Stress Fractures of the Athletic Leg, Ankle, and Foot
Donald R. Bohay MD, FACS
Jonathan P. Cornelius MD
John G. Anderson MD

Military personnel are more prone to stress fractures of calcaneus and metarsals. Athletic injured patients have more tibial stress fractures.
Stress fractures are most common in running sports as vertical ground reaction forces are three times greater compared to walking.
Fatigue fractures occur as a result of repetitive activity, when bone is subjected to abnormal stress.
With the proper index of suspicion, stress fractures can be diagnosed early. In most cases, patients will return to their prior level of competition after nonoperative treatment.
Patients with stress fractures give a classic history of insidious onset of pain in a localized area. The pain is exacerbated by repetitive activity and relieved by rest.
A change in running surface or shoes occasionally relates to the onset of symptoms.
Standing x-rays are the first line of imaging for these injuries. Most x-rays will initially be negative. A bone scan or magnetic resonance imaging (MRI) can be used for definitive diagnosis and may be positive within a few days of injury.
Stress fractures of the sesamoids and phalanges, although uncommon, do occur.
Fractures of the base of the second metatarsal may be seen in ballet dancers who are routinely in the en pointe position.
Stress fractures of the cuboid are rare and occur in runners.
Stress fractures of the navicular are common in basketball, football, and track and field athletes and difficult to diagnose and treat. Some cases require operative intervention.
Calcaneal stress fractures are more common than those in the talus. Patients will present with pain and swelling on both sides of the heel. These injuries occur in the military and in dancers.
Tibial shaft stress fractures are subdivided into fractures of the posterior and anterior tibia. Anterior tibial stress fractures are more problematic and should be considered for surgical intervention.
Stress fractures were originally described by Breithaupt (1), a Prussian military physician, in 1855. The observation was made after examination of soldiers with swollen, painful feet. From the first description of “march fractures,” our current understanding of stress fractures has grown immensely. Military personnel are different from sports injured patients as they are more prone to stress fractures of the calcaneus and metatarsals (2). Athletic injured patients have more tibial stress fractures (3,4,5). Stress fractures are seen in 10% to 15% of sports medicine patients (5,6,7). Stress fractures are most common in running sports as vertical ground reaction forces are three times greater compared to walking (8). Insufficiency fractures are defined as fractures that occur in abnormal bone which has been subjected to normal stress, and will not be covered here. Fatigue fractures occur when normal bone is subjected to abnormal stress.

Certain anatomical variants have been found to predispose patients to fatigue fractures. Pronated feet are implicated in tarsal and tibial stress fractures (4,7). An anatomic study by Giladi et al. (9) showed a high degree of external rotation
of the hip and narrow tibiae predispose individuals to stress fractures of the lower extremity. Other anatomical variants found to increase chances of stress fracture are leg length inequality and excessive forefoot varus (8). Talocalcaneal and calcaneonavicular coalitions place the talus at risk of fatigue fracture (6). A rigid cavus foot will predispose the athlete to fatigue fracture (10).
of the hip and narrow tibiae predispose individuals to stress fractures of the lower extremity. Other anatomical variants found to increase chances of stress fracture are leg length inequality and excessive forefoot varus (8). Talocalcaneal and calcaneonavicular coalitions place the talus at risk of fatigue fracture (6). A rigid cavus foot will predispose the athlete to fatigue fracture (10).
Biomechanics
Fatigue fractures occur as a result of repetitive activity. One proposed etiology suggests that fatigued muscles fail causing higher strains (7). Bone is subjected to compressive and tensile forces. Muscle contraction converts tensile forces into compressive forces, a phenomenon referred to as stress shielding. This protects the bone and prevents injury. When muscles fatigue this protective process fails and microfractures accumulate (6). In addition, muscle fatigue is accompanied by changes in gait, which may also alter the force on bone.
Pathophysiology
Wolff’s Law of Transformation states bone undergoes cyclical stress and rest allowing it to remodel the bony architecture to optimally withstand its environment. Bone is subjected to stress microfractures. Cortical bone remodels initially by osteoclastic resorption at the level of the osteon. Bone loss peaks at 3 weeks. The resorption cavities are filled with lamellar bone by osteoblasts, producing the Haversian system. The cycle takes 3 months to complete. An imbalance of resorption and bone deposition leads to weakening of bone (11). On the tensile side of bone, osteons debond at the cement lines causing microfractures (10). On the compression side, oblique cracks and longitudinal splitting occurs through canalicular defects and the Haversian canals (7). In cancellous bone stress forms trabecular microfractures producing microcallus. These thickened trabeculae appear as sclerosis on x-rays. Stress fractures represent a continuum of injury and bony response. In this dynamic process, if the stress continues, the fatigue fracture may become complete and ultimately displaced (3).
History
Patients with stress fractures give a classic history of insidious onset of pain in a localized area. The pain is exacerbated by repetitive activity and relieved by rest. Symptoms persist from weeks to months. As the injury progresses, the pain will occur earlier and with greater intensity during activity. A training error may relate to the onset of symptoms. Training errors include changes in distance, intensity, or frequency of workout. On occasion there may be change in the running surface or shoes. Inadequate footwear can also contribute. In athletes with exertional bone pain, 50% will have a stress fracture (7).
Physical Exam
Stress fractures will result in point tenderness over the affected bone. Patients will generally have a full range of motion at all joints, with normal muscle tone and function. Neurovascular function is normal. There may be some subtle soft tissue swelling in the foot. Running, walking, or toe walking may recreate the symptoms.
Imaging
Standing x-rays are the first line of imaging in these injuries. Most x-rays will initially be negative. Baseline films on presentation will allow recognition of radiographic changes later in the course of the injury. After several weeks long bones such as the metatarsals or tibia will show periosteal new bone formation or cortical break. Cancellous bone such as the calcaneus or tarsal bones will show medullary sclerosis. A bone scan is the investigation of choice, and it will be positive as early as 3 days after injury (7). The bone scan will be positive in all three phases with a focal intense area of uptake, even in the setting of normal x-ray findings (3). The bone scan is virtually 100% sensitive in diagnosing stress fractures, but its specificity is less than x-ray (4). Bone scan cannot distinguish stress fracture from neoplasm or infection (7). Computed tomography (CT) scans have a role in evaluation of navicular, calcaneal, tibial, and pediatric fatigue fractures. Magnetic resonance imaging (MRI) can also be a useful adjunct in some circumstances with negative films and equivocal bone scan.
Forefoot
Sesamoids
Stress fractures of the sesamoids, although uncommon, do occur. The sesamoids are subject to injury due to their positioning under the head of the first metatarsal. Up to 50% of body weight is born through the great toe complex (12). They impart mechanical advantage to the flexor hallucis brevis tendons by acting as a fulcrum. The medial sesamoid may be more frequently injured, although in one study the medial and lateral sesamoids were found to be involved equally (13,14).
Patients with stress fractures of the sesamoids will present with the classic history of a stress fracture. Patients will have pain standing on their toes or with dorsiflexion of the first toe. The differential diagnosis of pain in the first metatarsophalangeal joint includes sesamoiditis, chondromalacia, stress fracture, osteochondritis, turf toe, hallux valgus, medial plantar digital proper nerve syndrome, and bursitis (15,16).
Standing anteroposterior (AP) and lateral foot x-rays and a sesamoid view will evaluate the sesamoids (13). If a bipartite sesamoid is seen, a bone scan will be cold with a multipartite sesamoid and will show increased uptake with a stress fracture (15). In a histological study of the stress fractured sesamoid by
Van Hal et al. (14), the cellular structure of the stress fracture resembled a pseudoarthrosis (14).
Van Hal et al. (14), the cellular structure of the stress fracture resembled a pseudoarthrosis (14).
Initial treatment of a stress fracture of a sesamoid involves 6 weeks of immobilization in a cast or walker boot. Diebold has immobilized the toe in flexion using a K wire to achieve bone healing. After 6 weeks the foot is re-examined and x-rays taken. If unhealed, surgery may be considered. Surgical excision of one sesamoid may be considered, particularly if the sesamoid is fragmented (14) (Fig 51-1). If both sesamoids are excised the patient will have an unacceptable result of a cock-up-toe deformity, and this is highly discouraged (13). Partial excision of the smaller fragment can also be performed. Bone grafting of a well-defined fracture nonunion may be considered. If at the time of surgery there is no gross motion at the nonunion site, it is recommended that the nonunion be bone grafted. If there is gross motion the sesamoid should be excised as reported by Anderson et al. (12).
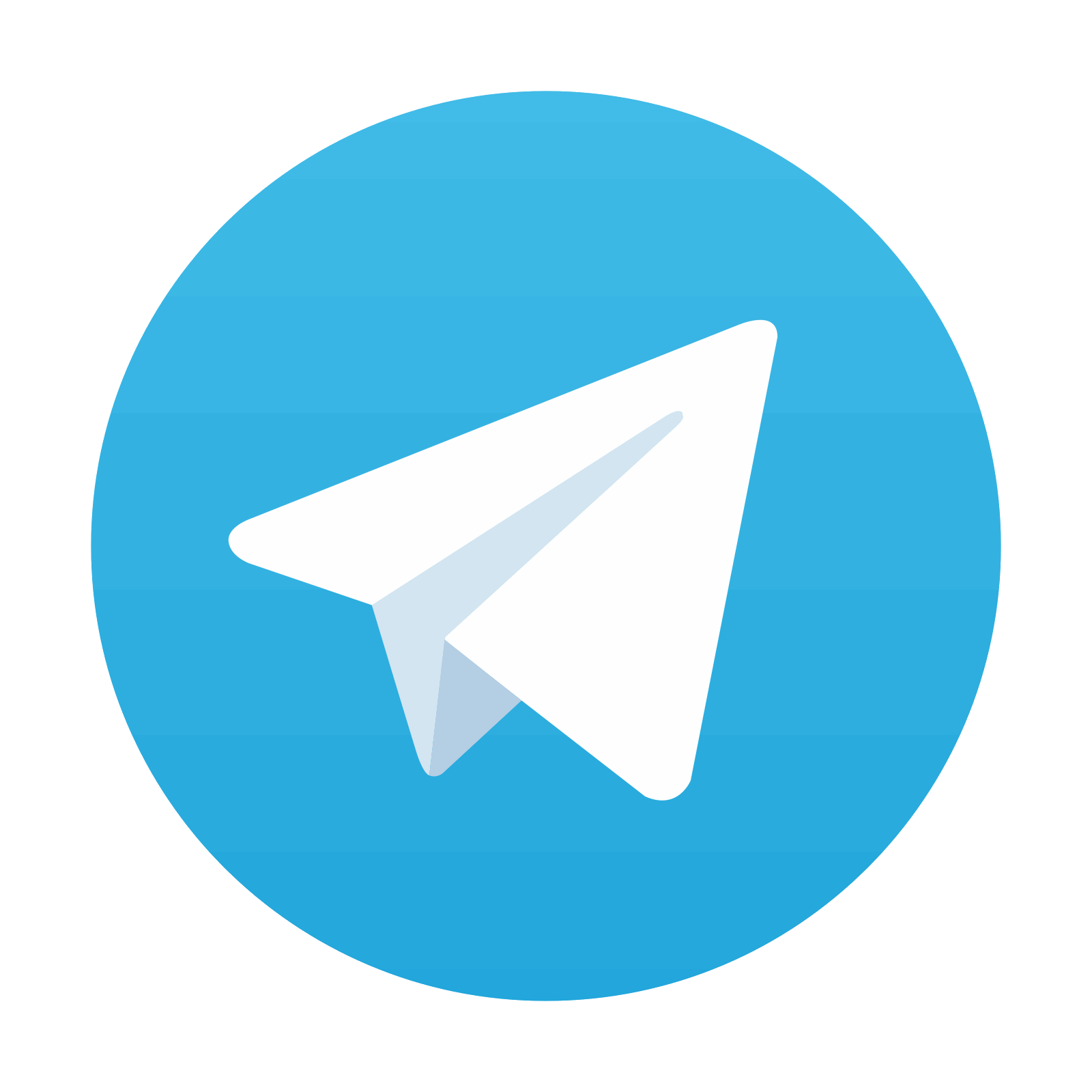
Stay updated, free articles. Join our Telegram channel

Full access? Get Clinical Tree
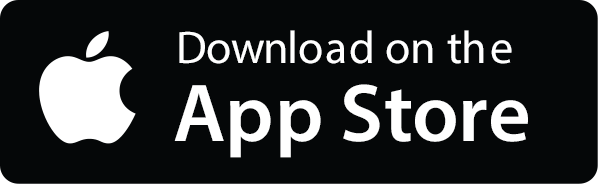
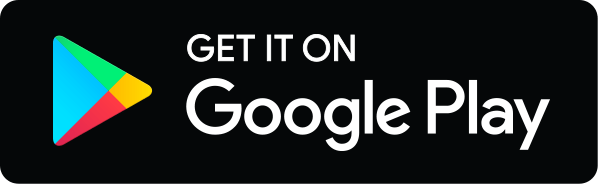
