2 Proposed mechanisms and effects of trigger point dry needling
Introduction
Many physical therapists and other clinicians have adopted a contemporary pain management approach and incorporate graded exercise, restoration of movement and posture and psychosocial perspectives into the examination, assessment and therapeutic interventions of patients presenting with pain complaints (Gifford & Butler 1997, George et al. 2010, Nijs et al. 2010, Hodges & Tucker 2011). The question emerges whether movement approaches by themselves are sufficient to address persistent pain states without eliminating peripheral nociceptive input?
Current pain science research supports that pain is produced by the brain, when there is a perception of bodily danger requiring specific action (Moseley 2003). In other words, the ‘issues are not just in the peripheral tissues’ (Butler 1991) and considering the meaning of pain in the context of the patient’s overall situation is critical (Moseley 2012). The effects of trigger point (TrP) dry needling (DN) cannot be considered without this broader biopsychosocial model (Gerwin & Dommerholt 2006). TrP DN must be approached from a pain science perspective, as it is no longer sufficient to consider TrP therapy strictly as a tool to address local muscle pathology.
As Moseley pointed out, nociceptive mechanisms that contribute to threatening information should be treated, where possible (Moseley 2003). Frequently, TrPs are a constant source of nociceptive input especially in persistent pain conditions (Giamberardino et al. 2007, Melzack 2001 Ge et al. 2011) and it follows that removing such peripheral input is indicated and consistent with the concepts of Melzack’s neuromatrix (Melzack 2001). In addition to their contribution to nociception, TrPs can contribute to abnormal movement patterns (Lucas et al. 2004, 2010).
Input from muscle nociceptors appears more effective at inducing neuroplastic changes in wide dynamic range dorsal horn neurons than input from cutaneous nociceptive receptors (Wall & Woolf 1984). Several studies demonstrated that TrPs activate the anterior cingulate cortex (ACC) and other limbic structures, but suppress hippocampal activity (Svensson et al. 1997, Niddam et al. 2007, 2008). Increased activity in the ACC is common in chronic pain conditions and is even present when pain is anticipated (Hsieh et al. 1995, Peyron et al. 2000a, 2000b, Sawamoto et al. 2000, Longo et al. 2012). When treating patients with DN techniques, it is imperative to avoid creating the impression that local muscle pathology would be solely responsible for the persistent pain (Nijs et al. 2010, Puentedura & Louw 2012). Rather than explaining TrPs as a local pathological or anatomical problem, it makes more sense to focus on the nociceptive nature of TrPs and their role in perpetuating central sensitization. Persistent peripheral nociceptive input increases the sensitivity of the central nervous system. Unfortunately, the contributions of TrPs often are not considered and individual patients may have gone through many unsuccessful treatment regimens with multiple diagnostic pathways. They may have developed fear avoidance or kinesiophobia, poor coping skills, and an anticipation of pain (Bandura et al. 1987, Vlaeyen & Linton 2000, Wager et al. 2004, Coppieters et al. 2006). Additionally, patients’ altered homeostatic systems may start contributing to the overall pain experience (Puentedura & Louw 2012) with decreased blood flow to the muscles (Zhang et al. 2009), abnormal cytokine production (Watkins et al. 2001, Milligan & Watkins 2009), constrained breathing patterns (Chaitow 2004), and abnormal muscle activation patterns (Moreside et al. 2007), among others. In some patients, the anticipation of pain and the pain associated with DN itself may activate threatening inputs, at which point DN becomes counterproductive. Fortunately, this is quite rare and for most patients TrP DN is a viable intervention (Dilorenzo et al. 2004, Affaitati et al. 2011).
Mechanisms and effects of trigger point dry needling
There are no studies of the effect of DN on the ACC and other limbic structures, but several papers suggest that needling acupuncture and non-acupuncture points does seem to involve the limbic system and the descending inhibitory system (Takeshige et al. 1992a, 1992b, Wu et al. 1999, Biella et al. 2001, Hsieh et al. 2001, Hui et al. 2000, Wu et al. 2002). DN studies of patients with fibromyalgia, which is a diagnosis of central sensitization (Clauw 2008, Dommerholt & Stanborough 2012), demonstrate that DN of a few TrPs does not only reduce the nociceptive input from the treated TrPs, but reduces the overall widespread pain and sensitivity (Ge et al. 2009, 2010, 2011, Affaitati et al. 2011). TrP DN often evokes patients’ referred pain patterns and their primary pain complaint (Hong et al. 1997). Needling of TrPs in the gluteus minimus or teres minor muscles may initiate pain resembling a L5 or C8 radiculopathy, respectively (Escobar & Ballesteros 1988, Facco & Ceccherelli 2005). Needling of TrPs in the sternocleidomastoid or upper trapezius muscles may trigger a patient’s migraine or tension-type headache (Calandre et al. 2006). Experimentally induced muscle pain impairs diffuse noxious inhibitory control mechanisms (Arendt-Nielsen et al. 2008) and DN does seem to effect central sensitization, presumably by altering the nociceptive processing (Kuan et al. 2007a, Mense 2010, Mense & Masi 2011). It is known that TrP DN reduces segmental nociceptive input and as such is therapeutically indicated (Srbely et al. 2010).
This chapter could end here, because the exact mechanisms of DN continue to be elusive. Since many studies and case reports have confirmed the clinical efficacy of DN, future research must be directed towards examining the underlying mechanisms (Lewit 1979, Carlson et al. 1993, Hong 1994, 1997, Hong & Hsueh 1996, McMillan et al. 1997, Chen et al. 2001, Cummings 2003, Mayoral & Torres 2003, Dilorenzo et al. 2004, Ilbuldu et al. 2004, Itoh et al. 2004, 2007, Lucas et al. 2004, Furlan et al. 2005, Kamanli et al. 2005, Mayoral-del-Moral 2005, Weiner & Schmader 2006, Giamberardino et al. 2007, Hsieh et al. 2007, Fernandez-Carnero et al. 2010, Lucas et al. 2010, Osborne & Gatt 2010, Tsai et al. 2010, Srbely et al. 2010, Affaitati et al. 2011). Slowly, bits and pieces of the myofascial pain puzzle are beginning to be explored.
Mechanically, deep DN may disrupt contraction knots, stretch contractured sarcomere assemblies and reduce the overlap between actin and myosin filaments. It may destroy motor endplates and cause distal axon denervations and changes in the endplate cholinesterase and acetylcholine receptors similarly to the normal muscle regeneration process (Gaspersic et al. 2001). Of particular interest are local twitch responses (LTR), which are involuntary spinal cord reflexes of muscle fibers in a taut band following DN, injections, or snapping palpation (Dexter & Simons 1981, Fricton et al. 1985, Hong 1994, Hong & Torigoe 1994, Wang & Audette 2000, Ga et al. 2007). Eliciting LTR is important when inactivating TrPs and confirms that the needle was placed accurately into a TrP. Several studies have confirmed that a LTR can reduce or even eliminate the typical endplate noise associated with TrPs which suggests that DN inactivates TrPs (Hong & Torigoe 1994, Hong 1994, Chen et al. 2001). There is a positive correlation between the prevalence of endplate noise in a TrP region and the pain intensity of that TrP (Kuan et al. 2007b). Endplate noise is a summation of miniature endplate potentials and is a characteristic of TrPs (Simons et al. 1995, 2002, Hong & Simons 1998, Simons 2001, Simons 2004). Moreover, eliciting LTRs appears to reduce the concentrations of many chemicals found in the immediate environment of active TrPs, such as calcitonin gene related peptide, substance P, serotonin, interleukins, and epinephrine, among others (Shah et al. 2003, 2005, 2008, Shah & Gilliams 2008). Shah et al. (2008) had speculated that the drop in concentrations may be caused by a local increase in blood flow or by interference with nociceptor membrane channels, or by transport mechanisms associated with a briefly augmented inflammatory response. The decrease of concentrations of substance P and calcitonin gene-related peptide corresponds with the clinical observation of a reduction in pain following deep DN (Shah et al. 2008). LTRs are often visible with the naked eye and can be visualized with sonography (Gerwin & Duranleau 1997, Lewis & Tehan 1999, Rha et al. 2011).
The effects of superficial DN are often attributed to stimulation of Aδ sensory afferent fibers, which may outlast the stimulus for up to 72 hours (Baldry 2005). It is true that stimulation of Aδ nerve fibers may activate enkephalinergic, serotonergic, and noradrenergic inhibitory systems (Bowsher 1998); however, type I high-threshold Aδ nerve fibers are only activated by nociceptive mechanical stimulation and type II Aδ fibers require cold stimuli (Millan 1999). Since superficial DN is neither a painful mechanical nor a cold stimulus, it is unlikely that Aδ fibers would get activated (Dommerholt et al. 2006). When superficial DN is combined with rotation of the needle, the stimulus may activate the pain inhibitory system associated with stimulation of Aδ fibers through segmental spinal and propriospinal hetero-segmental inhibition (Sandkühler 1996). Deep DN can be also combined with rotation of the needle, after which the needle is left in place until relaxation of the muscle fibers has occurred (Dommerholt et al. 2006). The mechanical pressure exerted with the needle may electrically polarize muscle and connective tissue, and transform mechanical stress into electrical activity, which is required for tissue remodelling (Liboff 1997). It is also possible that superficial DN may activate mechanoreceptors coupled to slow conducting unmyelinated C fiber afferents. This could trigger a reduction of pain and a sense of progress and well-being through activation of the insular region and anterior cingulate cortex (Olausson et al. 2002, Mohr et al. 2005, Lund & Lundeberg 2006).
Many clinicians combine superficial and deep DN with electrical stimulation through the needles (Mayoral & Torres 2003, Mayoral-del-Moral 2005, Dommerholt et al. 2006), which may activate the peri-aqueductal grey in some patients (Niddam et al. 2007). Unfortunately, there are no evidence-based guidelines of the optimal treatment parameters, such as optimal amplitude, frequency, and duration. Stimulation frequencies between 2 and 4 Hz are thought to trigger the release of endorphins and encephalin, while frequencies between 80 and 100 Hz may release gamma-aminobutyric acid, galanin and dynorphin (Lundeberg & Stener-Victorin 2002). Several rodent studies have shown that electrical acupuncture can modulate the expression of N-methyl-d-aspartate in primary sensory neurons (Choi et al. 2005, Wang et al. 2006). The ideal needle placement for e-stim with DN has not been determined either, although White et al. (2000) recommended placing the needle-electrodes within the same dermatomes as the location of the lesion.
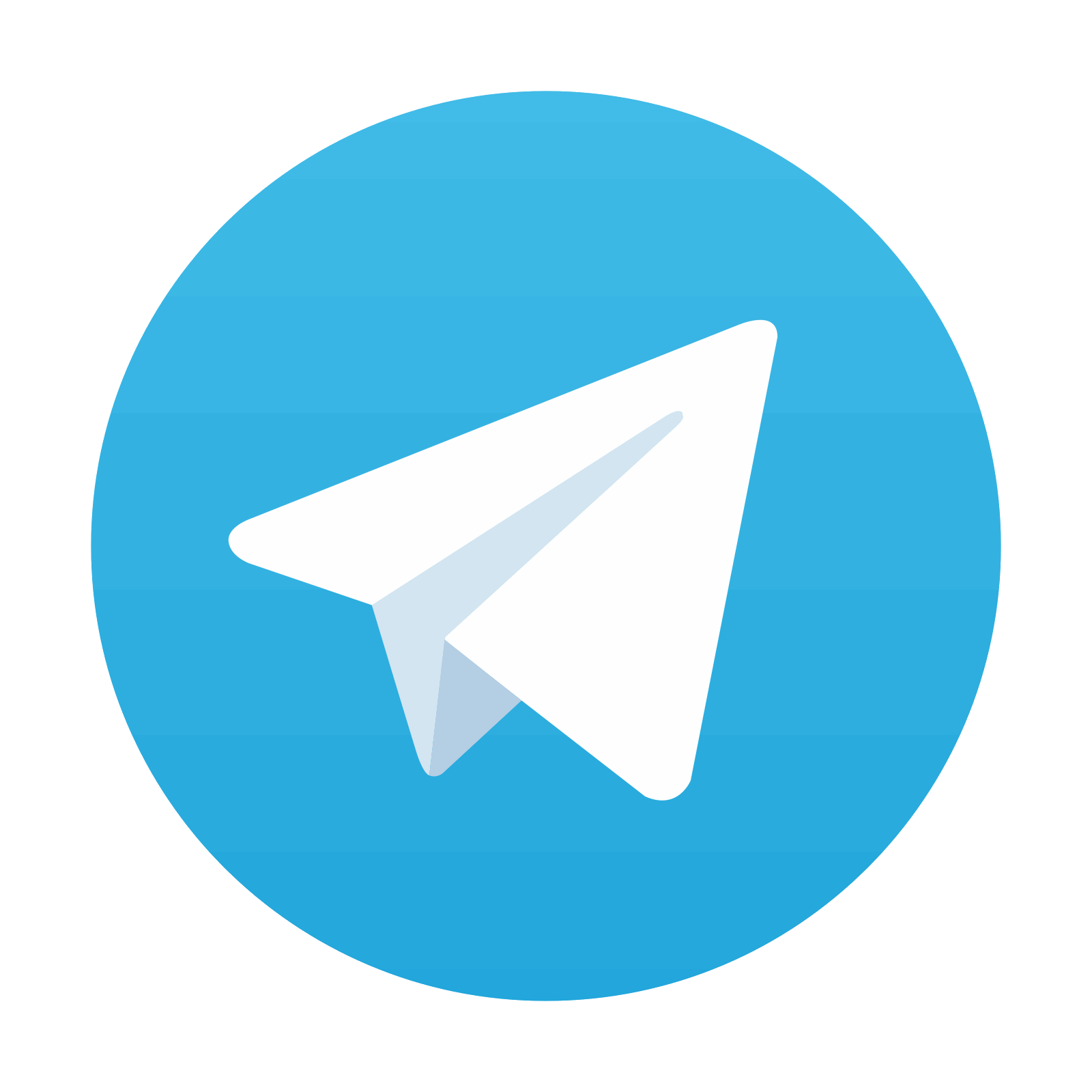
Stay updated, free articles. Join our Telegram channel

Full access? Get Clinical Tree
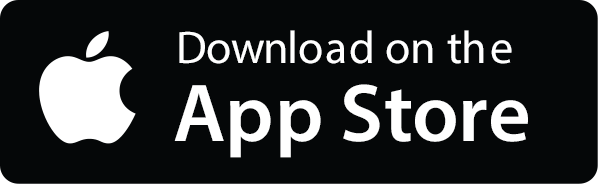
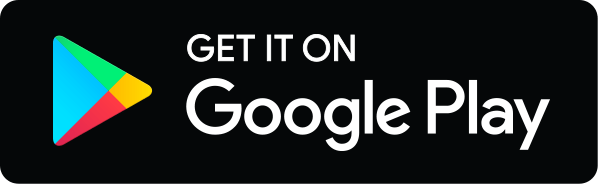