CHAPTER 7 Neurological assessment
2. Clinical overview of the function and organisation of the nervous system
3. The neurological assessment
4. Assessment of the level of consciousness
5. Assessment of lower limb sensory function
6. Assessment of lower limb motor function
7. Assessment of coordination and proprioception function
8. Assessment of autonomic function
Introduction
Why and when to undertake a neurological assessment
Neurological conditions are a primary cause of altered lower limb function, potentially leading to deformity, ulceration and in severe situations, amputation (Table 7.1). Consequently, a neurological assessment is a vital tool to determine the risk status, treatment and appropriate management for all individuals.
Table 7.1 Neurological conditions that may affect the lower limb
Condition | Description |
---|---|
Cerebral vascular accident (CVA) (stroke) | Due to haemorrhage, embolus or thrombosis of the cerebral arteries |
Parkinsonism | Degeneration of dopaminergic receptors. Usually idiopathic but can be drug induced |
Friedreich’s ataxia | One of a group of hereditary syndromes affecting the cerebellum. Inheritance is autosomal recessive. Onset in childhood, death usually around 40 years |
Multiple sclerosis | Patchy demyelination of the CNS. Shows relapses and remissions. Onset 20+ |
Poliomyelitis | Virus that affects lower motor neurones (LMNs) |
Syringomyelia | Progressive destruction of the spinal cord due to blockage of central canal, e.g. tumour |
Tabes dorsalis | Occurs with tertiary stage syphilis |
Spina bifida | Defective closure of vertebral column. Congenital |
Motor neurone disease | Degeneration of both upper motor neurones (UMNs) and LMNs. No sensory loss. Onset usually 40 and 60 years. Death usually due to respiratory infection. Idiopathic |
Subacute combined degeneration of the spinal cord | Due to lack of vitamin B12. Usually seen in pernicious anaemia. Affects both sensory and motor tracts in the spinal cord. See UMN signs, sensory and proprioceptive deficit. Reversible if detected in time |
Charcot–Marie–Tooth disease/peroneal muscle atrophy/hereditary motor–sensory neuropathy | Affects peroneal nerve, predominantly motor with variable sensory deficit. Commonest inherited neuropathy. Usually autosomal dominant. Onset in teens, slowly worsens |
Guillain–Barré syndrome | Post-viral autoimmune response, rapid onset, potentially fatal from respiratory failure. Predominantly motor effects, with muscle weakness and paralysis, but some sensory loss; 80% of patients show full recovery. Also chronic relapsing form |
Neurofibromatosis | Autosomal dominant condition that leads to tumours of nerves and compression of spinal cord |
Peripheral neuropathy | Occurs due to a variety of causes, e.g. alcoholism, injury, diabetes mellitus |
Myasthenia gravis | Autoimmune disease that affects the neuromuscular junction and leads to severe fatigue and weakness/paralysis |
Myopathies | Range of relatively rare diseases affecting muscle only. May be inherited or acquired. Symptoms similar to LMN diseases but no fasciculation |
To achieve this, the practitioner needs to link pertinent information from an assessment to possible underlying causes or abnormal responses. Indeed, the practitioner needs to think much like a clinical detective, allowing a diagnosis to be made based on a sound understanding of physiology, rather than matching the ‘evidence’ to a potential culprit. This allows the diagnosis to be clinically meaningful, for example, rubor and heat in the diabetic foot need not be dismissed as cardinal signs of infection. On the contrary, increased vasodilation due to autonomic dysfunction may in fact indicate Charcot’s foot. Hence, it is imperative that the clinician appreciates all potential diagnoses, and is capable, through a process of discriminatory assessment, to match physiology to pathology. In the above example, infection would remain a prime suspect, allowing appropriate intervention to save the individual from likely ulceration and amputation.
The National Institute of Health and Clinical Excellence (NICE; www.nice.org.uk) has produced guidelines stating that all individuals with diabetes mellitus should receive annual neurological screening. In addition, elderly patients may require further neurological monitoring, given that studies by Mold et al (2004) found that 26% of healthy individuals between 65 and 75 years displayed altered neurological status. The reported incidence in the ≥85 years age group was increased to 54%. Accordingly, although all new patients require an initial assessment, high-risk groups should receive a full review twice yearly under ideal circumstances.
Clinical overview of the function and organisation of the nervous system
Organisation of the nervous system
The nervous system can be organised on the basis of either its anatomical location (central and peripheral) or its function (somatic and autonomic) (see Fig. 7.1). However, while these classifications exist independently in text books, in reality, they function as a coordinated whole. For example, when learning to drive, we know that the clutch and accelerator are important, but only when they are in the correct combination will the car move effectively.
Building blocks of the nervous system
When conducting an assessment, it is important to know what one is assessing. Neurones are one of the primary building blocks of the nervous system (Fig. 7.2). They facilitate rapid communication by transmitting impulses from one to the next or to other excitable tissue, i.e. muscle, at a velocity of up to 100 m/second (Bawa et al 1984) (see Fig. 7.3).
Anatomical classification
Central nervous system
The central nervous system (CNS) contains all the structures lying within the brain and spinal cord. It consists of neurones and neuroglial (supporting) cells. The brain can be divided into forebrain, midbrain and hindbrain and is covered by the three meninges, protected by the cranium (Fig. 7.4). On average, it weighs approximately 1.3 kg. The parts of the brain and their main functions are listed in Table 7.2.
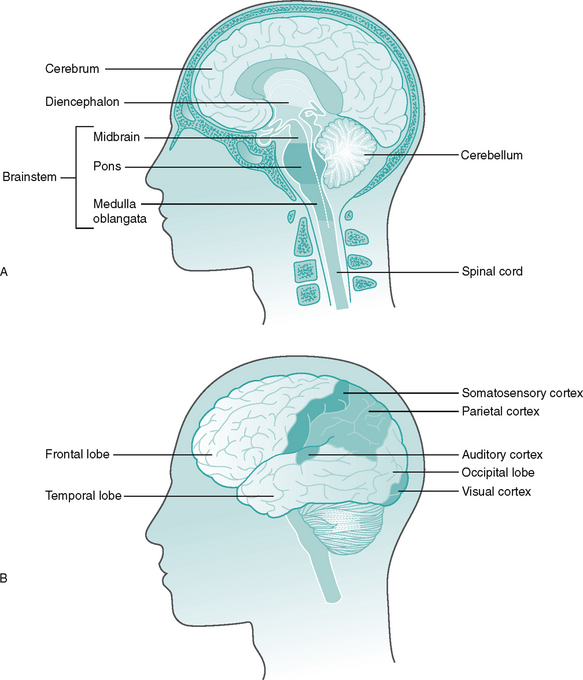
Figure 7.4 The brain A Anatomy of the brain B Position of the lobes and cortex.
(Adapted from Vander et al 1998.)
Table 7.2 Areas of the brain and their function
Area | Function | |
---|---|---|
Forebrain | Cerebral cortex | |
Diencephalon | ||
Midbrain | Corpora quadrigemina | |
Hindbrain |
The cerebral cortex (cerebrum) consists of two hemispheres (right and left) and is principally associated with sensory perception and voluntary motor activity, the somatosensory cortex (see Fig. 7.4). Damage to this area following a cerebrovascular accident (CVA), can result in features classically associated with this condition, i.e. spasticity, altered sensation and speech. The cortex is highly convoluted, which increases its surface area and, therefore, the number of neurones it contains – an estimated 102 783 000 synapses! The cerebellum consists of two hemispheres which are primarily composed of the anterior and posterior lobes and is a vital centre for the coordination of voluntary movement, posture and balance. Hence, an ataxic (uncoordinated) gait may be an indicator of a lesion to the cerebellum.
The spinal cord is enclosed in the 32 vertebrae of the spinal column (Fig. 7.5). In cross-section, it is composed of a grey centre, similar in shape to the letter H with a white outer area (Fig. 7.6A). The grey matter is composed of the cell bodies of the neurones. Ascending sensory axons to the brain and descending motor axons from the brain form the white matter. Ascending and descending axons are arranged into columns or tracts.
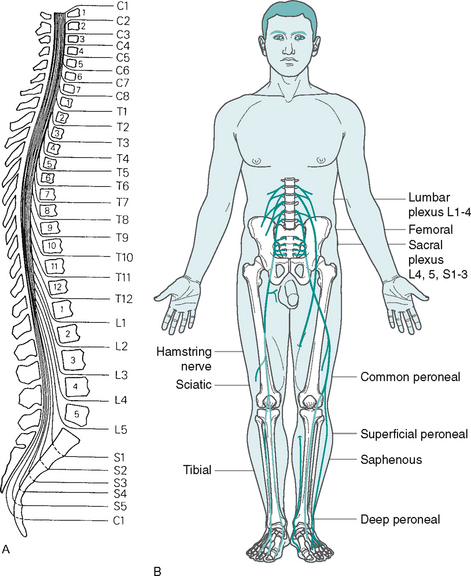
Figure 7.5 The spinal cord A Relationship of vertebrae to spinal cord segments (reproduced from Matthews & Arnold 1991, with permission) B Organisation of the lumbar and sacral plexi and innervation of the lower limb
(Adapted from McClintic 1980.)
Peripheral nervous system
• afferent (sensory) nerve fibres – carry impulses towards the CNS from receptors, e.g. pressure and vibration receptors
• efferent (motor) nerve fibres – carry impulses away from the CNS to effectors, e.g. muscles or sweat glands.
The afferent and efferent nerve fibres are arranged into 12 pairs of cranial and 31 pairs of spinal nerves. The spinal nerves emerge from the spinal cord as two roots, a dorsal (posterior) and a ventral (anterior) root, which join to form the peripheral mixed spinal nerve (Fig. 7.6A). The dorsal root ganglion contains the cell bodies of afferent (sensory) fibres. Incoming sensory information to the spinal cord is transmitted along an afferent neurone in a mixed nerve, into the dorsal root and enters the dorsal horn of the spinal cord, where it synapses with either an internuncial or motor neurone (Fig. 7.6B). The ventral root contains mainly efferent (motor) fibres, the cell bodies lying within the ventral and lateral horns of the spinal grey matter (Fig. 7.6A).
The spinal nerves are mixed, as they contain both afferent and efferent fibres, from both the somatic and the autonomic nervous system (see Fig 7.6B). Hence, damage to a spinal nerve may affect autonomic function (e.g. loss of bladder control) as well as motor and sensory function, depending on the site of damage. A dermatome is defined as an area of skin supplied by a single nerve’s dorsal root. Dermatomes overlap each other by up to 30%, so that following damage to a spinal nerve, loss of sensation in that area is limited, as adjacent dermatomes will respond to stimuli. The dermatomes of the lower limb are shown in Figure 7.7.
Functional classification
Somatic nervous system
1. Sensory – afferent nerves detect changes (stimuli) in the external environment and its relation with the body.
Stepping barefoot onto a drawing pin triggers a pain response.
2. Integrative – interprets and stores sensory information within the central nervous system (CNS).
The pain response will be recognised and processed.
3. Motor – efferent nerves produce a response, muscle contraction or glandular secretion.
Muscle contraction produced causing the foot to be rapidly withdrawn.
Autonomic nervous system
The afferent neurones of the ANS travel with those of the somatic system, although the receptors are situated in internal organs, i.e. baroreceptors of the carotid sinus. The efferent branches of the ANS differ from those in the somatic system, as there are two neurones (preganglionic and postganglionic) in each pathway. The first cell body lies within the CNS, with the second in the autonomic ganglia outside. The efferent outflow is divided into the sympathetic and parasympathetic systems (Fig. 7.8):
• Parasympathetic nervous system
This is the efferent branch of the autonomic nervous system, which prepares the body for action – the fight or flight response. The cell bodies of the preganglionic neurones are found in the lumbar and thoracic regions of the spinal cord (see Fig. 7.5B). Where an effector organ receives dual innervation (sympathetic and parasympathetic), the two branches usually act antagonistically, in a push–pull or accelerator–brake fashion.
Sensory pathways
The various changes in the internal and external environments are detected by receptors. Receptors are found within specialised organs, such as the rods and cones of the eye, and are also distributed throughout the body, for example the pain receptors of the skin and gut. Receptors within the specialised organs contribute to the complex senses of sight, hearing, taste and smell. Exteroceptors in the skin detect pressure, touch, temperature, pain and position sense in relation to the external environment. They are termed somatic receptors (Table 7.3). These receptors and their associated pathways form an integral part of the neurological assessment.
Table 7.3 Classification of the somatic receptors
Category | Sense | Receptor |
---|---|---|
Mechanoreceptor | Touch, pressure | Encapsulated and free nerve endings |
Thermoreceptor | Warmth, cold | Free nerve endings |
Nociceptor | Pain | Free nerve endings |
Proprioceptor | Position | Encapsulated nerve endings |
Interoceptors detect stimuli in the body’s internal environment that do not usually reach consciousness. They communicate with subconscious levels of the brain. Baroreceptors located in the walls of arteries and veins are a classic example of interoceptors; they monitor internal changes in blood pressure. Receptors in the muscles, tendons and joints registering position, sense, tension and degree of stretch are called proprioceptors. They send impulses both to the cerebellum, which is part of the unconscious brain, and to the part of the conscious brain called the somatosensory cortex.
The area served by a sensory unit (afferent nerve, its branches and the attached receptors) is called the receptive field (Fig. 7.9). A possible analogy for the receptive field is that of an umbrella. The spokes (receptors) lie beneath a waterproof skin (body tissues) and make up a frame offering coverage and protection for a specific area (receptive field). The framework feeds into a central strut (afferent nerve) and terminates at the handle (synapse to CNS). Receptor fields may overlap and the density of receptors may vary in different regions of the body. The precision with which a stimulus can be located and differentiated depends on the size of the receptive field and its density. For example our fingers and thumbs are very good at detecting stimuli from the external environment as the receptive fields in them are small and dense.
The peripheral afferent (sensory) pathway is the name given to the pathway from a receptor to the CNS. Stimuli detected in the periphery will initially be conveyed by afferent neurones to the spinal cord, where it may synapse with one or more neurones in the dorsal horn. Alternatively, if further integration and interpretation is required, the information must reach the appropriate part of the brain. The information travels to these higher centres in ascending tracts or columns of the white matter of the spinal cord (Fig. 7.10).
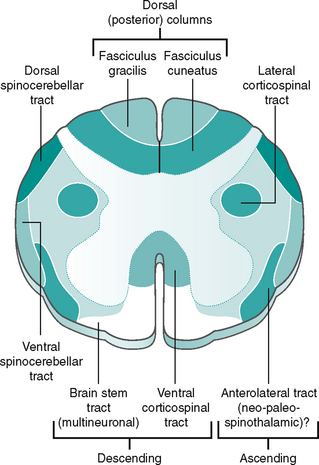
Figure 7.10 Transverse section of the spinal cord showing ascending and descending pathway
(Adapted from McClintic 1980.)
There are two main ascending tracts (Budd 1984):
Most of the tracts are named according to their origin and destination, i.e. the spinothalamic tract, carrying pain information, runs from the spinal cord up to the thalamus, an important sensory relay station in the brain. The ascending tracts from receptor to sensory cortex involve three neurone links (Fig. 7.11):
1. First-order neurons – convey impulses from the receptors to spinal cord or brain stem
2. Second-order neurons – convey impulses from the spinal cord and brain stem to thalamus
3. Third-order neurons – convey impulses from the thalamus to the somatosensory cortex, resulting in conscious perception.
The information carried by the three-neurone link is simultaneously transmitted to the cerebellum and brain stem (unconscious) by off-shoots (collaterals). The ascending tracts and their function are given in Figure 7.11.
The majority of ascending tracts cross over to the opposite (contralateral) side of entry, either in the spinal cord or in the brain stem, synapsing with areas of the thalamus before continuing to the conscious sensory cortex. Neurological damage to the spinal cord may produce numbness on either the contralateral side, if the site of damage is before the tracts cross, or the ipsilateral (same) side, if the site of damage occurs after the tracts have crossed. The organisation continues in the brain, allowing specific areas of the cortex to receive the information from the various parts of the body (Fig. 7.12). This is called somatotopic organisation. This arrangement explains why a stroke/CVA affecting a particular part of the sensory cortex produces numbness or paraesthesia in a particular part of the body on the opposite (contralateral) side.
Motor pathways
Just as all conscious stimuli are interpreted in the cortex, so all conscious actions originate there. The whole area is known as the sensorimotor cortex; one part is called the primary motor cortex and initiates conscious action (Fig. 7.12B). Damage to this region affects particular actions on the contralateral (opposite) side of the body. Close to this area is the premotor cortex, which is involved in the planning of actions. Since the actions produced by these neurones are the conscious movements of the body, the muscles involved will be skeletal and the neurones part of the somatic motor system.
The descending pathways from brain to spinal cord can be divided into two main tracts: the rapid corticospinal tract and the slower multineuronal tract (Fig. 7.13).
The corticospinal (lateral and anterior) tract is mainly responsible for the skilled movements of small, distal limb muscles, such as those used in dexterity. Most of the fibres, but not all, cross over in the brain stem and descend in the white matter of the spinal cord. The corticospinal tract forms a rough pyramid shape as it passes through the brain stem, hence is termed the pyramidal tract. Its location within the spinal cord can be seen in Figure 7.10.
The multineuronal tract (extra pyramidal) mainly influence the large, proximal limb muscles and the axial muscles of posture, having a predominantly inhibitory effect on the ventral horn cells (Fig 7.13). Consequently, damage to UMNs can lead to loss of the inhibition or ‘damping down’ effect on the LMNs, producing ‘hyper-reflexia’ or an exaggerated patellar reflex response. They are also responsible for the anti-gravity reflexes, which keep our knees extended and head erect, so maintaining upright posture. Although influenced by UMNs, the tracts are only recognisable as separate pathways, emerging from the brain stem to travel through the spinal cord as the vestibulospinal, tectospinal and reticulospinal tracts.
Basal ganglia
At present, the precise functions of the basal ganglia in movement are unknown, but they are thought to enable abstract thought (ideas) to be converted into voluntary action (Ganong 1991, p 200). Like the cerebellum, they function at an unconscious level and have no direct pathway to LMNs, but influence the sensorimotor cortex and the descending reticular formation. As the main action of the basal ganglia is on the descending extrapyramidal tracts, they have become known as the ‘extrapyramidal system’ and conditions affecting them are referred to as extrapyramidal syndromes, most significant among them being parkinsonism.
The final common pathway of conscious action is excitation of an LMN in the ventral (anterior) horn of the spinal cord and passage of an impulse along its axon in the spinal nerve to the skeletal muscle (Fig. 7.14). A single LMN can be subject to many simultaneous excitatory and inhibitory influences from many neurones, approximately 10 000–15 000 synapses. If the sum of these influences is excitatory, the LMN will be stimulated to discharge an impulse along its axon, causing the skeletal muscle to contract.
Reflexes
Reflex arcs
The pathway between receptor and effector is called a reflex arc, has five key elements and involves the CNS, although not always the brain (Fig. 7.15 and Box 7.1). Additionally, there are three reflexes of particular importance to the function of the lower limb:
Box 7.1 Essential elements of a reflex arc
1. A detector to detect the change (stimulus) in either the internal or external environment
2. Afferent neurones that send the information into the CNS along the afferent pathways
3. An integrating centre to match the appropriate response to the stimulus. This will be in the brain or spinal cord. Different parts of the CNS communicate with one another via ascending and descending pathways
4. Efferent neurones that carry instructions from the CNS via efferent pathways to the effectors (skeletal, smooth or cardiac muscle or gland)
Pain withdrawal reflex (see Fig. 7.16)
1. Injured cells release local chemical mediators that sensitise the nociceptors.
2. Proportional to the damage done, impulses are transmitted along the afferent pathways to the CNS (in this case, the spinal cord).
3. The afferent neurone activates internuncial neurones in multiple segments (point of entry, ascending and descending) of the spinal cord.
4. Efferent neurones (LMNs) transmit impulses from the CNS to the skeletal muscles (effectors).
5. The neurotransmitter, acetylcholine, is released by the efferent neurones and combines with receptors at the motor endplate on the muscle fibres, causing muscle contraction and withdrawal.
The presence of more than one synapse in the reflex arc means that the arc is described as polysynaptic. The reflex is ipsilateral, as sensory (afferent) input and motor (efferent) output both occur on the same side of the spinal cord.
Crossed extensor reflex (see Fig. 7.17)
1. A painful stimulus triggers the nociceptors (pain receptors).
2. Afferent neurones transmit impulses to the CNS.
3. The afferent neurones activate both ipsilateral and contralateral internuncial neurones within the spinal cord, at several levels.
4. Many efferent neurones (LMNs) become excited and their antagonists inhibited. This ensures that the flexors of the injured limb contract while the extensors relax, whereas in the contralateral limb the flexors are inhibited and the extensors contract to provide a rigid support.
5. Acetylcholine is released by the efferent neurones at the motor endplate causing muscle contraction.
Stretch reflex (see Fig. 7.18)
1. Striking the tendon with the patella hammer causes stretching of the muscle and activates specialised receptors (muscle spindles).
2. The stretched muscle spindles generate impulses which travel along the neurone towards the CNS.
3. The neurone enters the spinal cord and synapses directly with a motor (efferent) neurone in the anterior horn and an inhibitory internuncial neurone.
4. The excited efferent neurone (LMN) transmits the impulse from the CNS along its axon to the stretched extensor skeletal muscle fibres. The inhibitory internuncial neurone inhibits the antagonistic (flexor) muscles.
5. Acetylcholine is released by the efferent neurones and the extensor muscle contracts, while the flexor muscles relax, relieving the detected stretch.
Coordination and posture
Table 7.4 presents a summary of the various parts of the nervous system involved in posture, balance, gait and coordination of motor activity.
Table 7.4 Role of CNS in posture, balance and coordination
Action | Site | Function |
---|---|---|
Motor coordination | ||
Posture and balance | Cerebellum | Rich input, miniprogrammes |
Gait | All of above |
The neurological assessment
Clinical signs
The patient should be observed while walking, sitting and speaking, as well as while performing particular tasks. A change in the level of consciousness, inability to follow simple instructions and deficits in voluntary movement or sensation, including the presence of pain, all provide important clues. Important points to note are listed in Box 7.2
In-depth medical history
It is important to undertake a thorough medical and social history (Ch. 5). In particular, the following should be borne in mind. Onset and duration may provide vital clues as to the cause of a problem. For example, Guillain–Barré syndrome has a sudden, postviral onset.
A history of frequent falls with no loss of consciousness suggests a lesion in one of the areas of the brain dealing with balance and posture, such as the cerebellum or basal ganglia. Such episodes can be seen in patients with Parkinson’s disease or in multiple sclerosis (Table 7.5). Where loss of consciousness has occurred, the period of unconsciousness and the age of the patient should be taken into account prior to diagnosis. The presence of a severe headache is an important sign, since, although it often has a completely benign cause, it may also indicate a more serious event, e.g. brain tumour or subarachnoid haemorrhage or the less sinister migraine or tension headache. Consequently, it is vital that the onset, nature and duration of the ‘headache’ should be established.
Table 7.5 Common neurological conditions associated with falls
Condition | Clinical features affecting the lower limb |
---|---|
Parkinson’s disease | Shuffling and narrow based gait with small rapid steps. Loss of arm swing with difficulty initiating movement and turning quickly. Rigidity of leg extensors. A backward step may be taken to prevent falling when stopping |
Peripheral sensory neuropathy | Protective ‘withdrawal’ to pain response may be lost. Broad-based, high stepping, stamping gait and increased plantar pressures due to a loss of proprioception and sensory input. Darkness or removal of other sensory information exacerbates this condition, i.e. Romberg’s test (ask patient to close their eyes and note if they become unstable) |
Distal weakness | Damage to LMN can lead to limb being lifted up or over objects, i.e. lifting limb onto treatment couch. Foot drop and foot slap may occur, if the ankle dorsiflexors are impaired (particularly tibialis anterior). A waddling gait may develop and there is difficulty in rising from a seated position |
Cerebellar ataxia | A broad-based, unstable, tremulous gait often described as a ‘drunken’ stagger. Gait often leans towards the affected cerebellar side. Falls tend to occur backwards or sideways |
Spasticity | Associated with CVAs and UMN lesions. Extension of the hip and knee with a plantarflexed inverted foot leading to a limb length discrepancy. Toe box and lateral border of shoes become scuffed. Usually unilateral presentation with a circumductory gait for affected limb. |
General physiology
The causes of neurological disorders are many and can be classified as follows:
• Heredity, e.g. Huntington’s chorea, peroneal muscular atrophy, Friedreich’s ataxia, malignant hyperpyrexia
• Developmental defect, e.g. spina bifida, syringomyelia
• Trauma, e.g. severing of the spinal cord or a peripheral nerve, concussion
• Ischaemia, e.g. stroke, cerebral haemorrhage
• Compression, e.g. tumour of the cerebellum, Morton’s neuroma, common peroneal nerve palsy
• Infection, e.g. human immunodeficiency virus (HIV) infection, Creutzfeldt–Jakob disease, herpes zoster (shingles), Guillain–Barré syndrome, lepromatous neuropathy
• Autoimmune, e.g. myasthenia gravis, polymyositis, possibly multiple sclerosis
• Nutritional/metabolic, e.g. Korsakoff’s psychosis, subacute combined degeneration of the spinal cord, diabetic neuropathy
• Iatrogenic, e.g. tight plaster cast causing nerve palsy, drug-induced myopathies (lithium, high-dose steroids, etc.)
• Idiopathic, e.g. Parkinson’s disease, motor neurone disease, non-familial Alzheimer’s disease.
The effects of any lesion in the nervous system will depend on the area involved. For example, occlusion of the posterior cerebral artery, which feeds the occipital lobe of the brain (striate cortex), may result in visual disturbances, while occlusion of a cerebellar artery may elicit ataxia and an occlusion of the vasa nervosum of a peripheral nerve may effect a ‘glove and stocking’ paraesthesia. Nerve function deficit is called neuropathy and is classified according to the numbers and types of nerves involved and the site of the lesion (Table 7.6).
Table 7.6 Classification of neuropathies
Type of neuropathy | Description |
---|---|
Mononeuropathy | Abnormality of a single nerve |
Mononeuritis multiplex | Asymmetrical abnormality of several individual nerves |
Radiculopathy | Abnormality of a nerve root |
Polyneuropathy | Widespread, symmetrical abnormality of many nerves, usually characterised as sensory/motor/autonomic ‘glove and stocking’ distribution |
Assessment
Many neurological conditions present with multiple signs and symptoms because more than one part of the nervous system is affected. It is important to bear this in mind when assessing each of the above parts, ensuring that all the information is viewed contextually to facilitate a definitive diagnosis. For example, multiple (disseminating) sclerosis is a progressive disease affecting the CNS, where repeated patchy demyelination of nerve sheaths occurs, leading initially to a temporary and later, permanent loss of function. The nerve axons most often affected are the optic nerves – the optic nerve is considered an outgrowth from the CNS rather than a peripheral nerve. Multiple sclerosis along with Parkinson’s disease is the second most common disease to affect the CNS, after strokes (Wilkinson 1993, p 135). Similarly, neurological disturbances in the lower spinal cord can be betrayed via blurring of vision (diplopia), unsteady gait, weakness in the lower limbs, lower limb sensory loss and/or disturbances of micturition.
The type of pain and its distribution can help to establish whether the problem affects a nerve pathway or is referred via entrapment of a spinal nerve. Patients should be asked, if their limbs feel weak or sluggish (paresis): a slow, progressive onset of muscular weakness suggests muscular dystrophy, whereas an acute onset suggests a demyelinating disease. Alternatively, a burning, lancinating pain with allodynia (normal touch stimuli perceived as painful) indicates a neuropathic origin, such as painful peripheral diabetic neuropathy.
Social habits
Smoking and alcohol consumption should be noted. Smoking is a risk factor for certain conditions, such as atherosclerosis and therefore, CVAs. Chronic alcoholism can affect both motor coordination and memory (Korsakoff’s psychosis). Indeed, the actual cause is an alcohol-induced thiamine deficiency, which damages the limbic system (Wilkinson 1993, pp 49–50). Such patients appear alert and fully conscious, but recent memory of time and place is severely impaired. The patient denies any loss of memory and frequently attempts to disguise the deficit by confabulation. Similarly, indications of a lifestyle consistent with a risk of contracting HIV infection, such as intravenous drug misuse, may explain neurological deficit; the infection can produce a progressive encephalopathy.
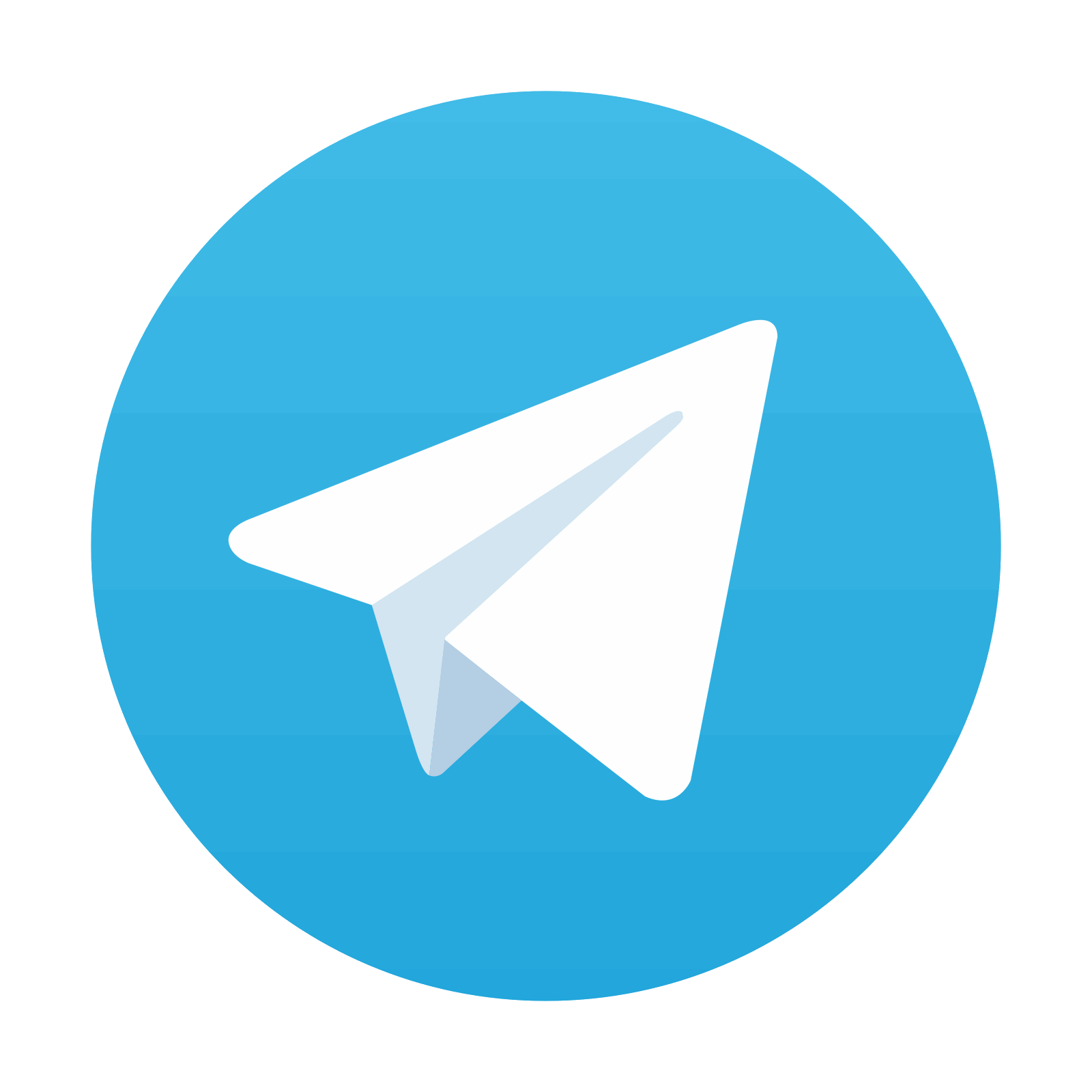
Stay updated, free articles. Join our Telegram channel

Full access? Get Clinical Tree
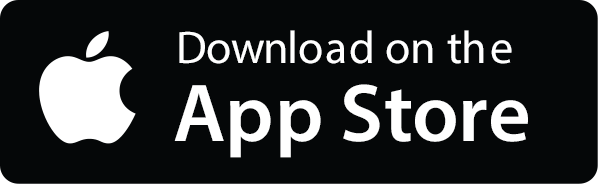
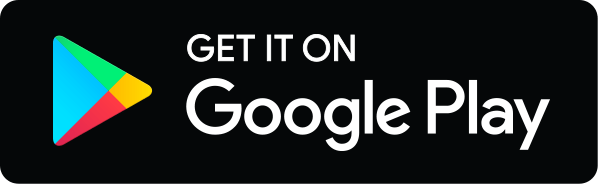