24
Peripheral Nerve Problems
Introduction
There is an ongoing plasticity within our nervous system. This means the nervous system can change, learn, and adapt or maladapt throughout the individual’s life span. Sensory receptors of peripheral nerves will become less sensitive to stimuli that is not harmful to the body if the stimuli is present for a long enough period of time. For example, if you walk into a room where there is a strong odor, say a baby’s diaper, your olfactory sensory receptors will eventually habituate, or decrease their responsiveness to this smell. Your PNS has temporarily changed its sensitivity to this odor. Still, if you leave the room and come back later, the strength of the stimulus (the bad smell) returns. The intensity of that stimulus is the same as when you entered the room the first time; your habituation to the stimulus was short lived. On the other hand, if your nervous system is exposed to that stimulus for a long enough time, your nervous system could alter, modulate, or permanently change. A gradual and long-lasting change in both the CNS and PNS is called modulation. Your sensitivity to a strong odor might permanently decrease (or increase) depending upon your nervous system’s attempt to maintain homeostasis. The capacity of our nervous system to modulate or change permanently is called neuroplasticity. This is the cornerstone of neurorehabilitation.1
Likewise, if there is an injury to a peripheral nerve (PNS) (for example, let’s say the median nerve), there would be muscle paralysis and loss of sensory feedback to the CNS. In this case, the patient would not be able to actively position his thumb in opposition to grab the cup handle due to paralysis of the opponens pollicus, nor would he be able to feel if the cup was too hot to handle safely. Such deficits in sensorimotor control lead the brain to rapidly attempt to reorganize to the reduction in sensory input and motor output.2 The CNS will quickly reorganize its cortical representations of the hand following an alteration of skin sensory inputs induced by a peripheral nerve injury.2 Neglect of a body part (for example, following a nerve injury with consequent motor paralysis) will also lead to changes in cortical organization. In fact, how the cortex reorganizes following a peripheral nerve injury has been proposed as a major factor in final functional outcomes, including whether or not our patient will develop chronic pain.2–5
Overview of Neuroanatomy
The CNS is well-organized with an intricate system of motor and sensory pathways arranged to reflect and respond to the continual input of information from our interaction in the environment. Cortical information is processed somatotopically with a homunculus or mapping in both the frontal lobe (for the motor pathways) and the parietal lobe (for sensory pathways) to specifically handle compartmental information and assure very quick feedback for movement and sensory integration. This cortical representation or homunculus mapping in the brain is arranged to reflect the amount of neuronal input and output demand; thus, the hand is represented as a much bigger part of the homunculus than is the elbow.5
The sensory and motor roots join together to form a spinal nerve (Fig. 24-1). Sympathetic nerve axons from autonomic ganglia also join the spinal nerve by way of the rami communicans. Therefore, spinal and somatic peripheral nerves are usually mixed with sensory, autonomic, and motor axons. Shortly after merging, the spinal nerve splits into dorsal and ventral rami. The ventral rami of all spinal nerves, except for T2 to T12, form networks of nerves called plexuses. There are four main plexuses; the largest are the brachial plexus and lumbar plexus.
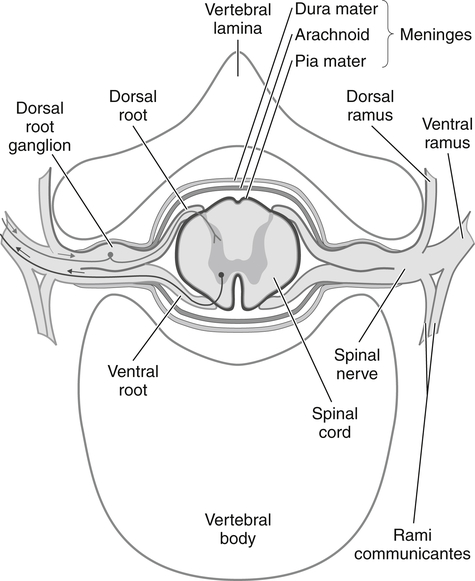
The brachial plexus (Fig. 24-2) is formed by the anterior rami of cervical roots C5 to T1. This plexus emerges from the anterior and middle scalene muscles and passes deep to the clavicle before entering the axilla. In the distal axilla, the axons from the plexus become the radial, median, ulnar, axillary, and musculocutaneous nerves. The entire upper limb is innervated by branches from this brachial plexus.
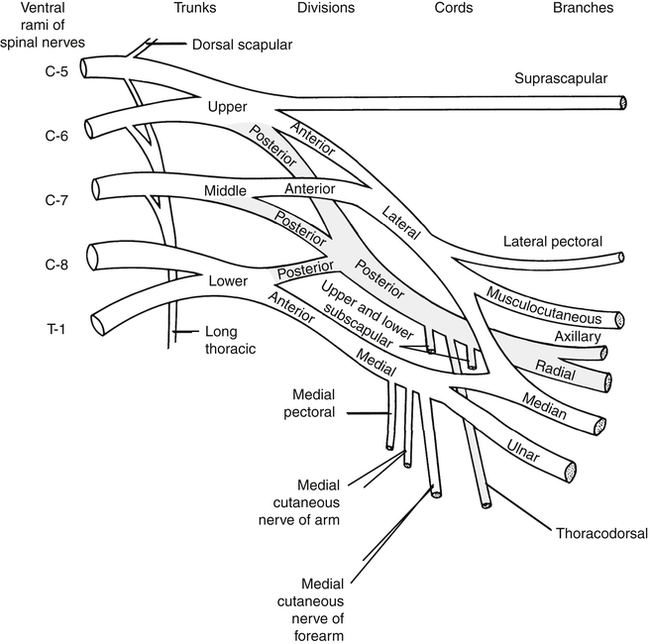
The peripheral nerve is composed of many very long cells that originate in the spinal region and progress distally in bundles, surrounded by multiple tissue coverings. These nerve cells are designed to be conduits for transmitting impulses—no matter how or in what position the body moves. We know that joints move in a matter of degrees, that muscles contract, and that tendons slide. Nerves also move; they move relative to the tissues that surround them.4 The nerve cell itself must stretch and slide within its protective tissue coverings, and the nerve trunk must glide relative to the surrounding external tissues while continuing to perform its essential duty, impulse conduction.
The normal nervous system is designed to protect its neurons and their peripheral axons during movement. It has been speculated that the gliding of the peripheral nerves across our joints and through anatomical tunnels promotes axoplasmic flow and enhances blood circulation to neural tissue.4 However, certain movements or postures can increase the amount of pressure within the nerve trunk, and if enough pressure is placed on the nerve trunk, temporary anoxia from diminished blood flow to the nerve will result. We’ve all experienced our foot “going to sleep” after sitting with our legs crossed for too long. In this scenario, a local compression of the peroneal nerve that runs behind the knee results in a temporary loss of blood flow to that nerve. This results in transitory sensory loss and brief muscle paresis. As blood flow is restored to this nerve, we experience pins and needles, burning, and a gradual return in ability to weight bear on our involved leg. However, given the right circumstances, this type of innocuous insult can turn into significant nerve pathology.
Axoplasmic flow within the nerve’s axon allows for a constant and controlled flow of substances that serves to maintain neural health and allow the nerve to function normally through electrochemical messaging.1,6,7 There is a constant flow of materials in each nerve fiber from the nerve cell body to its end organs (anterograde flow) and from the end organs in the muscles or sensory receptors to the nerve cell body (retrograde flow). Various substances and organelles are synthesized in the nerve cell body and are transported within the axon to and from its terminal. This requires a continuous energy supply of oxygenated blood flow. Compromised blood flow to a nerve will result in diminished axoplasmic flow. Impaired axoplasmic flow will lead to compromised neural function, including slowed and/or decreased synaptic activity, and trophic changes in the tissues served by that nerve.4,8,9
Peripheral nerves are ensheathed, protected, and at times constrained by multiple layers of connective tissue coverings: the endoneurium, perineurium, epineurium, and mesoneurium. These coverings create a nerve “bed.” Within this nerve bed, individual nerve fibers sit gently coiled in a wavy pattern. These fibers change position within the nerve bed, frequently entwining and separating into different fascicles as they course in an undulating fashion to their final destination. This meshing serves as a protective mechanism, allowing some play in the overall length of the nerve9 (Fig. 24-3). Thus, peripheral nerve fibers are capable of some degree of elastic stretch, or compression, allowing the longitudinal length difference needed when a joint moves into an end range posture. The ulnar nerve, for example, must stretch around the medial epicondyle of the humerus during full elbow flexion, and adapt to the slack or shortening that occurs during full elbow extension. This elongation and slack uptake is distributed evenly throughout a healthy peripheral nerve.9
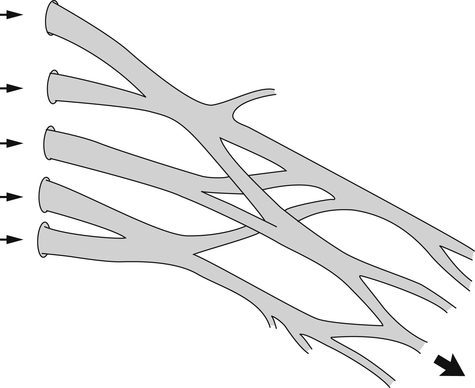
Generally, peripheral nerves are protected from injury by their external protective connective tissue coverings (Fig. 24-4). The outermost covering, the epineurium, functions to surround and cushion nerve fascicles, especially in locations where the peripheral nerve is vulnerable to compression.9,10 There is a greater percentage of epineural tissue present where the nerve is more superficial and/or located near a joint. For example, the ulnar nerve (because it traverses under the medial epicondyle of the humerus) is typically protected from contusions and compression by the large percentage of epineurial tissue present in that part of the nerve. The deeper layers of epineurium function like packing material to protect the fascicular groups of nerve fibers. Each fascicle is surrounded by a mechanically strong sheath called the perineurium. This connective tissue layer serves as a diffusion barrier, helping to preserve the specialized microenvironment inside the fascicles.
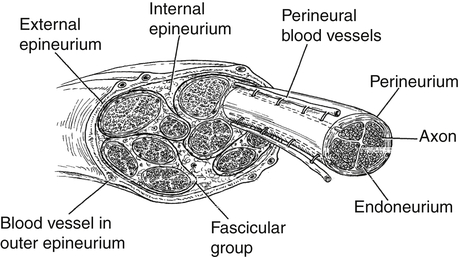
The fibers inside the fascicles are embedded in a loose connective tissue called the endoneurium, or basement membrane. Around each nerve fiber (or axon), however, the endoneurium becomes closely packed, forming a supporting wall. The endoneurium serves to electrically insulate individual nerve fibers from each other. Some of these nerve fibers are myelinated. A myelinated nerve fiber is one that has a longitudinal chain of concentrically wrapped Schwann cells surrounding it, creating an insulating tube that promotes fast impulse conduction. The Schwann cell tissue together with the closely packed endoneurial tissue constitutes the endoneurial tube.1 Along a myelinated nerve fiber, there are discrete areas not covered by the column of Schwann cells. These nodes or bared sections are called nodes of Ranvier. Portals, called ion channels, are located at these nodes. Conduction of an impulse along a myelinated nerve fiber can occur very rapidly as these channels open, allowing an infusion of charged ions that depolarize whole sections of the nerve fiber, from node to node, like a fuse that is continually being relit at each node.
Within the outermost covering, the epineurium, is a well-developed, longitudinally oriented vascular system that feeds the nerve fibers. Peripheral nerves are vulnerable to vascular changes as a result of altered circulation or blood flow within the epineurium of the nerve. Butler aptly describes nerves as “blood thirsty”9 with the nervous system consuming 20% of the available oxygen in circulating blood, even though this system consists of only 2% to 5% of the body mass. Lundborg and Rydevik demonstrated that when a nerve is stretched to about 8% (in a range of 5% to 10%) of its original length, venous stasis develops. The vulnerability of axons in the PNS to vascular changes is well-documented.8,11,12 The effects vary depending on the duration as well as the magnitude of the trauma. Prolonged stretch or compression with consequent compromised circulation will result in edema within the nerve’s connective tissue coverings once circulation is restored. This swelling will lead to nerve pathology.
Mechanism of Nerve Injuries: Incomplete versus Complete Injuries
Knowing the mechanism of the nerve injury is helpful for estimating prognosis, need for, and type of medical and/or therapeutic intervention. Etiology of the injury can include compression or entrapment from internal sources, such as a tumor or scar tissue or compression from an external source (such as, crutches or a cast). Nerve injury may occur via traction or stretching to the nerve, by avulsion or laceration, by chemical or electrical burns, or by radiation. Nerve injuries are classified by whether the nerve fibers are severed or whether the nerve fibers and/or their endoneurial tube remain in continuity despite various degrees of damage to the nerve’s connective tissue coverings and to the nerve fiber itself. Nerve injuries where the nerve is completely severed or where the nerve shows serious internal disorganization are considered to have the poorest prognosis for functional recovery.10,14 Typically, these cases require surgical intervention to allow for some amount of functional recovery.
Incomplete Injuries (Injuries Where the Nerve is Still in Continuity)
Neuropathy is the term used to describe pathologic conditions of the peripheral nerve. Incomplete injuries to a nerve are defined as those where the external connective tissue coverings remain to some degree intact. An incomplete injury has important therapeutic implications. Mononeuropathy involves damage to a single nerve.15 An example would be compression of the median nerve at the carpal tunnel, or carpal tunnel syndrome (CTS). Multiple mononeuropathy is a multi-focal asymmetrical involvement of multiple nerves.15 Compression of the right median and ulnar nerves as a complication of occupational stresses that require repetitious elbow and wrist flexion is an example of multiple mononeuropathy.
Altered axoplasmic flow in a peripheral nerve that results from diminished blood flow can cause the entire nerve to become sick and thus to become vulnerable to more than one site of irritation along its axon. Increased susceptibility for a secondary impingement may develop in areas where the nerve must traverse through spaces of increased friction (for example, fibroosseous tunnels). When the nerve has serial impingements or dual sites of pathologic manifestations that developed without a history of acute trauma, it is called double or multiple crush syndrome.15
Metabolic changes can result in neuropathies as well. Typically, one sees polyneuropathy, or bilateral extremity damage to two or more peripheral nerves. Peripheral polyneuropathy may involve the feet as well as the hands, and it occurs most frequently in people who smoke, who have nutritional deficiencies secondary to alcoholism, and who have autoimmune disease and/or difficulty controlling their blood sugar levels.10,15 Often such nerve damage is in a stocking and/or glove distribution. Neuropathies secondary to pregnancy will often resolve gradually as the woman’s body returns to its prenatal metabolic state.16 Other polyneuropathies may be stable with medications or may be progressive as the disease advances.
If this metabolic conduction block is of long enough duration and/or intensity, it will result in functional deficit, pain, and/or anesthesia in the region that that nerve supplies.7,11,12,13,17
Venous blood flow from the peripheral nerves is shown to be reduced at 20 to 30 mm Hg. A classically sited study by Gelberman, et al.13 demonstrated that with 90° of wrist flexion or extension, carpal tunnel pressure in those persons without CTS rose to 30 mm Hg on average. Thus, compressive forces on a peripheral nerve can be exacerbated by certain provocative postures, such as holding the wrist in end range flexion or extension. If these forces are augmented by concomitant pathology, such as swelling in the carpal tunnel area following a wrist fracture, a metabolic block or compression neuropathy may develop. If compression is of long enough duration (that is, if the compression is long standing but of a comparatively low level), one will see diminished microvascular flow in the nerve’s fascicles, resulting in a chronic reduction of the nerve’s axoplasmic flow and slowing of the nerve’s conduction of impulses (slowed conduction velocity). Function will fail sequentially in the following order: motor, proprioception, touch, temperature, pain, and then sympathetic function. Recovery occurs sequentially in the reverse order following timely conservative or surgical intervention.
Timelines and Healing (Incomplete Nerve Injuries)
Nerve compression initially results in a loss of proprioception and discriminative touch, followed by a loss of pain and temperature sensation. This loss of proprioception occurs first because compression of a nerve affects the large myelinated fibers (that carry information about proprioception and discriminative touch) prior to affecting the smaller, unmyelinated fibers. If the compression persists or is severe enough, the smaller fibers that carry information about pain and temperature also are lost.8
When the compression resolves, abnormal sensations called paresthesias occur as the blood supply to the nerve resumes. The client may complain of burning, tingling, or pricking sensations. Sensation gradually returns in the reverse order in which it was lost: dull, diffuse aching pain; perception of heat; sharp, stinging sensations; cold perception; conscious proprioception; and finally, discriminative touch.18
Complete Nerve Injuries (Injuries Where the Axon Is Transected and/or There Is Significant Loss or Disorganization of the External Connective Tissue Layers)
Grave consequences in terms of motor and/or sensory loss will occur following an injury where the peripheral nerve is severed, or where a segment of the nerve is severely traumatized with serious internal disorganization. Following such an injury, the nerve is divided into two parts: a proximal part attached to the nerve cell body, and a distal part, which undergoes degeneration. Functional recovery depends upon replacement of the lost distal portion by the outgrowth of new axonal processes growing to reach their corresponding original peripheral targets.17
When a nerve is severed, an immediate consequence is loss of its vital axoplasmic fluid.21 The axon itself contains 90% of the axoplasmic fluid present in that nerve cell. The axoplasmic transport system (with its retrograde and anterograde flows) allows the neuron to maintain its structure and health at its synapses. This system also allows the soma (or nerve cell body) to get feedback about the axon’s overall health including the health of the nerve’s distally located synaptic clefts. When this communication and transport system fails, the muscle and sensory receptors associated with that nerve change. There is a loss of or decrease in these receptors’ ability to receive or generate impulses. One will also see trophic changes in the target tissues: that is changes in the tissues served by that nerve that include abnormal hair growth, nail bed changes, cold intolerance, and soft tissue atrophy.
Another consequence of axonal transection is that the soma, or cell body, swells, and the cell’s nucleus is displaced peripherally. This reflects a change in the metabolic priority from production of neurotransmitters to production of structural materials needed for axon repair and growth. The more proximal the site of injury, the more intense the cell body’s reaction, which peaks about 2 to 3 weeks post injury.17 The proximal axon undergoes traumatic degeneration within the zone of injury (that is, to the next node or nodes of Ranvier). The endoneurial tubes of the proximal axon lie empty. Distal to site of injury, Wallerian degeneration, or the breakdown of the axon distal to the site of injury, occurs. This process begins about 48 to 96 hours after transection and concludes about 3 weeks post injury. During this time, there is progressive deterioration of the myelin, and the distal axon becomes increasingly disorganized. This one of the reasons that best prognosis for surgical repair is when the surgery can be performed within that 48 to 96 hours.14,17
