CHAPTER 28 Perfusion and oxygenation
1. Identify and describe two factors that impair perfusion and related wound repair processes.
2. Describe the microcirculation and tissue oxygen characteristics of the wound environment.
3. Discuss the effects of an activated sympathetic nervous system on the wound repair processes.
4. Differentiate among the roles of wound tissue oxygen and arterial oxygen in wound repair.
5. Describe four interventions to increase tissue oxygen or improve wound healing.
A great deal has been learned over several decades about tissue repair and the importance of perfusion and oxygenation to healing, although much of that information has not been applied widely in daily clinical practice. Recognition is growing that inadequate wound healing and complications, such as surgical site infection, of acute wounds likely have their origins early in the initial inflammatory response and early in the healing process, when tissue breaking strength is low and physiologic responses may not be optimal (Dubay and Franz, 2003).
Impact of oxygen and perfusion in wound healing
The critical roles of oxygen in healing are summarized in Table 28-1. Oxygen fuels the cellular functions essential to the repair process; therefore the ability to perfuse the tissues and the availability of oxygen (partial pressure of oxygen [PO2]) to the local wound area are critical to wound healing. The processes of tissue repair that require oxygen include oxidative bacterial killing and resistance to infection, collagen synthesis and fibroplasia, angiogenesis, and epithelialization (Hartmann et al, 1992; Hopf and Rollins, 2007; Hunt, Ellison, and Sen, 2004; Hunt and Aslam, 2004; Sen, 2003).
TABLE 28-1 Important Roles of Oxygen in Wound Healing
Event in Wound Healing | Specific Role of Oxygen |
---|---|
Inflammation: Bacteria control | Oxygen is substrate for enzymatic step for leukocyte production of reactive oxygen species (superoxide and other oxidants) during phagocytosis |
Proliferation: Angiogenesis | Local tissue hypoxia and oxidants induce release of growth factors that stimulate angiogenesis |
Proliferation: Collagen synthesis and cellular export | Oxygen with adequate vitamin C is required for enzymatic hydroxylation of procollagen to form triple helical, mature collagen structure for export from fibroblasts Production and release of collagen are necessary for tissue strength |
Proliferation: Epithelialization | Oxygen and growth factors are needed for generation of new epithelium |
Tissue oxygen levels are dependent on both perfusion status and oxygen content of the blood. However, because wounds remove only 1 ml of oxygen per each 100 ml of blood perfusing the tissues, compromised perfusion is more likely to jeopardize wound healing than is compromised oxygenation secondary to pulmonary conditions (Waldorf and Fewkes, 1995). Tissues that are adequately perfused are often able to heal even if the blood is poorly oxygenated or the patient is anemic. In fact, anemia usually does not significantly affect repair unless the hematocrit drops below 20% (Stotts and Wipke-Tevis, 1996). Arterial oxygen levels are not necessarily reflective of tissue oxygen delivery. Although PaO2 of 90 mm Hg in a healthy volunteer breathing room air maintains a wound PO2 of 50 mm Hg or greater, the postoperative patient experiencing autonomic nervous system activation or periodic desaturation will exhibit predictably low oxygen levels within the wound. This observation led to an approach of adrenergic activation as an etiology for wound hypoxia (West, 1990).
Wound tissue hypoxia
Wound tissue hypoxia occurs to some extent at the time of injury in everyone, regardless of their age or state of health (Silver, 1980). Incisions closed during elective surgery under the best of aseptic conditions appear to have the fewest reparative obstacles to healing. However, any tissue injury disrupts vascular and therefore oxygen supply. All wounds are relatively hypoxic at the center, in the range from 0 to 5 mm Hg (Niinikoski et al, 1972; Silver, 1969). After oxygen leaves the red blood cells in the capillaries, it diffuses into the wound space. The driving force of diffusion is partial pressure. In wounds, damage to the microvasculature, vasoconstriction, and intravascular fluid overload markedly increase intercapillary distances (Hunt and Hopf, 1997).
Local oxygen tension influences wound healing and bacterial control in several ways. Initial injured tissue is hypoxic, a state that acts as a stimulus for repair. The one potentially beneficial effect of moderate hypoxia is enhanced stimulus to neoangiogenesis (Wilson and Clark, 2003; Zamboni et al, 2003). However, prolonged and decreased local wound oxygen (tissue hypoxia) is a major contributor to wound complications (Goodson et al, 1979; Jönsson et al, 1988; Knighton et al, 1984). A main role of oxygen during repair is that of controlling bacteria within the wound site. During repair, production of reactive oxygen species by leukocytes for phagocytosis is needed prior to rebuilding tissue (Hopf and Rollins, 2007; Hunt, Ellison, and Sen, 2004). Oxidant production by leukocytes requires molecular oxygen and the presence of tissue oxygen levels of 45 to 80 mm Hg up to 300 mm Hg (Allen et al, 1997). In addition to their role in controlling bacteria, reactive oxygen species serve other roles in healing, which include acting as chemical signals and mitogens for fibroblasts and other cells, inducing cellular adhesion in neutrophils and macrophages, and cellular expression of growth factors that are critical for repair (Sen, 2003). Vascular endothelial growth factor that stimulates angiogenesis is one of the growth factors released in response to hypoxia and reactive oxygen species production (Hunt and Aslam, 2004). Through these processes, reactive oxygen species act to help coordinate the tissue repair process.
Factors that alter perfusion and oxygenation
Pain and stress
Cannon (1970) revealed the mechanisms that activate the autonomic nervous system to respond to stress. He directly observed the constriction of blood vessels in peripheral tissues during stress in animals. The mediators of the sympathetic and adrenal response to stress (epinephrine and norepinephrine) induced profound vasoconstriction in subcutaneous and skin blood vessels supplying peripheral tissues (Rowell, 1986). Sympathetic activation in the postoperative period is a function of the severing of afferent nerves, hypovolemia, fear, pain, and cold rather than anesthesia (Halter et al, 1977). Catecholamine levels may remain elevated for days after surgery (Derbyshire and Smith, 1984; Halter et al, 1977), and concentrations vary with the length and severity of surgery (Chernow et al, 1987). Norepinephrine is increased threefold in the early postoperative hours (Derbyshire and Smith, 1984), peaking with the patient’s first expression of pain (Niinikoski et al, 1972). Increased levels of circulating catecholamines, including epinephrine, triggered by pain and stress lead to peripheral vasoconstriction, decreased perfusion of blood to the skin and extremities, and, consequently, reduced oxygen availability in the tissues (Franz et al, 2008; Jensen et al, 1985). Evidence of how stress and pain limit perfusion has been demonstrated by infusion of exogenous epinephrine in healthy subjects. In a study designed to mimic the body’s response to stress, increasing levels of epinephrine decreased the level of subcutaneous tissue oxygen 45%, whereas heart rate and arterial PO2 did not markedly change (Jensen et al, 1985).
Hypothermia
Adverse consequences of perioperative body heat loss are significant and include prolonged hypothermia and postanesthesia recovery, longer postoperative warming time, shivering and thermal discomfort, reduced antibody and cell-mediated responses, reduced tissue oxygen peripherally, and increased incidence of wound infections (Reynolds et al, 2008). The effects of temperature on the amount of vasomotor tone primarily determine cutaneous capillary blood flow. Cooling increases norepinephrine affinity to α-adrenergic receptors on vascular smooth muscle. This augments the response of cutaneous vessels to autonomic activation, which increases constrictive vessel tensions up to fivefold (Vanhoutte et al, 1981).
Hypothermia-induced vasoconstriction has decreased subcutaneous oxygen tension in anesthetized volunteers (FiO2 0.6 mm Hg) to a mean of 50 mm Hg (Sheffield et al, 1992). Furthermore, a lower blood temperature shifts the oxyhemoglobin curve to the left, thereby increasing the amount of oxygen carried in the blood but decreasing the amount of oxygen released (Severinghaus, 1958). This effect may exacerbate tissue hypoxia induced by peripheral vasoconstriction.
Virtually all anesthetic agents are vasodilators and may cause a rapid initial decrease in core temperature (1°C–2°C) during the first hour after induction of anesthesia (Sessler, 1993). Internal redistribution of body heat from core to periphery is exacerbated by conductive and evaporative losses as a result of visceral exposure in a cold environment (Roe, 1971). Rapid initial body heat loss can be limited by heating the operating room and aggressive preoperative warming (Morris, 1971). Preinduction warming may nearly prevent rapid initial body heat loss (Hynson and Sessler, 1992).
Perioperative shivering in the elderly, although rare, is dangerous because oxygen demand may increase by 400% to 500% (Bay et al, 1968). This drastic increase in oxygen demand increases cardiac workload. Increased oxygen consumption has been shown to coincide with core decrements of 0.3°C to 1.2°C (Roe et al, 1966). Prolonged postoperative hypothermia is associated with increased mortality (Slotman et al, 1985) and myocardial ischemia (Frank et al, 1993).
Tobacco
Smoking is associated with poor wound outcomes in part through the catecholamine-mediated vasoconstrictive effects of nicotine, increases in carbon monoxide that reduce blood oxygen content, and effects on immune and proliferative cell function that reduce repair (Gottrup, 2004; Warner, 2005). Vasoconstrictive effects resulting in significant decreases in tissue oxygen tension occur after a single cigarette is smoked, and tissue oxygen requires an hour to return to baseline levels (Jensen et al, 1991). Reductions in tissue oxygen translate clinically into lower amounts of collagen production and reduced wound strength (Jorgensen et al, 1998). Based on multiple studies and reviews demonstrating an association between smoking and wound-related problems, including infection and poor wound healing, all patients with wounds should be advised to abstain from smoking.
Smoking is the single most predictive factor of wound complications in patients undergoing elective hip or knee replacement (Moller et al, 2003). Forty-seven percent of patients undergoing abdominoplasty who smoked had wound complications compared to 14.7% of those who did not smoke (Manassa et al, 2003). At least one study has shown that 2 weeks of abstinence is inadequate to limit wound-related problems, whereas a minimum of 3 to 4 weeks of abstinence has been associated with reduced incisional wound infection and improved wound healing (Kuri et al, 2005; Sorensen et al, 2003). Due to the reductions in tissue oxygen supply when a person smokes, the substantial time needed for levels to recover, and the multiple clinical studies documenting the significance of smoking as a factor that impairs healing, the Wound Healing Society recommends that patients stop smoking at least 3 to 4 weeks before elective surgery and that they not resume smoking in the postoperative period (Franz et al, 2008).
Obesity
Wound healing problems are more likely to occur in patients who are overweight, which is a growing national problem. More than 72 million Americans are considered obese (body mass index [BMI] ≥30), representing an obesity rate of 34% in adults older than age 20 years (Ogden et al, 2007). Individuals who are severely obese often suffer from a number of related health problems that potentially impact healing: type 2 diabetes, hypertension, coronary artery disease, sleep apnea, venous stasis disease, lower extremity ulcers, osteoarthritis, urinary incontinence, gastroesophageal reflux disease, fatty liver, cholelithiasis, and depression (Mun et al, 2001).
Obesity as a single factor is an independent predictor of surgical site infection (Harrington et al, 2004). Regardless of the surgical procedure, the incidence of postsurgical wound complications, such as infection and wound dehiscence, are higher in patients with obesity compared to those of normal weight (Franz et al, 2008). Postsurgical wound complication rates of patients with obesity have been reported to be as high as 15% to 22% (Fried et al, 1997; Israelsson and Jönsson, 1997; Myles et al, 2002; Vastine et al 1999; Winiarsky et al, 1998).
Several studies document low oxygen levels associated with obesity. Abdominal tissue oxygen tension is reported to be negatively associated with the percentage of fat as measured by body composition dual-energy X-ray absorptiometry, with obese subjects found to have lower adipose tissue capillary density (Pasarica et al, 2009). In surgical populations, patients with BMI greater than 30 kg/m2 who had undergone abdominal surgery had significantly lower tissue oxygen readings compared to those with BMI less than 30 kg/m2 (Fleishmann et al, 2005; Kabon et al, 2004). Tissue oxygen levels measured in patients with obesity fell below normal, even with administration of supplemental oxygen, and were within a range associated with increased surgical site infection risk.
Because of the increased vulnerability to wound complications, extra vigilance and measures to ensure peripheral perfusion must be taken with patients who are obese, including increased FiO2 during the perioperative period unless contraindicated by patient condition, maintenance of normothermia, and adequate fluid resuscitation. Higher oxygen concentrations may be needed in order to maximize tissue levels. In addition, larger doses of prophylactic antibiotics may be needed because of evidence indicating that decreasing serum and tissue concentrations fall below therapeutic levels as BMI increases (Anaya and Dellinger, 2006). Obesity is addressed in greater detail in Chapter 35.
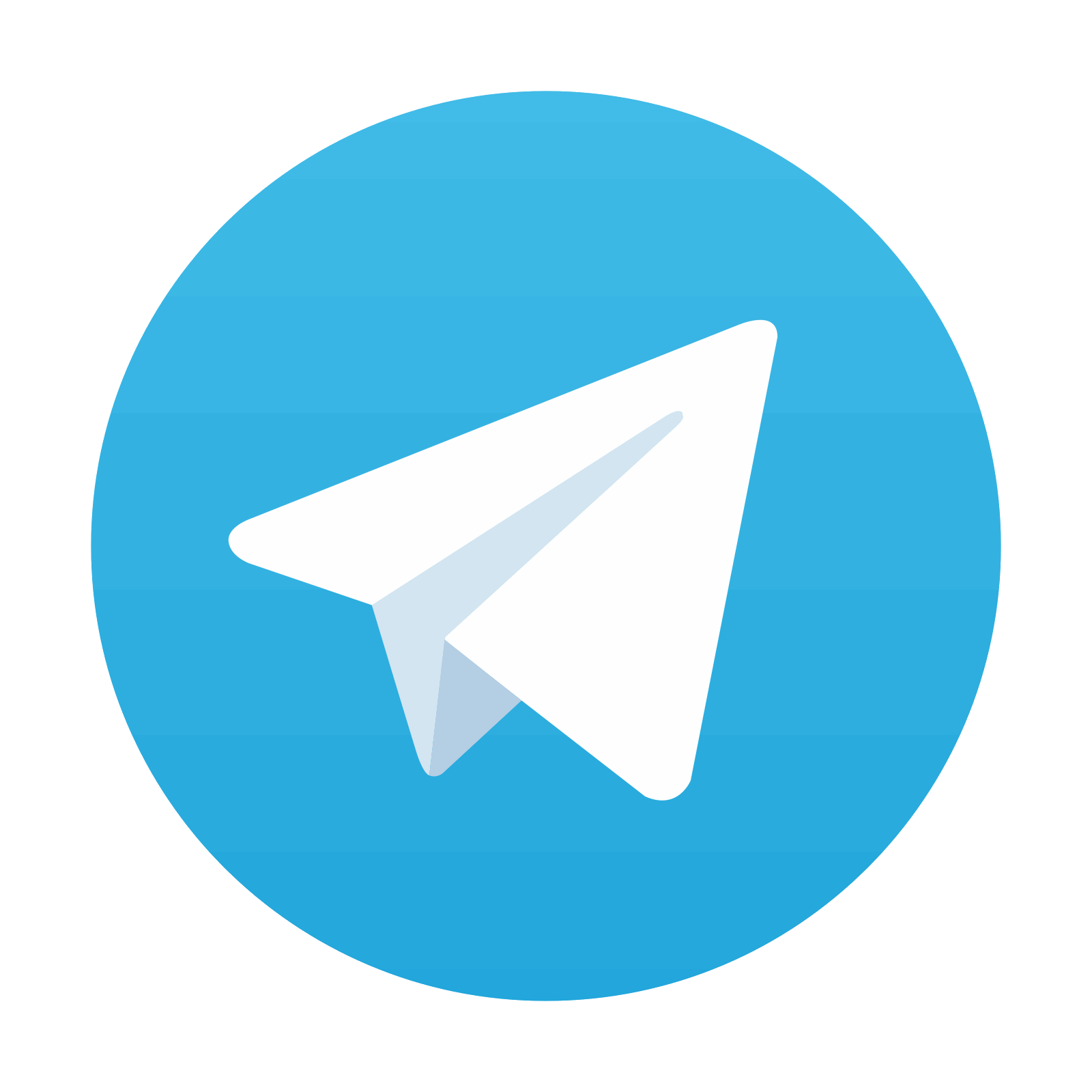
Stay updated, free articles. Join our Telegram channel

Full access? Get Clinical Tree
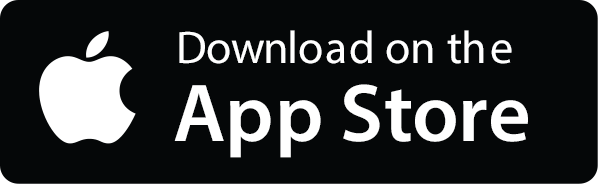
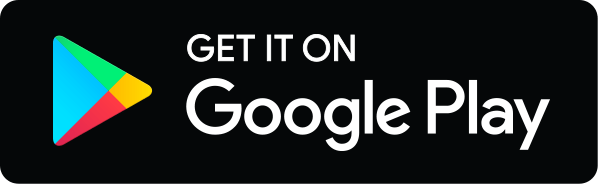