Etiology
No.
Problems (n = 70)
Complications (n = 188)
Reinterventions (n = 105)
Reoperations (n = 260
Revisions (n = 79)
Total
782
44%
24%
3.3%
10.1%
PAG
566
6.0%
13.4%
3.0%
6.3%
CTA
318
6.9%
19.5%
11.9%
–
Frx Seq
41
N/S
5%
4.9%
–
RA
23
21.7%
45%
26.1%
–
Acute Frx
18
11.1%
36%
6.3%
12.5%
Tumor
6
N/S
N/S
N/S
–
N/S
160
N/S
N/S
N/S
–
Revision
216
12.5%
33.3%
4.2%
15.7%
As RTSA has become a more common operation, the training of surgeons who perform this surgery has been evaluated by several studies. One study found that the complication rate decreases after ten cases [8]. Other studies have suggested that the learning curve may even extend beyond 100 cases [9]. A study of surgeons in the United States who were submitting cases for their board certification found that the complication rate was similar between those who were fellowship trained and those who were not [10]. This may be explained by the complexity of the cases of the fellowship trained group, but it may also indicate that the complications may be inherent to the use of RTSA. There is little doubt that the variety of complications and the number of complications of RTSA make it a challenging operation.
20.3 Deep Prosthetic Shoulder Infection
Deep prosthetic shoulder infection is one of the most frustrating complications for patients and surgeons alike. The need for multiple surgical procedures, intravenous antibiotics, and decreased functionality after several operations can be time-consuming and painful for patients. Reconstruction of both the humerus and glenoid sides can be challenging due to the bone and soft tissue loss, which occurs as a result of infected shoulder arthroplasty. Deep prosthetic shoulder infection after RTSA occurs roughly 2–3% of the time in primary cases and between 5 and 6% in revision cases [11]. This rate is higher compared to the infection rate of anatomic total shoulder arthroplasty which is roughly 1–2% and closer to the incidence of deep infection in knee or hip replacements [12, 13]. The increased incidence of deep prosthetic shoulder infection compared to anatomic shoulder replacements is thought to be secondary to prosthesis design, as with RTSA there are larger areas of dead space and there is more implant mass available for the formation of bacterial biofilm [2]. Additionally, the rotator cuff is typically absent in RTSA surgeries resulting in less viable tissue to protect the implant against bacterial colonization [14]. The most common microorganisms in deep prosthetic infections of RTSA are Staphylococcus aureus, coagulase-negative staphylococci, and Cutibacterium acnes [15]. C. acnes is of particular interest as it rarely presents in hip or knee replacement infections, and its slow growth rate explains the sometimes late presentation after RTSA. Risk factors associated with deep prosthetic shoulder infections after RTSA include diabetes, chronic steroid therapy, systemic lupus erythematosus, rheumatoid arthritis, previous surgical procedures, and remote sources of infection [16].
The diagnosis of deep prosthetic shoulder infection can be somewhat challenging especially in the setting of C. acnes as it is a low-grade infection and clinical symptoms are usually subtle. Swelling of the shoulder is rare, and limitations of range of motion can be masked by compensatory motion in the scapula-thoracic joint. Obviously the most diagnostic symptom is drainage from either the surgical wound or from a distant site, such as the anterior and posterior shoulder, biceps in the mid-arm, and the axilla. Pain after surgery, especially with a known history of a previous wound complication, is one of the most common symptoms of deep prosthetic shoulder infection [17]. Surgeons should ask when the pain began and if there was ever a period of pain relief after surgery. White cell count can be normal in deep prosthetic shoulder infection; however the erythrocyte sedimentation rate (ESR) and C-reactive protein (CRP) are typically elevated [18]. It is important to keep in mind that uncomplicated RTSA surgery itself will elevate ESR and CRP levels; however these should fall within normal limits at about 3 weeks after surgery [19]. Inflammatory markers in C. acnes infections however can be negative given its ability to produce only a low-grade inflammatory state [19].

Radiographic evidence of infection in RTSA can include radiolucent lines along the humeral and glenoid components along with signs of loosening. This radiograph shows radiolucent lines between the cement mantle and the bone extending throughout the entire humeral prosthesis. The glenoid prosthesis has radiolucent lines along the undersurface of the baseplate as well as around all of the screws indicating likely infection with possible loosening
Management of deep prosthetic shoulder infection primarily depends upon the time of presentation after the surgical procedure. Infections can be divided into acute infections (1–3 months), subacute infections (4–12 months), and chronic infections (>1 year). Superficial acute infections can be treated with local wound care and IV antibiotics, while deeper acute infections should be treated with irrigation and debridement, polyethylene inlay exchange, and antibiotics. Unfortunately irrigation and debridement with polyethylene exchange only successfully eradicates the infection in roughly 50% of acute infections and rarely works for subacute or longer infections [22]. One study by Zavala et al. emphasized the importance of recognizing deep prosthetic shoulder infections as early as possible [23]. In infections diagnosed in less than 2 weeks after surgery, irrigation and debridement, component retention, and 6 weeks of intravenous antibiotics cleared the infection in all cases.
Chronic infections are more difficult to treat and require more invasive techniques. Chronic infections typically require either a one- or two-stage procedure with direct prosthesis exchange in one-stage and prosthesis explant with placement of an antibiotic spacer followed by prosthesis reimplantation in two-stage revisions. A systematic review comparing these two treatment options suggested one-stage revisions might have a slight advantage over two-stage revisions [24]. Jacquot et al. had 100% healing with one-stage revision compared to 64% in two-stage revision although this difference was not significant [25]. Beekman also found that one-stage revisions for infected RTSA provided a 90% healing rate [26]. One-stage revisions reduce costs and duration of treatment with good functional outcomes.
Revision of infected arthroplasties can be fraught with many challenges. The first is to eradicate the infection. Recurrence of infection after revision for infected shoulder arthroplasty has been reported to be as high as 14.3% compared to an infection rate of less than 2% in primary RTSA [24]. Also the incidence of aseptic baseplate loosening after revision of RTSA is around 4%, whereas the incidence is around 1% for primary RTSA [27]. Other options for infected RTSA include removal of the components and implantation of an antibiotic spacer alone. While not a common treatment, some patients tolerate the spacer long term despite the limitations of pain and loss of motion with the device. Resection arthroplasty is another option for treatment of an infected RTSA; however the outcomes are typically poor [23]. As a result, this option should be reserved for patients with recurrent and incurable infections, multiple medical comorbidities, or inability to successfully carry out revision reconstructive procedures [23].
20.4 Instability

Radiographic evaluation and management of RTSA instability. (a) Immediate postoperative Grashey view of RTSA without evidence of instability. (b) 9 weeks post op Grashey view shows dislocated RTSA prosthesis. (c) Postoperative Grashey view after revision of humeral component including metal insert buildup and increase in polyethylene thickness. Intraoperative tensioning improved after humeral components revised. Modification to glenoid component was unnecessary
There are multiple factors that can contribute to instability after RTSA. The two major factors are the type of prosthetic design and the use of RTSA for revision of a previous shoulder arthroplasty. The Grammont prosthesis with a medial center of rotation and a more horizontal head-neck angle (viz., 155°) has been shown to have a higher dislocation rate than prosthesis with a more vertical head-neck angle (viz., 135°) [30]. Revision procedures have been found to have a higher instability rate regardless of the type of RTSA prosthesis system utilized [31].
Other variables, which may contribute to instability of RTSA, include the indications for surgery, technical aspects during surgery with implant-soft tissue tensioning, high patient BMI, male gender, and glenoid failure [31]. Additionally, like many of the complications after RTSA, infection may lead to instability by destruction of the soft tissues, bony erosion, and loosening of the components such that the prosthesis becomes unstable.
There are several nonoperative and operative options to address instability after RTSA. We recommend closed treatment for the first or second dislocations after RTSA. Once reduced under fluoroscopy, a thorough range of motion examination should be performed in order to determine what arm positions are associated with instability. Once the stable position of the RTSA is established, bracing or immobilization to avoid that position can be successful. Teusink et al. found that the treatment of early dislocations (defined as <90 days from the index surgery) with closed reduction and brace immobilization is a viable treatment option [32]. They found that closed reduction followed by 6 weeks of immobilization in a 30° external rotation brace resulted in 62% of shoulders stable at 28-month follow-up, while 29% continued to be unstable and required surgery. Another study by Chalmers et al. also reported around a 60% successful rate when treating instability with closed reduction and brace immobilization [33].
If closed reduction is unsuccessful and instability recurs after a previously successful closed reduction event, surgical intervention is often indicated. Operative strategies when revising a RTSA for instability include increasing the size of the polyethylene liner, increasing the height of the metal metaphyseal humeral component, and using retentive liners. Increasing the polyethylene liner thickness is a simple and often successful way to improve soft tissue tensioning via increasing offset, decreasing the socket-glenoid sphere space, and lengthening the deltoid lever arm (Fig. 20.2). Chalmers et al. were able to achieve stability in unstable primary RTSA only by increasing the polyethylene thickness in 80% of cases of instability [33].
Another strategy for surgically treating RTSA instability is to increase the size of the glenosphere. Retentive polyethylene cups may be beneficial, but they increase the rigidity of the construct, which increases concerns for implant loosening. Operative management should include a thorough evaluation of bony and soft tissue malpositioning that could cause impingement with subsequent levering of the prosthesis. If impingement is suspected, soft tissue debridement and resection of heterotopic ossification and previous bony spurs may be necessary. Unfortunately, in cases where bony and soft tissue resection is not enough to restore stability, extensive revision of the baseplate and humeral polyethylene components may be needed [34].
20.5 Scapular Notching

Scapular notching classification. Grade 1: notching below the level of the inferior screw. Grade 2: notching at the level of the inferior screw. Grade 3: notching above the level of the inferior screw. Grade 4: notching above the level of the inferior screw and involving the undersurface of the baseplate
The exact clinical significance of scapular notching is not known, but the concern is that it is a harbinger of baseplate loosening. Several studies show no significant decrease in functional outcomes or increase in implant failure rates despite the presence of notching on radiographs [38, 39]. The majority of notching seems to occur in the first 2 years after the index surgery, and studies suggest that progression after 2 years is minimal [40, 41]. Simovitch reported a 44% notching rate when using a Grammont-type prosthesis at an average of 4.5-month follow-up [40].
Nevertheless, there are documented cases of notch progression leading to implant failure as well as increased radiolucency around both humeral and glenoid implants after notching occurs [42]. One study by Levigne found that there was a higher incidence of radiolucent lines around the glenoid baseplate postoperatively when scapular notching was present [43]. Clinically however, there was no correlation between these radiolucent lines and baseplate failures. Several studies report that regardless of whether notching is present postoperatively, function and pain scores were equivalent for all groups [39, 44]. Other studies contradict these findings and show inferior clinical results when notching is present [3, 40, 45]. Mollon et al. found that patients with notching when compared to patients without notching had decreased range of motion, strength, inferior ASES, SST constant, and UCLA scores [46].
Despite the continued unclear role of scapular notching in RTSA, many strategies, including surgical technique and prosthesis design, have been developed to decrease its incidence. Nyffeler et al. performed a cadaveric biomechanical study to determine the optimal baseplate placement to avoid notching and found that inferiorly placed glenospheres develop less notching than those placed superiorly [47]. Another study confirmed these biomechanical findings by showing that optimal glenosphere positioning leads to decreased notching in a clinical setting [48]. Another technical strategy that is used to prevent notching includes placing the baseplate and glenosphere with 10–15° of inferior tilt. The belief is that increasing the prosthesis-scapular neck angle (PSNA) increases the available arc of motion before the humeral component impinges on the scapula. Gutierrez et al. showed using a computerized model that inferior tilt of the glenosphere improves impingement-free range of motion, which in theory would result in decreased scapular notching [35]. Despite these findings, clinical evidence is still lacking on whether inferior tilt prevents notching. Kempton et al. did not find any clinical benefit when comparing a neutrally versus inferiorly tilted glenospheres [49].
Prosthesis design can also affect the amount of notching. Prostheses with a lateralized central of rotation have been shown to have a decreased incidence of notching when compared to traditional Grammont-type implants with a more medial center of rotation [37, 50]. RTSA with a 145° humeral neck angle also shows decreased rates of scapular notching. The use of a larger glenosphere allows a larger surface of curvature around which the humerus polyethylene can rotate before it comes in contact with the scapular neck and is thought to prevent notching as well [51].
20.6 Glenoid Baseplate Loosening
Baseplate loosening can be either septic or aseptic, but regardless of the cause, the result can be catastrophic due to pain and wear of the glenoid bone by the loose components. The first goal when a patient has baseplate loosening is to determine which of these two processes is causing the loosening. Baseplate loosening should be presumed to be due to septic causes until proven otherwise. Consequently, blood studies (viz., erythrocyte sedimentation rate and CRP), aspiration, and, when available, metal reduction MRI should be performed.

Pre- and post-failure radiographs taken at different time intervals reveal a gross difference in baseplate positioning with associated hardware failure indicating AGBL
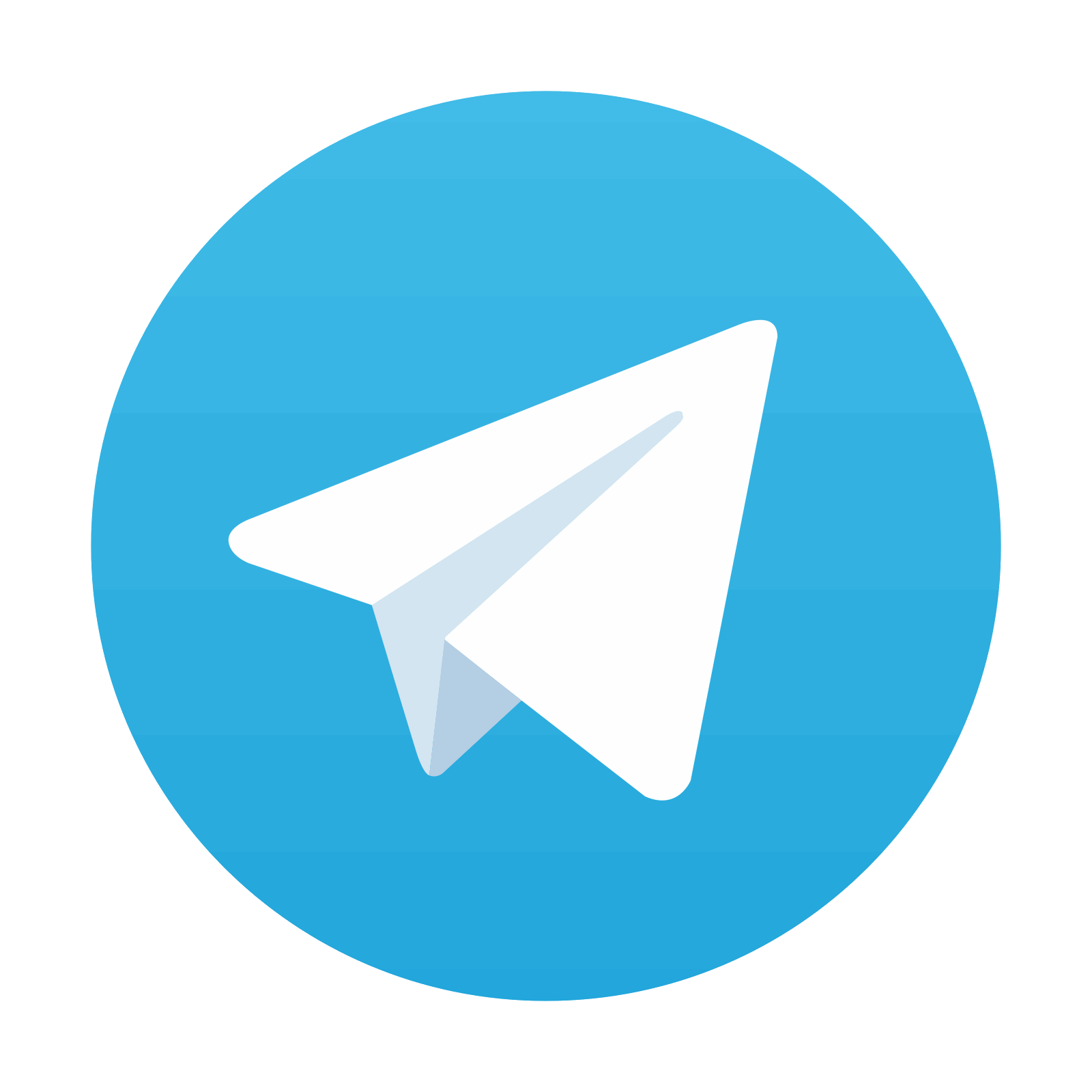
Stay updated, free articles. Join our Telegram channel

Full access? Get Clinical Tree
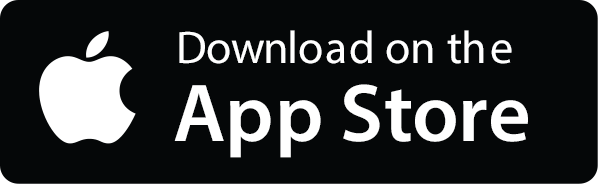
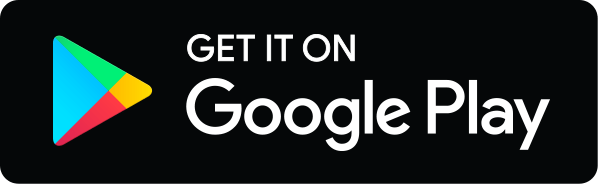