Chapter 15 Neuromuscular Training
Neuromuscular control involves the subconscious integra-tion of sensory information that is processed by the central nervous system, resulting in controlled movement through coordinated muscle activity.1 Dynamic joint stability and postural control are the result of coordinated muscle activity achieved through neuromuscular control. Any injury that disrupts the mechanoreceptors, alters normal sensory input, or interferes with the processing of sensory information may result in altered (also referred to as decreased or dysfunctional) neuromuscular control. Consequently, impairments of the neuromuscular system often result in dysfunctional dynamic joint stability and postural control. Neuromuscular control impairments can also change movement patterns and increase the risk for musculoskeletal injury. Conversely, musculoskeletal injury, by disrupting the interactions within the neuromuscular system, can be a cause of altered neuromuscular control. The authors believe an understanding of the neuromuscular control system and the functional manifestations of neuromuscular control are fundamental to designing effective treatment programs and meaningful research studies related to dynamic joint stability.
PROPRIOCEPTION
Why can one baseball pitcher exhibit excessive glenohumeral motion yet never experience injury, while another pitcher with the same glenohumeral motion require surgery in order to throw effectively without pain? Why does one athlete experience a single-episode lateral ankle sprain, while the next athlete develops chronic ankle instability? The answer to both questions is most commonly proprioception. For the healthy athlete, high levels of proprioception can contribute to enhanced neuromuscular control and functional joint stability, thus decreasing the risk for injury. For the injured athlete, restoration of proprioception is critical to ward off repetitive injury (Figure 15-1). Therefore proprioceptive training plays a key role for the athlete in both injury prevention and rehabilitation.
Proprioception may be inferred from Sherrington’s 1906 description of the “proprioceptive-system” as the afferent information from proprioceptors (e.g., mechanoreceptors) located in the proprioceptive field that contributes to conscious sensations, total posture, and segmental posture.2,3 Proprioception is a product of sensory information gathered by mechanoreceptors.2 This definition views proprioception primarily as a sensory activity. More recently, authors have expanded the definition of the proprioceptive system to include the complex interaction between the sensory pathways and the motor pathway (efferent system).4 One assumption underlying both definitions of proprioception is that incoming sensory information processed by the central nervous system (CNS) has not been compromised. In the presence of an injury that disrupts mechanoreceptor input, proprioceptive function will be compromised and may lead to movement dysfunction.
Over the years, many terms have been used either synonymously with proprioception or to describe proprioception including kinesthesia, joint position sense, joint stability, and postural control. Kinesthesia and joint position sense may both be viewed as submodalities of proprioception.3 Kinesthesia refers to the sensation of joint movement (both active and passive), while joint position sense refers to the sensation of joint position.3 Joint stability is accomplished through both passive (osseous congruity and ligamentous restraints) and dynamic (coordinated muscle contractions) mechanisms. Dynamic joint stability is the result of neuromuscular control and proprioception, while postural control is the result of integrated visual, vestibular, and proprioceptive inputs.5,6 Consequently, any disruption in mechanoreceptor input that affects proprioception will negatively affect dynamic joint stability and posture.
SENSORIMOTOR SYSTEM
Joint Receptors
Ruffini receptor endings are described as slowly adapting mechanoreceptors because they continue their discharge in response to a continuous stimulus.7 These receptors have a low activation threshold and are active during both static and dynamic joint conditions. Consequently, Ruffini endings may signal static joint position, intraarticular pressure, and amplitude and velocity of joint rotations.7–10
Golgi tendon organ-like receptors are the largest of the articular mechanoreceptors. They are also slow to adapt to stimuli, have a high activation threshold, and are active only during dynamic joint states. Some researchers have suggested that the high threshold of the Golgi tendon organ-like ending makes this receptor ideally suited for sensing the extremes of the joint’s normal movement range.11 The Golgi and Ruffini endings belong to a group called spray endings. Collectively, these mechanoreceptors represent a virtually continuous morphological spectrum of receptors.12 Whether the spray endings should be divided into distinct receptor types has not been resolved.
Pacinian corpuscles are the only rapidly adapting joint receptor. Sensitive to low levels of mechanical stress, the Pacinian corpuscle is active only during dynamic joint states. Therefore this receptor is silent during static conditions and constant velocity situations but is sensitive to joint acceleration and deceleration.13
Cutaneous Receptors
Sensory information from cutaneous (skin) receptors is processed by the CNS in conjunction with joint and muscle receptors. The role of cutaneous receptors in initiating reflexive responses, such as the flexion withdrawal reflex in response to potentially harmful stimuli, is well established.15 Cutaneous receptors may also signal information regarding joint position and kinesthesia when the skin is stretched.15,16 However, no evidence indicates that these receptors contribute significantly to these sensations17 or that cutaneous receptors contribute to joint stability.18
Muscle Receptors
The muscle spindle and the Golgi tendon organ (GTO) are the two primary types of muscle receptors.15 The muscle spindle lies in parallel with extrafusal muscle fibers and has three main components: (1) intrafusal muscle fibers, (2) sensory axons that wrap around the intrafusal fibers and project afferent information to the CNS when stimulated, and (3) motor axons that innervate the intrafusal fibers and regulate the sensitivity of the muscle spindle1,15,19,20 (Figure 15-2). The primary sensory axons from the spindle make monosynaptic connections with alpha motoneurons in the ventral roots of the spinal cord that, in turn, innervate the muscle where the muscle spindle is located.1 Collectively, this feedback loop is called the muscle stretch reflex.15 Although extrafusal muscle fibers comprise the bulk of the muscle responsible for generating force and are innervated by alpha motoneurons, intrafusal fibers are composed of a small bundle of modified muscle fibers that function to provide feedback to the CNS and are innervated by gamma motoneurons.21 Sensitivity of the muscle spindle is continually modulated by the gamma motor system. This allows the spindle to be functional at all times during a contraction21 and modulate muscle length.15,22–24 Sensory output from the muscle spindle is triggered at low thresholds, is slowly adapting, and senses joint position throughout the range of motion.15,20
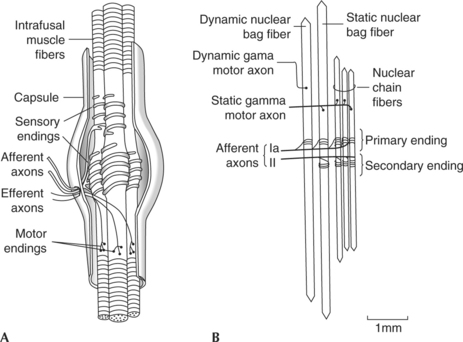
Figure 15-2 A, Gross structure. B, Structure and innervation of the intrafusal fibers of the muscle spindle.
(From Williams GN, Chmielewski T, Rudolph K, et al: J Orthop Sports Phys Ther 31:546-566, 2001.)
The GTO is located at the musculotendinous junction and is positioned in series with extrafusal muscle fibers and tendon. A single axon enters the GTO and then branches into many unmyelinated endings that are woven in and between the collagen fibers at the musculotendinous junction. Thus during a muscle contraction the tendon is stretched, straightening the collagen fibers and distorting the receptor endings of the GTO afferent neurons.25 Increased activity of the GTO afferents results in inhibition of the motor neurons innervating the muscles that were stretched while exciting the motor nerves of the antagonistic muscles. This feedback loop is often referred to as the inverse myotatic reflex. Because each organ is connected to a small number of muscle fibers and can respond to low levels of force (as little as 0.1 g),22 the GTO can provide the CNS with specific force feedback.22–24
Motor Response Pathways
Sensory information gathered by mechanoreceptors is sent to the spinal cord via afferent pathways for processing and ultimately results in a regulation of reflexes and muscle activity. In addition to being influenced by incoming afferent information, the resulting motor response also depends on the level of processing of afferent inputs within the CNS. The processing can occur at three different levels: the spinal cord, the brainstem and cerebellum, and the cerebral cortex (Table 15-1).
Table 15-1 Motor Response Intervals
Motor Response Type | Level of Mediation | Able To Be Modified |
---|---|---|
Spinal reflexes | Segmental level of spinal cord | No |
Long-loop reflexes | Brainstem and cerebellum | No |
Triggered reactions | Cortical centers | Yes |
Voluntary reactions | Cortical centers | Yes |
Spinal reflexes represent the shortest neuronal pathways, and consequently the most rapid responses to afferent stimuli. These responses are typically uniform in nature and modified by the intensity of the afferent signals.15 Spinal reflexes range from simple monosynaptic reflexes to complex multisynaptic circuits resulting in coordinated activity of groups of muscles.13,15,26 The speed of spinal reflexes is faster than ligamentous failure, yet is not considered modifiable through training to aid in dynamic joint stability.27,28
Sensory information mediated at the brainstem and cerebellum is typically referred to as a long-loop reflex.27–29 Because sensory information travels a greater distance before being processed, these responses are typically longer than spinal reflexes. However, because these pathways are multisynaptic with potentially more sources of sensory input, long-loop reflexes are flexible and may adapt when feed-forward information is provided to the system.27,28,30 As a result of both the adaptability and the relative quickness with which they occur, these pathways are thought to be important in the maintenance of dynamic joint stability.1,31
Both voluntary reactions and triggered reactions are processed at the cortical level of the brain and represent the longest motor response times. Voluntary reactions involve the processing of multiple variables and are highly flexible.27,28,32 In contrast, “triggered” reactions represent preprogrammed, coordinated reactions that occur in response to afferent stimuli that trigger them into action.1,28 One example of a triggered reaction has been termed the wine glass effect28: When one holds an expensive wine glass, one instinctively tightens the grip on it if the glass begins to slip. The cutaneous message that the object is slipping comes from the fingertips, but the muscle response to increase grip pressure comes from the forearm. In this case the reaction occurs quickly, is probably not conscious in nature, and appears to have the overall purpose of reorganizing the system slightly to complete an action successfully. Because of their preprogrammed nature, triggered reactions occur slightly more quickly than voluntary reactions but may be unable to accommodate to circumstances in atypical situations.1
POSTURAL CONTROL
Postural control involves controlling the body’s position in space for the dual purposes of stability (balance) and orientation (maintaining an appropriate relationship between the body segments and between the body and the environment for a task).6,33 The postural control system uses complex processes involving both sensory and motor components and results from the combined integration of visual, vestibular, and proprioceptive afferent inputs.5,6 The combined effort of these sensory modalities lays the framework for dynamic balance (stability). If feedback from any one of these modalities is impaired, then postural stability suffers.1
Investigators have identified several postural control strategies that result from different types of perturbations applied during stance. Typically, if forward sway is induced as a result of a posterior horizontal perturbation, muscles on the posterior aspect of the body are recruited.34 Conversely, if backward sway is induced from an anterior horizontal perturbation, muscles on the anterior aspect of the body are recruited.34 Additionally, small perturbations applied during standing result in sway at the ankle joint; this is called an ankle strategy.35 On the other hand, large perturbations result in large movements at the hip; this is called a hip strategy.35 The hip strategy is also implemented when the individual cannot generate enough force with the ankle strategy. A third strategy called the stepping strategy is implemented when the perturbation displaces the center of mass outside the individual’s base of support.6 Postural control strategies can be modified and are adaptive to the circumstances of the moment; however, in the absence of other instructions they are predictable.6 Evidence suggests that a person’s expectations of impending perturbations and training can have a significant impact on the magnitude and variability of the responses.6 These postural control strategies provide stability in stance and therefore are applicable to the maintenance of joint stability in the lower extremity during stance.1
The motor skills that people perform daily including walking pose a complicated problem for the neuromuscular system because many muscles crossing multiple joints must be coordinated to produce a given outcome. Bernstein36 called this the “degrees of freedom problem.” One theory for controlling the degrees of freedom problem is based on the concept of motor programs (a set of commands that are prestructured at higher brain centers and define the essential details of a skilled action). The most recent update to this theory, put forth by Schmidt,28 contends that features that do not vary among different skills—the relative timing, force, or sequence of components—are stored in memory as motor programs, while the parameters that do vary (e.g., speed or duration) are specified according to the task at hand. These programs are under central control and are generally not dependent on feedback from the periphery.28 Feedback is, however, used to select the appropriate motor program, monitor whether the movement is in keeping with the program, and reflexively modulate the movement when necessary.27,28
The idea of a central pattern generator is similar in concept to a motor program. These control mechanisms located in the spinal cord produce mainly genetically defined, repetitive actions, such as gait.37 The concept of the central pattern generator is supported by animal studies including spinalized models, which have shown that the rhythmic pattern of gait can continue in the absence of feedback from the limbs or descending control from the brain.38–40 Central pattern generators may be turned on or off by a variety of stimuli, although they are primarily stimulated or inhibited by signals originating in the brainstem.39–41 Although gait is centrally controlled at the lower brain and spinal cord level, descending influences from higher brain centers including the cerebellum, visual cortex, hippocampus, and frontal cortex, along with peripheral sensory input, permit effective gait even when unexpected changes in the environment are encountered.6,27,28 Thus gait is controlled through the complex interaction of central pattern generators, descending input from higher brain centers, and feedback from peripheral sensory receptors. Through this complex interaction and similar processes that occur with other motor programs, the neuromuscular system acts to maintain joint stability during dynamic situations.1
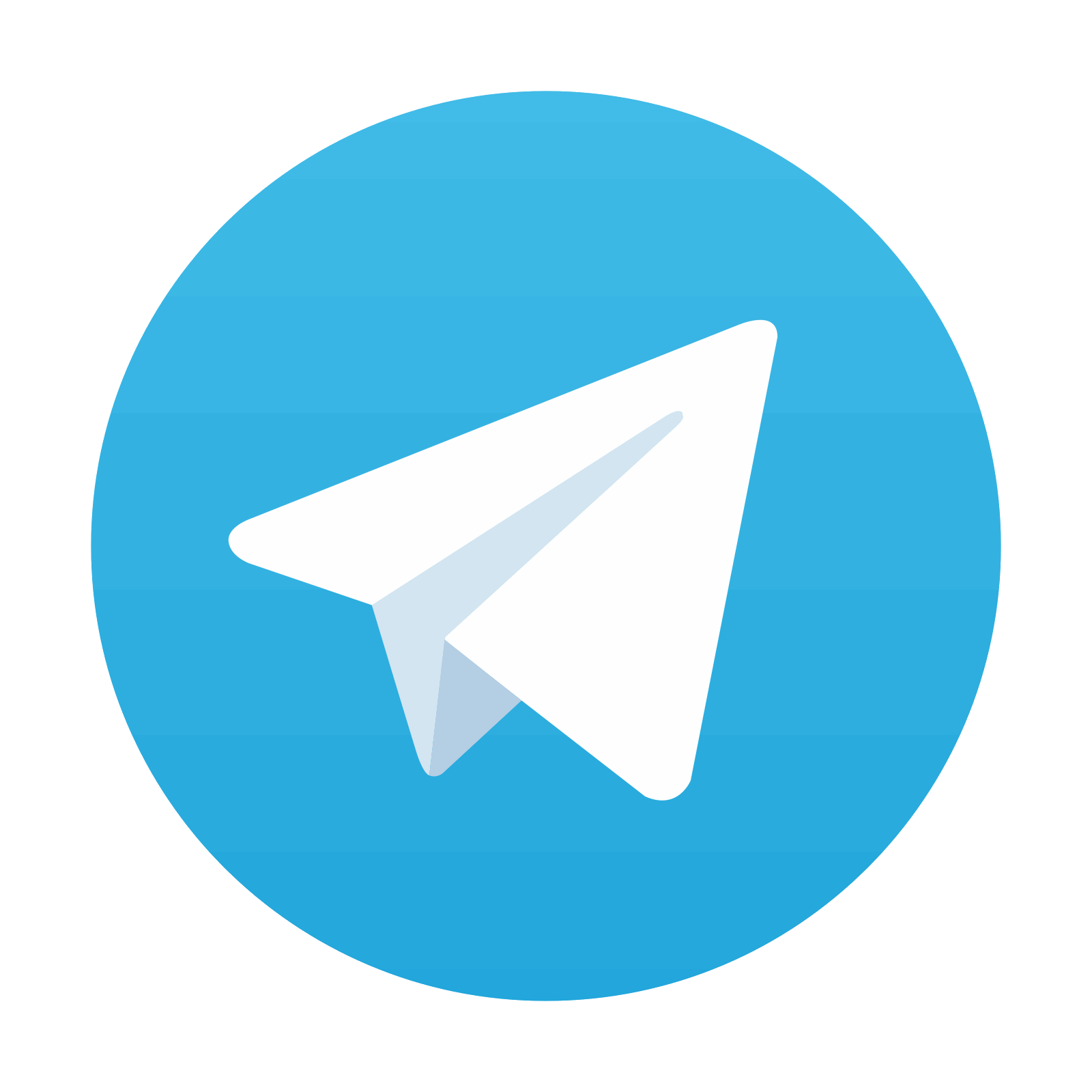