Fig. 5.1
Caveolins regulate myostatin and related TGF-β signaling at the type I receptor level. (a) Schematic representation of myostatin and related TGF-β signaling and caveolin-3. Type I serine/threonine receptor (R) controls intramuscular signaling by activating R-Smad (Smad2/3). Caveolin-3 suppresses the type I receptor. (b) In vitro autophosphorylation of constitutively active type I TGF-β receptors, ALK4 and ALK5. Cell lysates from COS-7 cells cotransfected with FLAG-tagged wild-type or mutant caveolin-3 (CAV3P104L) and HA-tagged constitutively active ALK4 (HA-caALK4) or ALK5 (HA-caALK5) were immunoprecipitated with anti-HA agarose. The in vitro kinase reaction was initiated by the addition of kinase reaction buffer and [γ-32P] ATP. Phosphorylated caALK4 and caALK5 were detected by autoradiography. Immunoprecipitated caALK4, caALK5, and caveolin-3 were analyzed by immunoblotting with anti-HA (α-HA) or anti-FLAG (α-FLAG) mAb. Bands corresponding to phosphorylated type I receptor (in vitro kinase assay), total type I receptor (α-HA), and caveolin-3 (α-FLAG) are shown
5.3 Ceveolin-3 Suppresses the Type I TGF-β Receptor: Relationship to the Pathogenesis of Caveolin-3-Deficient LGMD1C
Recently, caveolin-1 has drawn considerable attention as a critical regulator of TGF-β signaling. Caveolin-1 binds to and suppresses activation of the TGF-β1 receptor type I serine/threonine kinase (TβRI), which induces growth arrest in non-muscle cells [26]. Caveolin-1 facilitates ligand-bound TβRI internalization and ubiquitin-mediated degradation [27]. In addition, caveolin-1 interacts with type II and type I receptors for bone morphogenic proteins (BMPs) in vivo [28]. These findings indicate that caveolin-1 regulates TGF-β signaling at the receptor level.
Based on the role of caveolin-1 in non-muscle cells, we postulated that caveolin-3 likely inhibits myostatin and TGF-β signaling in muscle cells. Indeed, we found several caveolin-3 binding motifs in the cytoplasmic kinase domain of the TβRI [29]. We cotransfected caveolin-3 and a constitutively active TβRI in COS-7 monkey kidney cells (Fig. 5.1b). We found that caveolin-3 colocalized and co-immunoprecipitated with the TβRI. Furthermore, phosphorylation of the TβRI was decreased by wild-type caveolin-3 and, conversely, increased by the P104L disease-causing mutant caveolin-3 in vitro [3]. These results indicate that wild-type caveolin-3 inhibits activation of the TβRI, whereas mutant caveolin-3 enhances activation. Genetic introduction of the myostatin prodomain or administration of type II decoy receptor (ActRIIB-Fc) drastically rescues muscle atrophy in a LGMD1C mouse model (CAV3P104L). This therapeutic effect is associated with a decrease in TGF-β signaling, which is elevated in mutant mouse muscle [29]. Thus, caveolin-3 normally suppresses myostatin and TGF-β signaling at the type I receptor level, thereby preventing muscular atrophy. These findings suggest that excessive TGF-β signaling, triggered by caveolin-3 deficiency, could participate in the pathogenesis of muscular atrophy in LGMD1C.
5.4 TβRI Inhibitors Potentially Suppress Multiple TGF-β Signaling Pathways
Small-molecule compounds antagonizing TGF-β signaling at type I receptors (TβRIs) have recently been developed as targeted drugs for advanced cancer [30]. Tumor cells in advanced stages become refractory to TGF-β-induced growth arrest, but often overexpress TGF-β1, TGF-β2, and TGF-β3 [31]. TGF-β family members promote the epithelial–mesenchymal transition, immunosuppression, and angiogenesis, resulting in tumor growth and metastasis [31]. Each member of the TGF-β family binds to the type II receptor, which, in turn, recruits a TβRI. Seven different TβRIs—activin receptor-like kinases 1–7 (ALK1–7)—determine the intracellular signaling specificity of the 33 members of the TGF-β family. Small-molecule TβRI inhibitors were originally developed to compete with the binding of adenosine triphosphate (ATP) to the kinase domain of ALK5, the TβRI for TGF-β1–3 [15, 16]. TβRI inhibitors have been found to suppress tumor enlargement and metastasis in the advanced stages of cancer in animals [32, 33]. Notably, these inhibitors also suppress a similar kinase domain in ALK4, the type I receptor for activin, and potentially block ALK4/5, the type I receptor for myostatin [30]. However, the effects of TβRI inhibitors on activin, myostatin, and TGF-β1–3 signaling in muscle remain unclear.
5.5 A TβRI Inhibitor Ameliorates Muscle Atrophy and Weakness in a Rodent Model of Caveolin-3-Deficient LGMD1C by Impacting Muscle Precursor Cells
Myostatin suppresses the differentiation of C2C12 myoblasts exposed to low-serum conditions [34]. Using an efficient retrovirus-mediated gene transfer system [35], we assessed the effect of a TβRI inhibitor, Ki26894 [33], on the differentiation of C2C12 myoblasts expressing TGF-β1 or activin A, as well as myostatin (Fig. 5.2a) [36]. Transferring C2C12 myoblasts expressing an empty vector from high-serum (growth) to low-serum (differentiation) media caused them to fuse and form multinucleated myotubes. We then stained the cells with antibodies against myosin heavy chain (MyHC). Adding Ki26894 to the culture media enhanced myoblast fusion and myotube formation (Fig. 5.2a, green). In comparison, myotube formation was impaired in C2C12 myoblasts expressing myostatin, activin A, or TGF-β1 (Fig. 5.2a, blue), compared with controls harboring an empty vector. Notably, Ki26894 alleviated the impairment in myotube formation induced by the TGF-β family members (Fig. 5.2a, red). TβRI inhibitors enhance myoblast differentiation in vitro by suppressing the activity of several members of the anti-myogenic TGF-β family, including activin, TGF-β1, and myostatin. Ki26894 also prevented the impairment in myotube formation induced by LGMD-causing mutant caveolin-3 [36].
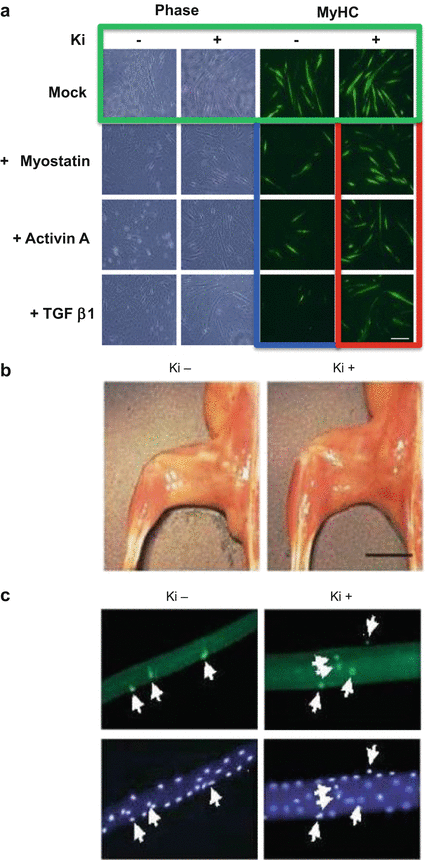
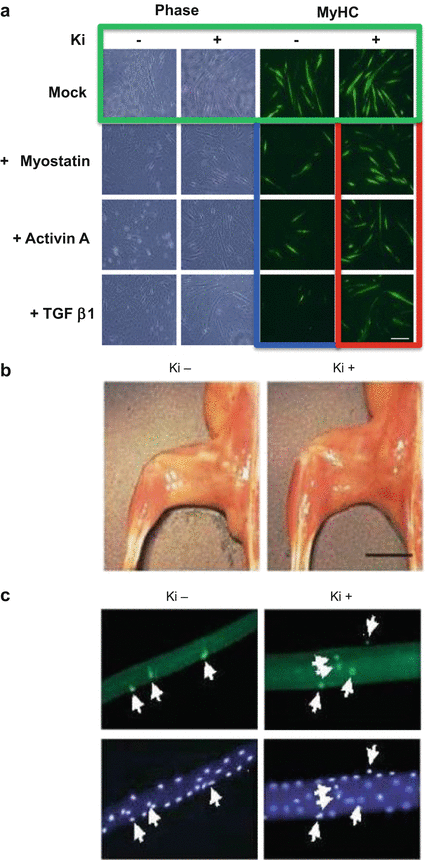
Fig. 5.2
TβRI inhibitor Ki26894 (Ki) prevents muscular atrophy in a caveolin-3-deficient LGMD1C mouse model. (a) Ki26894 suppresses the impairment in myogenesis produced by myostatin, activin A, and TGF-β1. C2C12 myoblasts expressing an empty vector were grown in DMEM containing 10 % FBS (growth medium) and then differentiated in DMEM containing 2 % horse serum (differentiation medium), without (−) or with (+) 10 nM Ki26894 for 6 days. The cells were then stained with the muscle differentiation marker myosin heavy chain (MyHC). (b) Oral administration of Ki26894 ameliorates muscular atrophy. Appearance of de-skinned hind limb from 16-week-old mice treated without (−) or with (+) Ki26894. Scale bar, 5 mm. (c) Fluorescence images of satellite cells attached to single myofibers isolated from the EDL muscles of a rodent model of caveolin-3-deficient LGMD (CAV3P104L) at 16 weeks of age. The white arrow indicates caveolin-1-positive satellite cells
We orally administered Ki26894 to a rodent model of caveolin-3-deficient LGMD (CAV3P104L) from 6 to 16 weeks of age. Muscle atrophy was reduced in Ki26894-treated animals compared with untreated mice (Fig. 5.2b). Ki26894 increased muscle weight, muscle strength, and myofiber size, as well as centrally nucleated regenerative myofiber number in the mouse [36]. Furthermore, Ki26894 ameliorated the reduction in the number of satellite cells attached to isolated single myofibers in the mice (Fig. 5.2c). Ki26894 also increased muscle mass and strength and elevated the number of satellite cells, in wild-type littermate mice. These findings indicate that TβRI inhibitors strongly antagonize anti-myogenic TGF-β signaling and alleviate muscle atrophy.
5.6 Future Directions
As mentioned above, caveolin-3 regulates skeletal muscle volume by suppressing multiple anti-myogenic TGF-β signaling pathways. Pharmacological intervention strategies based on TβRI inhibitors are expected to prevent the progression of muscle weakness better than those based on inhibitors that suppress myostatin signaling alone. An increase in the number of satellite cells and the enhancement of myoblast differentiation induced by this type of inhibitor suggests that they may hold substantial therapeutic potential for the treatment of muscle atrophy in various diseases, including muscular dystrophies, sarcopenia, and cancer cachexia. To evaluate their effect on muscle performance, TβRI inhibitors should be tested in large-animal models of muscular atrophy. However, much additional study is required before clinical application of these compounds. For example, it is necessary to define the optimal, but nontoxic, dosage of these drugs for the treatment of individual patients with muscular atrophy. To facilitate this, we have developed an ex vivo myostatin activity assay as a convenient real-time biomonitoring system to determine how myostatin signaling is affected by the administration of these drugs in individual patients [36]. Furthermore, novel delivery systems targeting skeletal muscles also require development before the full potential of these drugs can be realized.
Acknowledgment
We are grateful to all collaborators involved in developing anti-myostatin therapeutics and to T. Kenmotsu, N. Naoe, M Harada, M. Kimura, F Uemura, E Sugita, and K. Tanda (Department of Neurology, Kawasaki Medical School) for providing technical assistance. This work was supported by a research grant for Neurological and Psychiatric Disorders from the National Center of Neurology and Psychiatry (23–5, 26–8) and Welfare of Japan and from the Japan Society for the Promotion of Science (JST, AS24212769) and by research project grants from Kawasaki Medical School (23-T1, 26-T1).
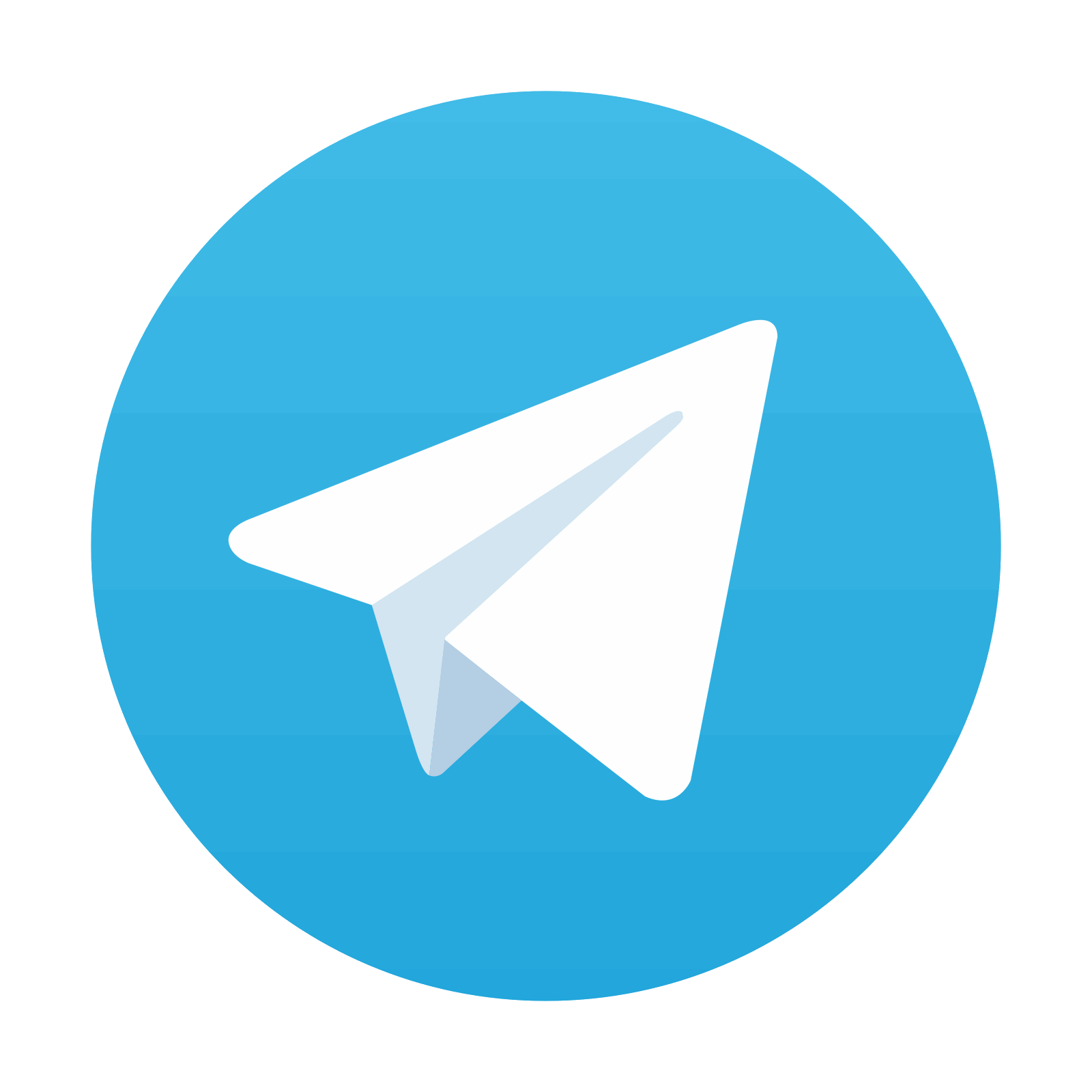
Stay updated, free articles. Join our Telegram channel

Full access? Get Clinical Tree
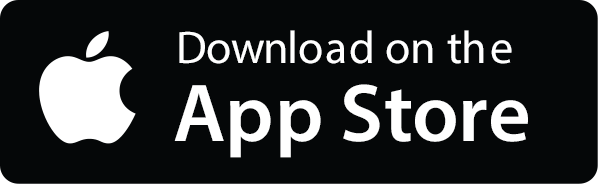
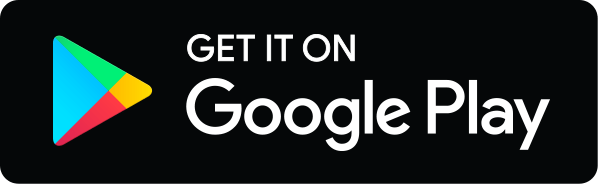