end and subluxation within the peroneal groove at the other end. Traumatic dislocation is associated with sports, including skiing, soccer, football, ice skating, and gymnastics.
Tendon disorders about the foot and ankles are both a diagnostic and therapeutic challenge. Most disorders result from overuse with intrinsic and extrinsic factors contributing in a multifactorial manner. To understand and clinically manage tendon problems of the foot and ankle one must gain an understanding of the basic science of normal and diseased tendon including anatomy, biology, and biomechanics. Also, expertise and experience in clinical evaluation and treatment are essential. This chapter will begin with comprehensive discussions on the management of tendon disease in the foot and ankle.

Tendon Anatomy
Collagen accounts for approximately 70% of the dry weight of a tendon (1) with 95% of this collagen being Type 1 (2). The tendon microstructure was described by Kastelic et al. (3). The endotenon is a mesh of loose connective tissue that encloses blood vessels, lymphatics, nerves, fibroblasts, and bundles of collagen fibers. The endotenon layer of the tendon is continuous with the muscles perimysium at the musculotendinous junction. The tendon has proprioceptive nerve endings at the musculotendinous junction and pain receptors ending in the peritendinous tissue. The mechanoreceptors include Pacini corpuscles (velocity sensors), Ruffini corpuscles (pressure sensors), and Golgi tendon organs (tension receptors).
Tendon Biology
Nondiseased Tendons
Approximately 95% of tendon’s collagen is Type I, which is embedded in an extracellular matrix of proteoglycans. Elastin represents only a small portion (2). The biosynthesis of collagen begins with polypeptides that are synthesized and then hydroxylated and glycosylated. These polypeptides aggregate into a triple helix referred to as procollagen. The procollagen bundles are then secreted by the fibroblasts where they undergo extracellular modifications including hydrolysis of peptide bonds to form a collagen molecule (tropocollagen). The collagen molecule is the most fundamental unit of the hierarchical organization of the tendon as described by Kastelic et al. (3) (Fig 50-1). Five of these tropocollagen units aggregate to form a microfibril. Intramolecular cross-links exist within the collagen molecules while intermolecular cross-links bind adjacent collagen molecules together. The microfibrils associate in a quartered staggered array (overlap by one-fourth) and are grouped into subfibrils, which in turn are grouped to form fibrils. The fibrillar collagens polymerize side-by-side and end-to-end into a long fibrillar aggregate or collagen fibers. The collagen fibrils are arranged longitudinally but have a wavy or crimped appearance (4,5) secondary to the helical structure of the underlying molecules.
The nondiseased tendon has well described histological features (6) including highly organized, tightly packed parallel collagen bundles (6,7) that stain pink-red (eosinophilic) with hematoxylin and eosin staining (7) (Fig 50-2). The sparse nuclei are elongate and found interspersed between the collagen bundles (6,7).
Diseased Tendon
The terminology used to describe tendon disease is somewhat confusing and there is a lack of consistency in the literature (8,9). Some use the term tendonitis with the suffix
“itis” denoting inflammation that is often the case when the paratenon is involved with or without involvement of the tendon itself. Others use the term tendinosis with “osis” denoting a degenerative process of the tendon itself that may be clinically asymptomatic with no evidence of an inflammatory infiltrate (12,13,14,15,16).
“itis” denoting inflammation that is often the case when the paratenon is involved with or without involvement of the tendon itself. Others use the term tendinosis with “osis” denoting a degenerative process of the tendon itself that may be clinically asymptomatic with no evidence of an inflammatory infiltrate (12,13,14,15,16).
![]() Fig 50-1. Hierarchical organization of the tendon. (Adapted from Stanish WD, Curwin S, Mandell S. Tendinitis: Its etiology and treatment. Oxford University Press; 2000:49–64; with permission.) |
Descriptions of the histology of diseased tendons are derived from biopsies of human patients with subcutaneous rupture and human patients with chronic, localized tendon symptoms. These studies demonstrate many consistent features suggestive of a common pathological process that may represent a continuum with tendon rupture as the end-stage. The histopathological features include: disorganized collagen arrangement, increased number of cells, rounding of cell nuclei, variable collagen staining, and increased glycosaminoglycan content (13,17,18,19,20) (Fig 50-3). These features may represent a healing response to repetitive microtrauma (21).
Tendon Biomechanics
The biomechanical response of the tendon to strain was reviewed by Stanish et al. (22) (Fig 50-4). When the tendon is strained at low levels, the first response is a straightening of the wavy appearance of the collagen fibrils. This is represented by the “toe region” of the stress strain curve and results from the decreased elastic modulus as supplied by the elastic fiber component of the tendon (23).
As strain increases, the collagen fibers are engaged and the increased modulus of elasticity is reflected by the more linear portion of the tendon stress-strain curve. As long as the strain remains less than 4% the process is reversible due to the elastic recoil of the elastic component of the tendon. At strain levels of 4% to 8% the collagen fibrils slide past one another and the cross links begin to break (24). This results in microtrauma (molecular damage) to the tendon. The stress strain curve becomes linear as the tendon stiffens, and as collagen cross links begin to fail, the curve becomes convex and the tendon begins to rupture. At strains greater than 8% it is common for the tendon to fail or rupture completely. This represents the highest point on the stress-strain curve (ultimate tensile failure). The mechanical failure in the underlying collagen is not due to breakage of collagen molecules, but pulling apart of adjacent molecules, and it is generally believed that when the tendon fails it is most often a consequence of increased forces placed across abnormal tendon.

Achilles tendon disorders represent a small portion of tendon disorders in general. Achilles disorders are cited to be increasing in frequency in the modern civilized world (13,14,25). Most disorders result from overuse with intrinsic and extrinsic factors contributing in a multifactorial manner. The end result is a diseased tendon that is both symptomatic and biomechanically inferior predisposed to catastrophic rupture.
Achilles Anatomy
A merging of the insertions of the gastrocneimus, which has its origin at the femoral condyle posteriorly, and the soleus, originating from the posterior superior aspect of the tibia and fibula, forms the Achilles tendon. The soleus component varies from 3 to 11 cm while the gastrocneimus varies from 11 to 26 cm (26). The confluence of these two tendons insert on the calcaneus posterior and inferior to the superior calcaneal tuberosity. The Achilles tendon rotates 30 to 150 degrees before its insertion point. This permits elongation and elastic recoil within the tendon allowing stored energy to be released during the appropriate phase of gait.
The blood supply of the Achilles arises from branches of the posterior tibial and peroneal arteries and is similar to that for other tendons surrounded by a paratenon as described by Mayer (27). Namely, it receives its blood from three sources: vessels in the musculotendinous junction, vessels in the surrounding connective tissue, and possibly from the osseous insertion vessels. A consistent decrease in the number of blood vessels in the mid portion of the Achilles tendon has been documented (28,29,30). This corresponds to the area identified by Kreuger-Franke et al. (31) as the most common site of rupture.
Achilles Tendon Biomechanics
Absolute force and the angular application of these forces play a role in the creation of Achilles tendon pathology. Peak forces of 3786 N have been measured within the Achilles tendon (32), which corresponds to a force that approximates six to eight times body weight during vigorous activities (30,33); however, the Achilles most commonly experience stresses that correspond to strains less than 4% (24) corresponding to a force of five times body weight (33).
Achilles Clinical Implications
Although the clinical presentation of an acute Achilles rupture may be a somewhat obvious chronic rupture or more subtle, Achilles pathology may present a diagnostic challenge.
Achilles pathology may be categorized as insertional tendonitis involving the tendon-bone junction or noninsertional tendonitis involving the tendon proximal to its insertion (34). Noninsertional tendonitis may involve the paratenon and/or the tendon itself. Involvement of either of these two components may occur in isolation or in combination (Fig 50-5) as classified by Puddu et al. (10).
Insertional Achilles tendon disease may also be subdivided into insertional calcific tendonitis, Haglunds deformity (referring to a prominent posterior superior tuberosity of the calcaneus), retrocalcaneal bursitis, precalcaneal bursitis, and calcaneal exostosis “pump bump” or “skaters heel.”
Catastrophic rupture of the Achilles tendon (Fig 50-6) represents the final stage of Achilles tendon pathology. The most common patient profile for human Achilles tendon rupture would be that of a male in his third or fourth decade of life that plays sport occasionally. Men to women rupture rate has been reported from 2:1 to 12:1 (10,35,36). The mean age has been estimated between the 30’s and 40’s (17,18,31,36,37) with the left Achilles being ruptured more commonly than the right, probably reflecting right side dominance with left leg pushing off (38). The site of rupture has been reported to occur in the myotendinous junction in 12.1%, the insertion in 4.6%, and 3.5 cm proximal to the insertion in 83% (36).
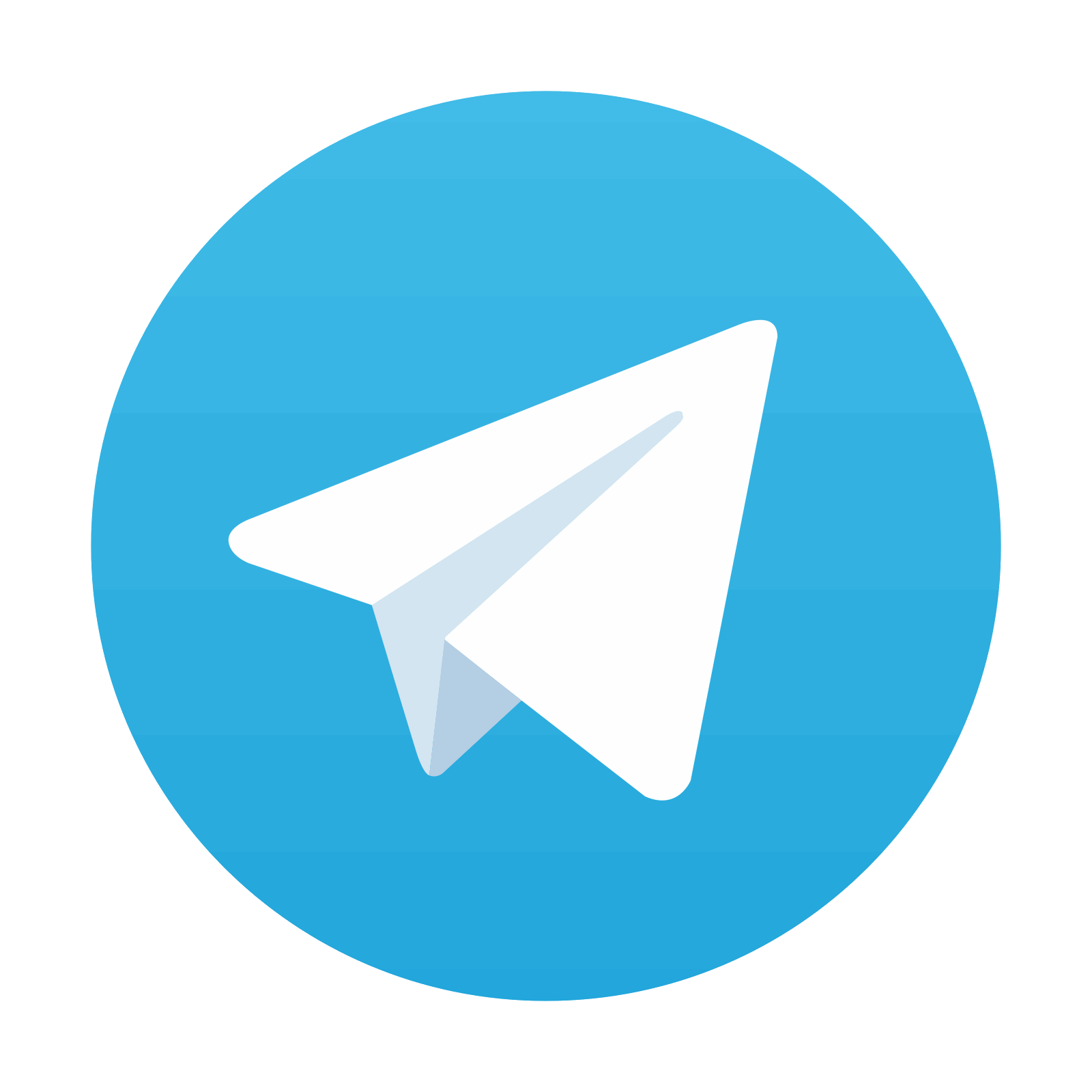
Stay updated, free articles. Join our Telegram channel

Full access? Get Clinical Tree
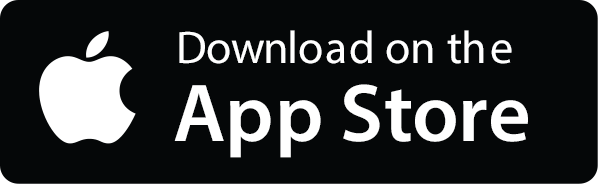
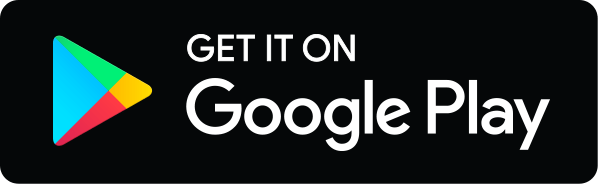