X-ray
MRI
Treatment
Grade 1
Normal
Positive on STIR image only
3 weeks rest
“Stress reaction”
Grade 2
Normal
Positive STIR plus positive T2
3–6 weeks rest
“Stress injury”
Grade 3
Discrete periosteal reaction
Positive STIR, T1 and T2, without definite fracture line
12–16 weeks rest
“Stress fracture”
Grade 4
Periosteal reaction and/or fracture
Positive STIR, T1 and T2 plus fracture line
16+ weeks rest
“Stress fracture”
Epidemiology
Stress fracture is a relatively uncommon injury in the general populace. According to a large cross-sectional survey of adolescents, the lifetime prevalence was below 4 % [5]. Estimates of the burden of injury are, therefore, widely variable when studying smaller groups. Fifteen percent of all reported stress fractures are sustained by distance runners, in whom that diagnosis accounts for half of all injuries [6]. A large study of intercollegiate athletes noted twice the incidence of stress fracture among women compared to men [7]. In studies of military populations, the rate ratio has been reported as high as 10 to 1 [8–12], with up to 20 % of female recruits in basic training sustaining a stress fracture [9–13]. In the broader population of ambulatory adolescents and young adults, no such gender difference has been noted [14]. Otherwise, studies in adult populations have shown increased prevalence with age [8, 11], and Caucasian or Asian ethnicity [11].
Risk Factors
Prevention of stress fracture, as with any injury, starts with identification of risk factors. Low injury rates make definitive determination of risks challenging. Many authorities categorize factors as either intrinsic to the athlete or related to external exposures (extrinsic). In either category, only some elements are modifiable. In addition, a study of nearly 900 United States Military Academy (USMA) cadets developed a multivariable model in which the maximum variance in risk of stress fracture explained by all potential predictors was only 10 % [9].
Extrinsic Factors
Among the putative causes for stress fracture are factors related to the bone-loading activities, including training surface, techniques, equipment including footwear, and coaching. By far the greatest factor is training volume, which incorporates intensity and quantity, the latter of which is more easily studied. In the Growing Up Today Study (GUTS), a cross-sectional survey of 5,461 daughters of the nurses participating in the national Nurses’ Health Study II, engaging in greater than 16 h/week of moderate-to-vigorous physical activity conferred a 79 % increased odds of reporting a history of stress fracture. Every hour of high impact physical activity was associated with an odds ratio (OR) for stress fracture of 1.05 (95 % confidence interval, CI: 1.02–1.09), with running (OR 1.12, 95 % CI: 1.05–1.21) and cheerleading/gymnastics (OR 1.12, 95 % CI: 1.03–1.21) the most significant culprits [5]. A subsequent prospective study of a similarly derived cohort of 6,831 girls refined the findings, with both 12–16 h/week of such activity (hazard ratio, HR 2.78, 95 % CI: 1.41–5.47) and 16–20 h/week (HR 2.65, 95 % CI: 1.37–5.12) associated with even greater risk of stress fracture(s). Running and cheerleading/gymnastics showed the same 12 % increased risk of injury for every hour of participation, as did basketball, in this sample, with hours of any high impact activity associated with an HR of 1.08 (95 %CI: 1.05–1.12) [15].
Intrinsic Factors
Biomechanical factors such as lower extremity alignment, including the so-called Q-angle formed at the knee by the axes of the femoral and tibial shafts; hip, knee, and ankle flexibility; mid-foot hyper-pronation; constitutional hypermobility; and core and lower extremity strength have all been examined for potential associations with stress fracture, without consistent findings. Calf girth measurements have shown promise related to tibial stress fractures, probably due to the protective effect the calf muscles can provide the tibia in absorbing repetitive ground reactive forces in running [16].
The interplay between muscle and bone is also demonstrated by the few studies that attempt to quantify overall physical fitness. In male USMA cadets, those who exercised <7 h/week in the year prior to entry had twice the risk of sustaining a stress fracture than those who trained more [9]. Among women recruits entering the Army, those who failed a pilot 5-min step test for fitness at pre-entry examination had a 76 % increased incidence of stress fracture during training [17]. Examination of female US Marine Corps recruits identified low aerobic fitness and <7 months of pre-boot camp lower-extremity weight training as risk factors for stress fracture in logistic regression modeling [18], while in a large cohort of 2,345 Finnish women military personnel, poor muscle strength and a poor result in a 12 min run were associated with bone stress injuries [8]. An interesting finding associated the subjective report of “burnout” with stress fracture in female Israeli military recruits in basic training [19]. A systematic review of stress fractures in both athletic and military populations speculated that overall physical fitness is more important to injury risk than other factors, with the observed increased prevalence in women possibly attributable to poorer pre-activity fitness, which has typically not been measured, either in studies or routine pre-participation evaluations [10].
In a case-control study of female adolescents presenting to a sports medicine clinic with stress fractures prospectively diagnosed by radiographs, reported family history of osteoporosis or osteopenia was the only significant predictor, with an OR of 2.96 (95 % CI: 1.36–6.45), controlling for all other important potential risk factors [20]. The prospective GUTS mentioned above [15] confirmed this finding, as did a large cross-sectional analysis of 2,312 active duty army women, which also identified white race as a risk factor for stress fracture [21]. Most other studies have not examined this covariate. Few small studies have examined polymorphisms in the estrogen receptor, androgen receptor, lactase [22], and vitamin D receptor (VDR) genes, with only polymorphisms in VDR significantly associated with stress fracture in male military personnel [23].
Bone Resilience
Most research attention has been paid to the intrinsic ability of the skeleton to withstand the repeated stresses that cause injury. Examinations of bone geometry have demonstrated an expansion of cross-sectional area and cortical thickness related to the ground reactive forces of running and jumping, but thresholds have not been established that correlate with stress fracture [24]. Increased use and standardization of advanced imaging techniques like peripheral quantitative computed tomography (pQCT) may allow for better understanding of the geometric and architectural parameters that can resist stress fracture.
Our greatest understanding of bone quality, therefore, comes from investigations of bone mineral density (BMD). Starting with Myburgh’s sentinel 1990 matched case-control study of 38 adult female runners, there has been a growing body of consistent evidence that at least some stress fractures are associated with decreased BMD [25]. This literature includes retrospective [26] and prospective studies of American [27–29] and international [16] track and field athletes as well as American [21] and Israeli [19] female soldiers. The association between low BMD and stress fracture persists in meta-analyses [30].
Menstrual Function
Menstrual history has been a less consistent predictor of stress fracture in studies examining this factor. The GUTS cohort studied in 2005 demonstrated that being post-menarcheal was protective against stress fracture, with an OR 0.61 (95 % CI: 0.40–0.93) in an adjusted multivariable model [5]. The subsequent prospective GUTS estimated at least a 30 % increased risk (HR) of stress fracture for every year later age of menarche [15]. In addition to family history of stress fracture, Friedl’s large military study identified history of amenorrhea as a risk factor for stress fracture [21]. A prospective analysis of female USMA cadets demonstrated a 44 % decrease in stress fracture risk for every additional year since menarche [9]. The association with delayed menarche was first noted by Warren et al. in ballet dancers in 1986 [31]. In addition to delayed menarche [16, 26] conferring increased risk, most [16, 18, 21, 25, 28, 32, 33], but not all [20, 27, 29] cohort and case-control studies have found an association between a variety of measures of current menstrual function and stress fracture.
Nutrition
Although the association of athletic energy availability with risk of stress fracture has not been studied, general nutritional status is associated with injury, with active females who are underweight (e.g., <75 % ideal [34]) having increased rates [11]. In women soldiers, lowest adult weight is associated with risk of stress fracture (although not current weight) [35]. In the prospective matched case-control study performed in Boston, increased BMI was associated with odds of stress fracture, perhaps as a proxy for decreased fitness levels, although the effect was mitigated and not significant in multivariable models [20]. Field’s large prospective GUTS cohort did not demonstrate any significant association between stress fracture and being either overweight or underweight [15]. Moreover, positive responses to disordered eating behaviors by these girls on the Youth Risk Behavior Surveillance System questionnaire did not show any association with stress fracture [15].
Other studies have associated elevated cognitive dietary restraint ratings (although not frank disordered eating) [26, 36, 37] and increased eating disordered inventory (EDI) scores [38] with impaired BMD in active female adolescents, independent of menstrual history. Few have directly linked elements of disordered eating with stress fracture, perhaps due to the low incidence of this injury in most studied cohorts, although one study did associate self-reported stress fracture with measures of compulsive exercise, controlling for global scores on the Eating Disorder Examination Questionnaire (EDE-Q) [39].
Dietary intake of the primary macro- (dairy) and micro-nutrients (calcium and vitamin D) associated with bone health have not shown consistent associations with stress fracture risk. Myburgh’s early study did demonstrate lower dietary calcium and dairy intake in cases with stress fracture, but a review of the literature showed that most cross-sectional and population-based studies do not support this finding [40, 41]. However, two prospective studies, including a randomized clinical trial (RCT) in female navy recruits [42], demonstrated a protective effect of increased current dietary calcium intake [42, 43]. Among young adult female competitive distance runners, higher intakes of skim milk and other dairy products were also protective [43]. Vitamin D has been less well studied, although the naval RCT also supplemented vitamin D to 800 IU daily over 8 weeks [42]. Among adolescents, a prospective analysis of food frequency questionnaires of the participants in the GUTS cohort demonstrated a protective effect among those in the highest quintile of vitamin D intake (HR 0.48, 95 % CI: 0.22–1.02, p = 0.04 for trend), stratifying for at least 1 h of weekly moderate-to-vigorous physical activity [44].
Clinical Applicability
As addressed elsewhere in this text, assessment of nutritional status and menstrual history constitute essential health promotion for female athletes, with attention to correct any identified deficits, especially in those who have sustained a bony injury. The dilemma posed to the clinician managing a patient with stress fracture is therefore whether to evaluate BMD. In 2007 the International Society for Clinical Densitometry developed guidelines to help identify children and adolescents who might warrant skeletal assessment by dual emission X-ray absorptiometry (DXA). These guidelines defined “clinically significant fractures” as
Any long bone fracture of the lower extremities
Vertebral compression fractures
Two or more long bone fractures of the upper extremities
but did not specifically address stress fractures [45].
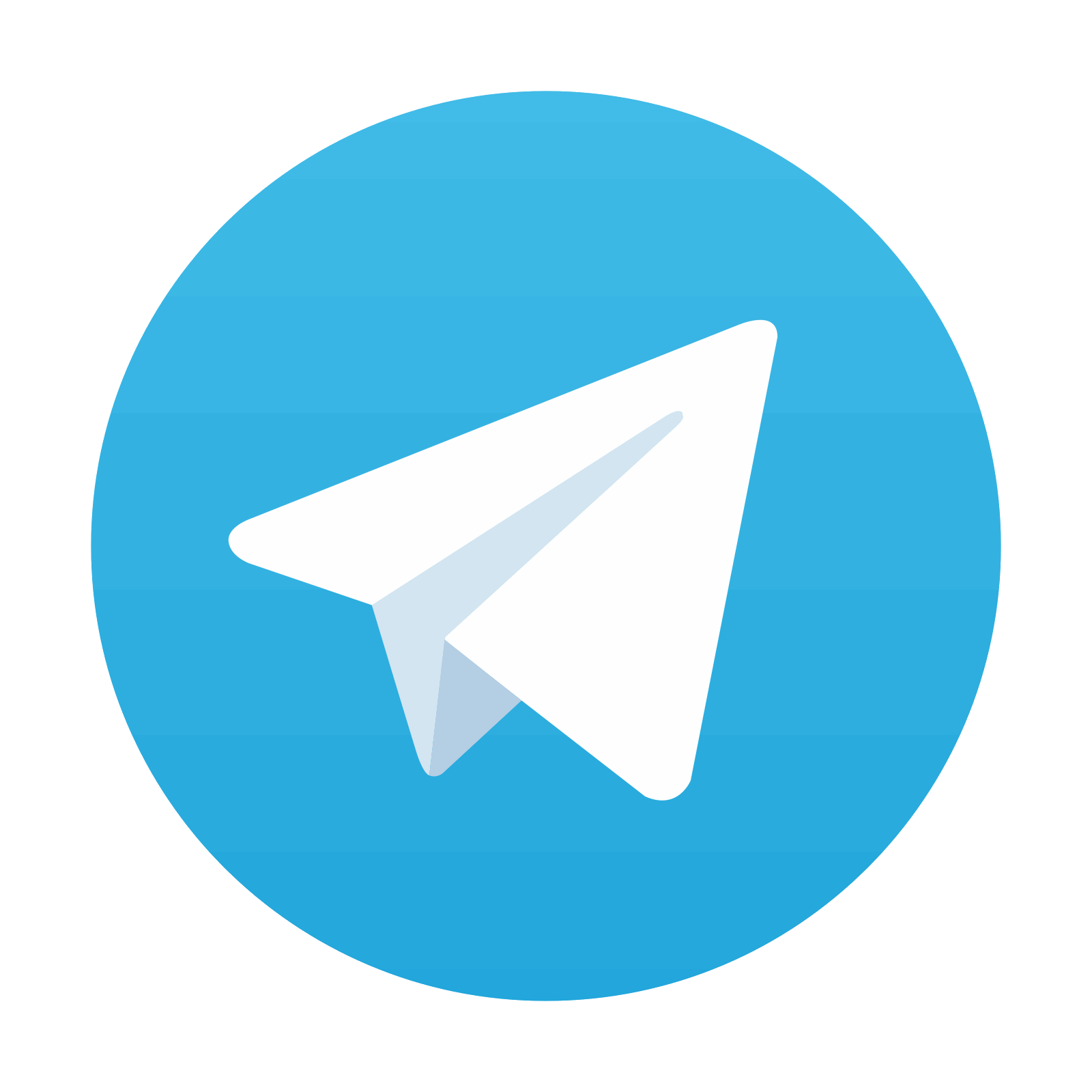
Stay updated, free articles. Join our Telegram channel

Full access? Get Clinical Tree
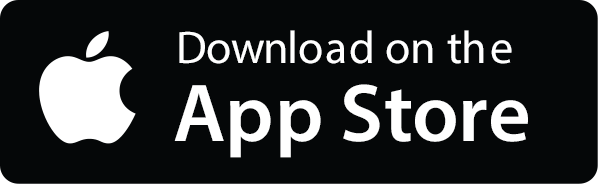
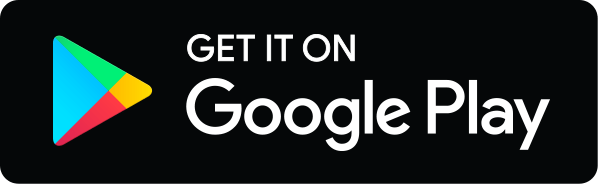