, Francois Lintz2, Cesar de Cesar Netto3, Alexej Barg4, Arne Burssens5 and Scott Ellis6
Keywords
Subtalar jointInstabilityWeight bearing CTImagingIntroduction
Chronic hindfoot instability is a frequent problem that is evident in up to 33% of patients with a history of ankle sprains [1]. Hindfoot instability often includes the ankle joint but can also affect the subtalar joint [2–4]. While ankle joint instability can be diagnosed clinically, an accurate assessment of the subtalar joint remains elusive [2, 3, 5]. To provide an adequate treatment for patients with post-traumatic hindfoot instability, a meaningful assessment of the subtalar joint is desirable [2].
Two ligaments are the primary stabilizers of the subtalar joint: the interosseous talocalcaneal ligament (ITCL) and the calcaneofibular ligament (CFL) [6–9]. Other ligaments providing subtalar joint stability include the cervical ligament, lateral talocalcaneal ligament, and deltoid ligament [6–8, 10, 11]. Recent cadaver studies showed that the CFL is potentially the most important stabilizer of the subtalar joint when subjected to inversion and external rotation stress [7, 8]. As the CFL crosses both the ankle and subtalar joint, the stability of both joints is affected after injury. In contrast, the ITCL only provides stability to the subtalar joint [10].
Variation in injury patterns and its complex anatomy make diagnosing subtalar joint instability particularly challenging [2, 12]. Long-lasting instability of the lateral ligament complex results in degenerative changes and chronic hindfoot pain [2, 12–14]. The impact of subtalar joint instability on this development remains unclear [15]. This emphasizes the relevance of a radiographic diagnosis, currently performed by several two-dimensional (2D) measurements including tibiotalar tilt (TT), anterior talar translation (ATT), and subtalar tilt (STT) [2, 4, 16–21]. However, these measurements are limited in their ability to identify subtalar joint instability when using stress radiographs [2, 4].

Frequently used measurement methods in conventional radiographs and clinical examination of subtalar joint instability [2]
While conventional radiographs are limited in assessing the subtalar joint, weight bearing computed tomography (CT) scans have demonstrated emerging diagnostic applications as they offer an accurate representation of hindfoot joint alignment under weight bearing conditions [22–25]. However, the clinical use of this imaging modality to diagnose subtalar joint instability has yet to be investigated. We hypothesized that isolated subtalar joint instability can accurately be diagnosed when using weight bearing CT scans in a cadaver model.
Methods
Data Source and Specimens
Seven pairs of fresh frozen male cadavers (tibial plateau to toe-tip) were included (mean age 63 ± 5 [range 54–69] years; mean weight 77.2 ± 6.9 [range 68.7–90.7] kg; mean BMI 24.1 ± 1.3 [range 22.2–25.7] kg/m2). Inclusion criteria were 20 to 70 years of age and a body mass index (BMI) of less than 35 kg/m2. Only male cadavers were included to ensure a homogeneous cohort. Exclusion criteria were a history of foot and ankle injuries or previous foot and ankle surgery.
Experimental Setting

Experimental setting. (a) The radiolucent frame (polyoxymethylene, an engineering thermoplastic providing high stiffness, low friction, and dimensional stability – Delrin™) used to hold the Ilizarov apparatus consisted of a base plate and four pillars located lateral to the foot. This allowed the Ilizarov apparatus to rotate in a concentric cylinder when torque was applied. While torque was manually applied, the Ilizarov apparatus was rigidly fixed to the frame through four slots (∗), each accepting a clamp that fastened to the ring in order to maintain the torque over time. (b) The tibia was fixed into an Ilizarov apparatus that fits into the frame. A post (∗) was centrally positioned at the proximal end of the Ilizarov to accept a torque wrench. The distal end of the post was placed in the line of the axis of the tibial shaft (at the level of the tibial plateau). Two Kirschner wires (K-wires) were placed on the level of the proximal ring through the tibia (from anteromedial to posterolateral and from medial to lateral). The same fixation holes at the Ilizarov apparatus were used when possible for each experiment. Then, the K-wires on the distal ring were placed similar. Loads were placed on a proximal plate fixed to the Ilizarov apparatus (arrow). The hindfoot was fixed using six 1.5 mm K-wires drilled through the calcaneus using a standardized hole pattern in the side of the retaining box (∗∗). If the tibia was fixed properly to the Ilizarov apparatus, the position of the heel was typically flush to the bottom of the frame. (c) The frame fit into the computed tomography (CT) scanner [1]

Transection of the ligaments providing stability to the subtalar joint. (a) Transected calcaneofibular ligament (CFL, ∗); posterior facet of the subtalar joint (∗∗). (b) Additional transection of the interosseous calcaneofibular ligament (ITCL); talus (∗); sinus tarsi showing the transected ITCL (∗∗); posterior facet of the subtalar joint (∗∗∗). A lamina spreader was used to ensure good visualization of the sinus tarsi (including the medial aspect). (c) Transection of the deltoid ligament; tibialis posterior tendon (∗); transected deltoid ligament (∗∗); flexor digitorum longus tendon (∗∗∗) [1]
Non-weight bearing and weight bearing (85 kg; determined from the average of specimen donor anthropometrics) CT scans with and without application of 10 Newton meter (Nm) internal torque applied at the Ilizarov apparatus (corresponding to external torque of the foot and ankle) were collected [27]. Ten Nm torque was chosen for consistency with cadaver studies testing the stability of the distal tibial syndesmosis [27, 28]. Preconditioning of the specimen was performed by consistent loading of the frame with 42.5 kg and 85 kg for 2 minutes each before experimentation.
Imaging and Measurements
Digitally reconstructed radiographs (DRRs) were automatically created using the CT scan dataset (CurveBeam LLC, Warrington, USA). The anteroposterior (AP) view of the ankle joint was generated perpendicular to a line connecting the center of the calcaneus (midway between the medial and lateral process of the tuber calcanei) and the second metatarsal base on a dorsoplantar (DP) view [29]. By virtually externally rotating the foot 90 degrees, a lateral view was generated. Additionally, a 30/40-degree Broden view was reconstructed (30 degrees internal rotation and 40 degrees upward tilt of the foot) [19]. The TT and ATT were measured on the AP and the lateral view, while the STT was assessed on the 30/40-degree Broden view [2, 4, 19–21]. TT and ATT measurements were performed to additionally evaluate the effect of ligament transection (CFL and deltoid ligament) on ankle joint congruency.

Measurements performed on single computed tomography (CT) images (discrete calcaneofibular ligament [CFL] dissection). (a) Lateral view showing the measurement of the anterior talar translation (ATT). (b) Anteroposterior (AP) view showing the measurement of the tibiotalar tilt (TT). (c) 30/40-degree Broden view (middle plain) showing the measurement of the subtalar tilt (STT) [1]
Statistical Analysis
Intraclass correlation (ICC) was used to quantify the agreement of measurements between and within observers. Estimates and 95% confidence intervals (CI) were calculated for each type of measurement within each view. Interobserver agreement was modeled with a two-way random effect model of absolute agreement with a single measurement per observation. Intraobserver agreement was modeled with a two-way mixed effect model of consistency with a single measurement per observation. Agreement was rated as very good with an ICC > 0.80; good with an ICC = 0.61–0.80; moderate with an ICC = 0.41–0.60; fair with an ICC = 0.21–0.40; and poor with an ICC < 0.20 [31]. Measurements for interobserver agreement calculation were done by a fellowship trained orthopedic surgeon and a research analyst. For calculation of the intraobserver agreement, measurements were performed two times with an interval of 3 weeks by a fellowship trained orthopedic surgeon.
Linear mixed effect models were fit for responses. Within DRR and CT measurements, separate models were fit for each measurement (TT, ATT, and STT) and, for DRR measurements, within each view. Cadaver, a random effect, foot (left or right), and a fixed effect were included in all models in addition to the variables presented. Models were fit for subsets of the data, and estimates and 95% CI were given for differences in measurements in different levels of a specific variable. For each model, only the differences in response that were associated with either different load application, different torques, or different conditions were calculated; the data was subset by the other two variables, and they remained constant within each model. The first set of models compared the differences in response for full versus non-weight bearing with no torque applied (condition constant within each model). The second set compared the differences in response to 10 Nm versus 0 Nm of torque applied with full weight bearing load (condition constant within each model). The last set compared the differences between Conditions 1 through 3 and the native ankles, with full weight bearing load (torque constant within each model). Coefficients and 95% confidence intervals were reported, and statistical significance (marked by an asterisk in all tables and graphs) was determined based on a P value less than 0.05. All calculations were done in R 3.4.1, specifically using packages psych and lmerTest.
Results
Digitally Reconstructed Radiographs
Reliability of digitally reconstructed radiographs measurements assessed by intraclass correlation (ICC) [1]
Measurement | Interobserver: ICC(2,1) estimate (95% CI) | Intraobserver: ICC(3,1) estimate (95% CI) |
---|---|---|
TT | 0.22 | 0.29 |
(−0.07, 0.50) | (−0.06, 0.58) | |
ATT | 0.75∗ | 0.88∗ |
(0.55, 0.87) | (0.78, 0.94) | |
STT | 0.88∗ | 0.97∗ |
(0.70, 0.95) | (0.93, 0.98) |
Native | Condition 1A | Condition 1B | Condition 2 | Condition 3 | ||
---|---|---|---|---|---|---|
Estimate (95% CI) | ||||||
DRR | TT (degrees) | 0.03 | −0.18 | −0.18 | −0.25∗ | −0.20∗ |
(−0.13, 0.19) | (−0.54, 0.17) | (−0.36, 0.00) | (−0.42, −0.08) | (−0.37, −0.03) | ||
ATT (mm) | −0.23∗ | −0.16 | −0.03 | −0.19∗ | −0.11 | |
(−0.38, −0.08) | (−0.32, 0.00) | (−0.26, 0.20) | (−0.37, 0.00) | (−0.30, 0.08) | ||
STT (degrees) | 0.01 | 0.10 | −0.08 | −0.15 | 0.08 | |
(−0.44, 0.47) | (−0.31, 0.51) | (−0.32, 0.16) | (−0.50, 0.20) | (−0.20, 0.36) | ||
CT | TT (degrees) | −0.03 | −0.22∗ | −0.04 | −0.16 | −0.08 |
(−0.17, 0.11) | (−0.40, −0.04) | (−0.23, 0.15) | (−0.41, 0.10) | (−0.23, 0.08) | ||
ATT (mm) | −0.17 | −0.07 | 0.03 | −0.18 | −0.09 | |
(−0.45, 0.11) | (−0.94, 0.80) | (−0.43, 0.49) | (−0.58, 0.22) | (−0.44, 0.26) | ||
aSTT (degrees) | −0.02 | −0.12 | −0.04 | −0.08 | −0.06 | |
(−0.24, 0.21) | (−0.26, 0.03) | (−0.21, 0.13) | (−0.30, 0.14) | (−0.20, 0.08) | ||
mSTT (degrees) | 0.11 | 0.12 | −0.04 | 0.05 | −0.01 | |
(−0.07, 0.28) | (−0.05, 0.28) | (−0.26, 0.18) | (−0.10, 0.21) | (−0.20, 0.19) | ||
pSTT (degrees) | 0.17 | −0.04 | 0.14 | 0.07 | 0.05 | |
(−0.29, 0.63) | (−0.72, 0.63) | (−0.22, 0.49) | (−0.31, 0.46) | (−0.32, 0.42) |
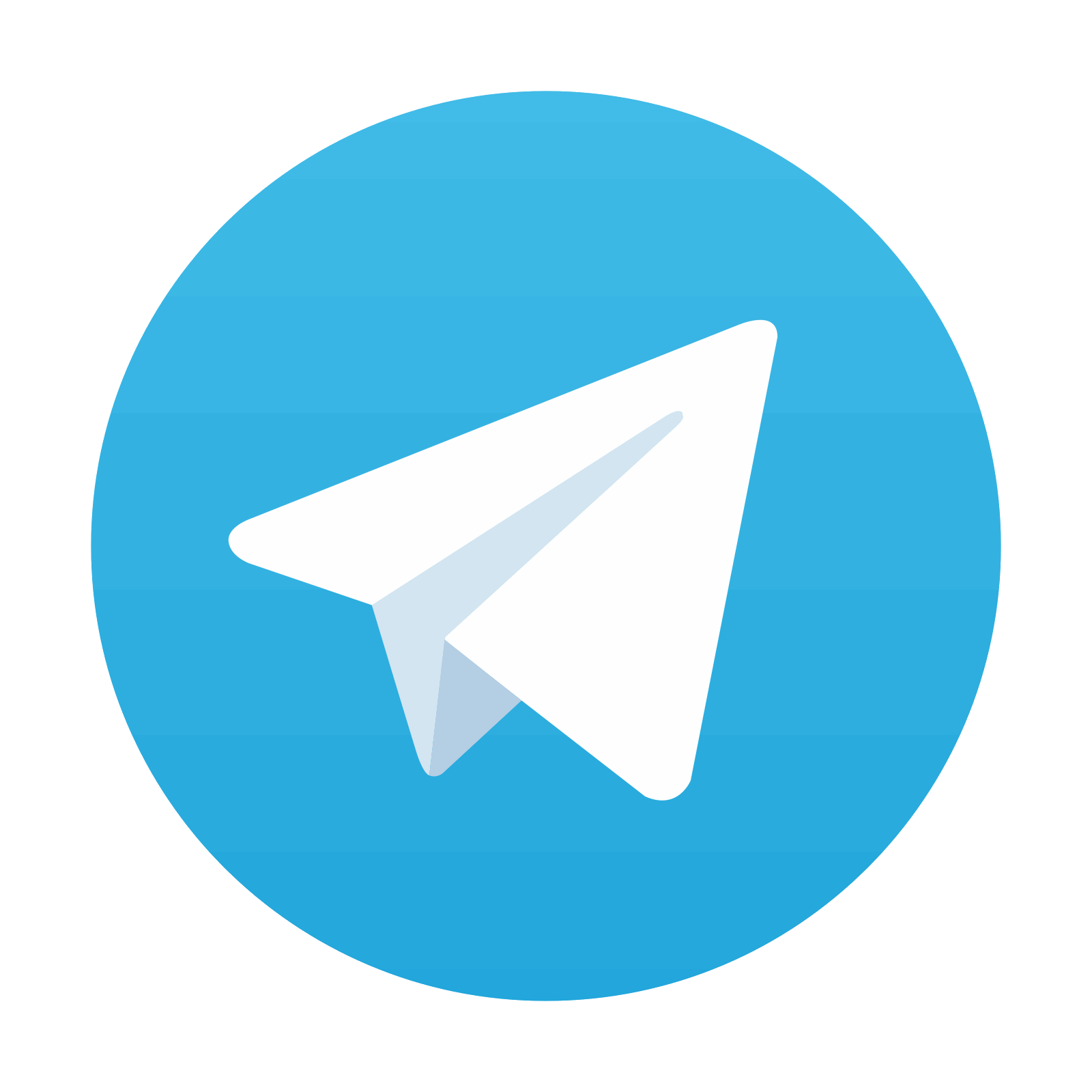
Stay updated, free articles. Join our Telegram channel

Full access? Get Clinical Tree
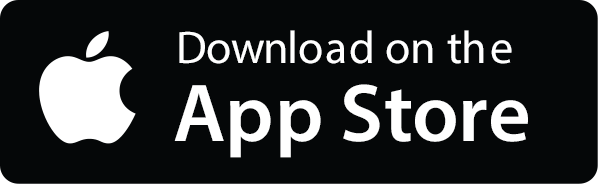
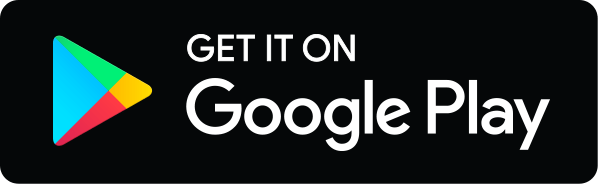