Fig. 6.1
Hormonal and other changes in patients with hypothalamic amenorrhea. In patients with hypothalamic amenorrhea, there are alterations of hormones and other factors that affect the secretion of gonadotropin-releasing hormone (GnRH), including low levels of leptin and high levels of both ghrelin and neuropeptide Y (NPY). Β-endorphin, corticotropin-releasing hormone (CRH), dopamine, and γ-aminobutyric acid (GABA) are factors that negatively influence GnRH secretion. Some of these factors may also serve as hunger signals from the peripheral to the central nervous system and as links between nutrition and reproduction. Hallmark findings in adolescents and young women with hypothalamic amenorrhea include overactivity of the hypothalamic–pituitary–adrenal axis, suppression of the hypothalamic–pituitary–adrenal axis, and alterations in thyroid hormone regulation. FSH follicle-stimulating hormone, LH luteinizing hormone, TSH thyrotropin, and T 3 triiodothyronine. (Reprinted from Gordon CM. Clinical practice. Functional hypothalamic amenorrhea. N Engl J Med. 2010;363(4):365–71, with permission from Massachusetts Medical Society)
Luteinizing Hormone
LH is secreted by gonadotropes in the anterior pituitary and is essential for reproduction. In females, LH is critical for ovulation (the “LH surge”), stimulation of estradiol precursor production (theca cell production of androgens), and maintenance of the corpus luteum. The gonadal hormones released as a result of the coordination of GnRH, LH and FSH, have modulating effects on both the hypothalamus and pituitary. For further details about normal menstrual function, please see Chap. 3.
In a study of 49 adult women with FHA (primary or secondary amenorrhea), the LH secretory patterns over 12–24 h were compared to those during the early follicular phase of normally cycling women. The range of abnormal LH pulses in those with FHA included 8 % apulsatile, 27 % low frequency/low amplitude, 8 % low amplitude/normal frequency, 43 % low frequency/normal amplitude, and 14 % normal frequency/normal amplitude pulses [7]. In comparing 11 pm with 8 am LH secretory parameters of 14–21 year old amenorrheic athletes, eumenorrheic athletes, and eumenorrheic, nonathlete controls, overnight secretory LH pulse height, total pulsatile secretion, and area under the concentration time curve (AUC) were lower in the amenorrheic athletes versus controls [9].
Loucks et al. compared the 24-h patterns of LH pulsatility in eumenorrheic, sedentary women with decreased energy availability from two study-imposed conditions: dietary restriction alone versus dietary restriction and exercise combined. In the exercising group, low energy availability decreased LH pulse frequency by 10 % during waking hours, and increased LH pulse amplitude by 36 % during waking and sleeping hours. The decrease in LH pulse frequency was much less severe than the decrease seen with energy deficiency from diet alone. When energy availability was 45 kcal/kg of lean body mass/day, the stress of exercise did not lower LH pulse frequency nor increase pulse amplitude. This study supports the theory that decreased energy availability is a key component to GnRH and LH disruption in FHA, rather than exercise stress, at least in an acute setting [10].
Follicle-Stimulating Hormone
FSH, like LH, is produced by gonadotropes in the anterior pituitary and is essential for reproduction. During the early follicular phase of the menstrual cycle, FSH simulates granulosa cells to convert androgens from thecal cells to estradiol, and stimulate folliculogenesis. As inhibin B levels rise, a product of the granulosa cells, FSH production is inhibited and levels fall in the late follicular phase, typically allowing just one follicle to proceed to ovulation. Toward the end of the luteal phase, progesterone and estradiol concentrations fall, allowing FSH to rise again as the next ovulatory cycle begins. For further details about normal menstrual function, please see Chap. 3.
FSH release is at least partially controlled by GnRH pulsatility. It has been assumed that FSH deficiency acts together with the disruption of LH pulsatility to impair reproductive function in FHA. However, while extensive work has demonstrated abnormalities in LH pulsatility in amenorrheic athletes, changes in patterns of FSH release have not been well elucidated. One study of daily urinary hormonal levels over 3 months in eumenorrheic, recreational runners reported that the elevation in FSH levels during the luteal–follicular transition was lower in athletes with luteal phase defects (shortened luteal phase) versus sedentary and exercising subjects with ovulatory cycles [11]. Over the course of the 3 months, all sedentary controls in this study had consistent menstrual cycle phases, with 90 % of all cycles being ovulatory. However, in the exercising women, only 45 % of cycles were ovulatory, 43 % had luteal phase defects, and 12 % were anovulatory [11].
In a study of patients with FHA versus eumenorrheic controls, only a minority of patients with FHA had a low serum basal FSH level [12]. These lower levels were associated with fewer 6–9 mm follicles in the ovaries, based on ultrasonography. However, despite normal serum FSH levels and normal numbers of 6–9 mm follicles in the majority of the FHA subjects, the functional follicle markers of FSH sensitivity, inhibin B and anti-Müllerian hormone (AMH), were noted to be abnormal, losing some of the correlations to FSH and follicle numbers expected and seen in controls. This suggests that FSH action on the ovary is incomplete and is not accurately reflected by a serum level nor by follicle number noted on ultrasound [12].
Gonadal Hormones
Estrogens
FSH stimulates ovarian production of estrogens by granulosa cells. In addition, estrogens are produced in smaller amounts in adipose tissue, the liver, adrenal glands, and the breasts. Estrogens play important roles in a myriad of physical functions including development of female secondary sexual characteristics, reproduction (by building the endometrial lining and causing the LH surge), sexual desire, coagulation, cardiovascular health, and bone health. While the numerous functions of estrogen are beyond the scope of this chapter, it is important to remember its role in skeletal health, noting that estrogen acts primarily as an antiresorptive hormone, inhibiting osteoclast activity. The greatest accretion of lifetime bone mass is achieved during puberty [13]. Therefore an insufficiency of estrogen during the critical pubertal years can have a profoundly negative effect on a young woman’s skeletal health. Estradiol levels in female athletes have correlated highly with lumbar, hip, and whole body BMD in various studies [14]. Please see the following chapters in this book: Chaps. 4, 5, 8, 10.
As with other causes of FHA, it is well established that estradiol levels are lower in amenorrheic athletes compared to eumenorrheic athletes and nonathletes [15, 16]. When 3 months of daily urinary estrone-1-glucuronide (E1G) excretion patterns were studied in women grouped by exercise status (sedentary or exercising) and menstrual status (ovulatory, luteal phase defect, or anovulatory), the variability in estrogen patterns was interesting. There were no significant differences in peak concentrations of E1G excretion among groups. However, the day of the E1G peak was significantly later in exercisers with luteal phase defects compared to exercisers who ovulated. Exercisers with anovulatory cycles had lower E1G excretion in the early follicular phase versus those who were sedentary and ovulatory, and had lower E1G over the entire follicular phase compared to sedentary and exercising ovulators and exercisers with luteal phase defects. During days 6–12, E1G excretion was lower in anovulatory exercisers versus sedentary and exercising ovulators, and the AUC for E1G during the follicular phase was lower, as well. During the luteal phase, E1G AUC was lower in anovulatory exercisers and those with luteal phase defects compared to sedentary ovulating women [17]. Further research is needed to determine the importance of subtle changes in estrogen and other hormone levels in eumenorrheic athletes with luteal phase defects and anovulatory cycles.
While the importance of estrogen for bone health has been established, and there is well-founded concern regarding bone health risks in those athletes with FHA, there have been mixed results in studies assessing the effects of oral contraceptive pills on BMD. Some have shown an increase, some have shown a decrease, and some have found no change in various bone parameters, including BMD [18]. In a study of adolescent girls with anorexia nervosa (AN) and normal weight controls (C), our group found that at baseline, all BMD measures (as assessed by DXA) were lower in AN versus C. When physiologic estradiol replacement and cyclic progesterone was given to some of the AN patients, those who received transdermal estradiol patch had significant increases in spine and hip BMD over the 18 month study [19]. Unlike oral contraceptive pills, estradiol given as a transdermal patch does not suppress IGF-I levels, which is anabolic to bone [20]. Transdermal estrogen studies in Triad patients may help determine if some hormonal replacement in conjunction with improved energy availability could enhance bone health.
Progesterone
Progesterone, or pregn-4-ene-3,20-dione (P4), belongs to a class of hormones called progestogens, and is the major naturally occurring human progestogen. P4 is produced by the ovaries, the adrenal glands, and during pregnancy by the placenta. It is stimulated by LH and its effects are enhanced by estrogens. Like all human steroids, P4 is a by-product of cholesterol. In turn, it can then be converted to the mineralocorticoid, aldosterone, and androstenedione, testosterone, estrone, and estradiol [21]. Please see the interrelationships among progesterone, androgens, and estrogens in women in Fig. 6.2.
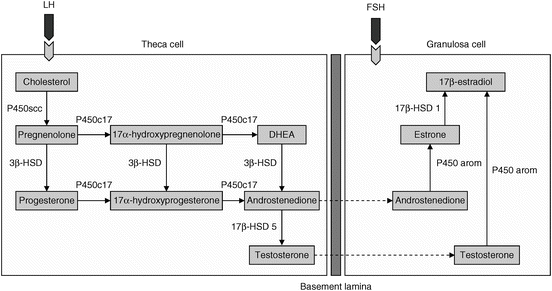
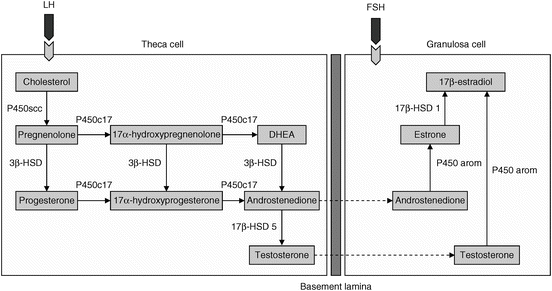
Fig. 6.2
The two-cell, two-gonadotropin hypothesis of ovarian stereoidogenesis regulation. Androgens synthesized by the theca cell diffuse into the avascular granulosa cell compartment. Following stimulation from follicle stimulating hormone (FSH), androstenedione and testosterone are aromatized to estrone and estradiol, respectively. DHEA dehydroepiandrosterone; HSD hydroxysteroid dehydrogenase; LH luteinizing hormone; P450scc cytochrome P450 (CYP) side-chain cleavage; P450arom CYP P450 aromatase (Reprinted from Enea C et al. Circulating androgens in women: exercise-induced changes. Sports Med. 2011;41(1):1–15, with permission from Springer Verlag)
While it has other small roles in human function, P4 is primarily important for proper menstrual cycle function, fertility, and pregnancy. It is particularly vital for the vascularization and maintenance of the endometrial lining during the luteal phase of the menstrual cycle and throughout pregnancy. In FHA, progesterone levels are reduced. Together with the inappropriately low levels of estradiol, these result in an absence of appropriate follicular development, ovulation, and luteal function [22]. As discussed above, luteal phase defects affect a large proportion of physically active women. Disorders of the luteal phase are characterized by poor endometrial maturation subsequent to inadequate P4 production and short luteal phases, and are associated with infertility and habitual spontaneous abortions [23].
Androgens
The adrenal glands and ovaries produce small amounts of testosterone, but more abundantly secrete weaker androgens. These include dehydroepiandrosterone (DHEA) and its sulfo-conjugate, DHEA sulfate (DHEAS) secreted by the adrenals, and androstenedione secreted by the adrenals and ovaries. These steroids can undergo peripheral conversion to more potent androgens, including testosterone and 5α-dihydrotestosterone (DHT), and can also be converted to estradiol [21].
Testosterone
In a study of women with anorexia nervosa, normal-weight women with FHA, and eumenorrheic controls, total and free testosterone were lower in women with anorexia nervosa than in controls, but subjects with FHA had normal androgen levels. Lower free and total testosterone predicted lower BMD at most skeletal sites measured and free testosterone was positively associated with fat-free mass. After controlling for BMI, total and free testosterone did not remain predictors of BMD [24]. In a small study of bone microarchitecture using flat panel CT in subjects with anorexia and healthy controls, total and free testosterone levels were positively associated with bone volume fraction (the volume of mineralized bone per unit volume of the sample) and trabecular thickness. These associations remained significant after controlling for BMI [25]. Because testosterone can be converted to estradiol, the relationship between testosterone and bone is thought to be mediated through estradiol. In fact, even in a study of male collegiate athletes, estradiol levels were more important determinants of BMD than testosterone [26].
Dehydroepiandrosterone
As mentioned above, DHEA is a steroid produced in the adrenal glands and is a precursor of testosterone, estradiol, and other steroids. Its secretion is largely regulated by ACTH, but angiotensins, gonadotropins, and prolactin may also regulate its production. DHEAS is the hydrophilic storage form that circulates in the blood, but it is converted to DHEA, the principle form used in steroid hormone synthesis. DHEAS concentrations are typically 250–500 times higher in the blood than DHEA. It is the most abundant steroid in circulation, but the extent of its physiological functions is unclear. While it is a clear precursor for various steroids, it has also been found to be a weak agonist and antagonist at testosterone receptors and a stronger agonist at estrogen receptors [27]. There have been contradictory findings in studies regarding DHEAS levels in individuals with anorexia nervosa. Some showed lower concentrations of DHEAS in those with anorexia versus healthy controls [28], higher levels [29], or no difference [24, 30]. In a preliminary study by Oskis et al., salivary DHEA levels were significantly higher in those with anorexia nervosa compared to controls [31]. Gordon et al. found a negative correlation between DHEAS and the bone resorption marker, urinary N-terminal telopeptide (NTX), in adolescent girls with anorexia nervosa [32]. In a recent double-blind, randomized, placebo-controlled trial by DiVasta et al., combined therapy with DHEA and low-dose estrogen/progestin also led to attenuation of bone loss and favorable changes in bone geometry in older adolescents and young women with amenorrhea in the setting of anorexia nervosa [33]. Use of micronized DHEA was safe, and in combination with low-dose estrogen/progestin had beneficial effects on bone health measures in young women with anorexia nervosa. More work is needed to understand the skeletal effects of adrenal steroids in this clinical setting.
Growth Hormone: Insulin-Like Growth Factor-I Axis
Growth hormone (GH), or somatotropin, is a polypeptide synthesized and secreted by the somatotrope cells of the anterior pituitary. Its production is under the control of hypothalamic growth hormone-releasing hormone (GHRH) and somatostatin, as well as ghrelin. GHRH promotes GH gene transcription and translation and increases GH release, while somatostatin has inhibitory effects on GH release. Ghrelin is a GH secretagogue. GH is necessary for overall body growth, including bone growth, but also influences metabolism of carbohydrates, proteins, and lipids. Low blood glucose, sleep, and exercise stimulate the production and release of GH. Alternatively, elevated blood levels of cortisol and glucose decrease its production and secretion. GH effects on growth and metabolism are mostly mediated by IGF-I, a peptide produced in the liver and other tissues in response to GH receptor activation by GH. IGF-I circulates bound to specific proteins, IGF-binding proteins (IGFBPs) and acid labile subunit, which regulate its bioavailability and bioactivity [34]. In addition, GH has direct effects on bone and fat. Both GH and IGF-I are bone anabolic.
In one study, mean serum GH and IGF-I correlated positively with fitness in adolescent girls, but 5 weeks of endurance training led to decreases in IGF-I and IGFBP-5 [35]. Whereas both endurance and resistance exercise have been shown to stimulate acute increases in GH and subsequently IGF-1 [36], decreases in IGF-I and IGFBP-5 over a longer time period in this study were thought to be partially explained by an energy deficit. Studies of anorexia nervosa patients have consistently shown an acquired resistance to GH, with decreased liver production of IGF-I despite elevated GH levels [37]. Low levels of GH-binding protein suggest decreased expression of the GH receptor, possibly accounting for the state of GH resistance during severely decreased energy availability [38]. In addition, IGF-I levels vary with the severity of under nutrition, and correlate positively with body mass index (BMI) and fat mass [39].
Laughlin and Yen [40] found a 70–80 % increase in 24 h mean GH levels in both amenorrheic and eumenorrheic athletes compared to sedentary controls, but with differences in pulsatile patterns. Although pulse amplitude was increased 60 % in eumenorrheic athletes with no change in pulse frequency, amenorrheic athletes demonstrated more frequent pulses and an elevated baseline between pulses. The pattern of GH pulses seen in amenorrheic athletes was associated with a 35 % decrease in GH-binding protein levels, which was not seen in eumenorrheic athletes. In this particular study, levels of IGF-I and IGFBP-3 did not differ in the eumenorrheic or amenorrheic athletes, but IGFBP-1 levels were two- to fourfold higher in the amenorrheic athletes versus the eumenorrheic athletes and controls. This resulted in a threefold reduced ratio of IGF-I/IGFBP-1 in amenorrheic athletes, which likely decreased the bioactivity and hypoglycemic effect of IGF-I [40]. In addition, consistent with other work, LH pulse frequency was related positively with insulin levels and the ratio of IGF-I/IGFBP-1, and negatively with IGFBP-1 concentrations. Overall, amenorrheic athletes displayed evidence of a hypometabolic state, including decreased basal body temperature and reduced levels of plasma glucose and serum GH-binding protein, a decrease in the IGF-I/IGFBP-1 ratio, accelerated GH pulse frequency, and elevated interpulse GH levels [40].
Few studies have focused specifically on GH pulsatility patterns in athletes with FHA. In one study of five amenorrheic and five eumenorrheic athletes, volunteers participated in two hospital admissions involving a 50 min submaximal exercise bout (70 % maximal oxygen consumption) and an 8 h nocturnal blood sampling period [41]. The amenorrheic athletes demonstrated an increased baseline serum GH level, increased number of nocturnal GH peaks, a prolonged half-life, and more brief GH secretory bursts of decreased mass. The amenorrheic athletes had more disorderly patterns of GH secretion, which paralleled elevated trough concentrations. Both disorderly and elevated trough GH release correlated with blunting of the GH secretory response to acute exercise, seen in the amenorrheic athletes. Concentrations of plasma IGF-I and its associated binding proteins were not significantly different between groups. These findings were different from those reported in patients with anorexia nervosa, who have decreased IGF-I levels, no difference in GH half-life, and increased pulse mass. Results were similar in that both anorexia nervosa subjects and these amenorrheic athletes had decreased regularity of GH pulses and increased pulse frequency [41]. Whether these differences in GH and IGF-I patterns are due to different mechanisms in amenorrheic athletes without eating disorders versus a more severe energy-restricted state, or are simply the result of a small study sample remains to be clarified. Certainly more studies are necessary to better understand GH and IGF-I secretory patterns in female athletes along the menstrual and energy availability spectrum.
Hypothalamic–Pituitary–Thyroid Axis
Thyroxine (T4) and 3,5,3′-triiodothyronine (T3) are tyrosine-based hormones produced by the thyroid gland as a result of thyroid-stimulating hormone (TSH) secretion by the pituitary. TSH secretion is regulated by T3 and T4 negative feedback, as well thyroid-releasing hormone (TRH) secretion from the hypothalamus. The thyroid hormones play critical roles in most bodily functions, including metabolism and bone mineral homeostasis, with T3 being the more metabolically active hormone compared to T4, a precursor of T3. For example, hyperthyroidism increases metabolic rate, decreases bone mineral density, and is associated with increased fracture risk. Hypothyroidism decreases metabolic rate, and while some studies have demonstrated increases in BMD in those with hypothyroidism, bone quality was poor and has been associated with increased fracture risk [42]. T3 is important for local IGF-I secretion in bone, which may account for poor bone quality in patients with hypothyroidism [43].
In a cross-sectional study of hypothalamic–pituitary–thyroid function in amenorrheic athletes versus eumenorrheic athletes and controls, Loucks et al. found lower serum levels of T4, T3, free T4, free T3, and reverse T3 (rT3) in amenorrheic athletes, and only lower serum T4 levels in eumenorrheic athletes [44]; there were no differences in thyroid-binding globulin or circadian rhythm of TSH secretion. TSH response to TRH stimulation was blunted in amenorrheic athletes versus eumenorrheic athletes, but not compared to eumenorrheic controls [44].
In a subsequent study of the effects of exercise and energy availability manipulations on thyroid function, 46 eumenorrheic, sedentary women were randomly assigned to low intensity exercise, high intensity exercise, or no exercise and low (8 kcal/kg body weight/day) or high energy availability (30 kcal/kg body weight/day) treatments [45]. After 4 days of the various exercise and energy availability regimens, those with low energy availability had 15 % lower T3, 18 % lower free T3, 7 % higher T4, and 24 % higher reverse T3. When energy availability was sufficient, exercise quantity (0 vs. 1,300 kcal/day) and intensity (40 vs. 70 % of aerobic capacity) did not affect the thyroid hormones. Hypothalamic sensitivity to the negative feedback of T3 remained intact, leading to higher levels of T4 in those subjects who were energy restricted. In the energy-deficient groups in this study, T3 levels fell despite sufficient T4 production (precursor of T3), suggesting decreased peripheral deiodinase activity [45]. In a follow-up study of sedentary women divided into groups based on energy availability (10.8, 19.0, 25.0, or 40.4 kcal/kg lean body mass/day) [46], decreases in T3 (16 %) and free T3 (9 %) occurred abruptly when energy availability decreased from 25 to 19 kcal/kg lean body mass/day and increases in free T4 (11 %) and reverse T3 (22 %) occurred abruptly when energy availability decreased from 19.0 to 10.8 kcal/kg fat-free mass/day. No significant changes in total T4 were found [46]. These findings were similar to those noted in anorexia nervosa, where T3 levels are low and reverse T3 levels are elevated [47]. In other studies, exercise and energy restriction have demonstrated increases, decreases, and no change in T4 levels [44–48]. Thus, a further understanding of the interplay of energy and exercise and the hypothalamic–pituitary–thyroid axis is needed.
Hypothalamic–Pituitary–Adrenal Axis
The hypothalamic–pituitary–adrenal (HPA) axis plays a critical role in a person’s ability to respond to both emotional and physiologic stress. The hypothalamus secretes corticotropin-releasing hormone (CRH) to trigger the secretion of adrenocorticotropic hormone (ACTH), which stimulates the release of cortisol, a glucocorticoid, from the zona fasciculata of the adrenal cortex. Cortisol has many roles, but primarily functions to alter carbohydrate, protein, and lipid metabolism, increase blood sugar via gluconeogenesis, and suppress the immune system. In addition, it inhibits bone formation, increases bone resorption, decreases calcium absorption, and enhances calcium excretion [49]. In the short-term, stress-induced activation of the HPA axis is adaptive, but chronic exposure of tissues to high cortisol levels secondary to long-term stress may be maladaptive, resulting in adverse effects particularly for bone [8].
Acute intense exercise at or above the anaerobic threshold as well as chronic endurance training have consistently demonstrated activation of the HPA axis and elevated cortisol levels. Studies in individuals exercising at a lower intensity have typically shown minimal if any cortisol response, except when prolonged activity leads to decreased glucose concentration [50]. For example, when subjects performed endurance exercise for 14 h at 50 % VO2 max, cortisol, ACTH, and CRH responses were unchanged as long as plasma glucose was maintained. The authors suggested a threshold plasma glucose concentration of ≥3.3 mmol/L (59.5 mg/dL) to prevent increases in cortisol [51]. Further work has also clarified HPA patterns with training and overtraining. Short-term intensified training increases both ACTH and cortisol; however, there may be some adaptation with training over time, leading to a reduced response of the HPA axis except when a substantial anaerobic component is present. Conversely, overreaching can lead to an increase in ACTH with a blunting of the adrenal cortisol response, with full-blown overtraining eventually leading to significant under activity of the entire HPA axis and the sympathoadrenal system [50].
Over 20 years ago, Kanaley et al. published a small study involving eumenorrheic athletes in various phases of their menstrual cycles (early follicular, late follicular, and mid-luteal) and amenorrheic athletes. All athletes perform 90 min of treadmill running at 60 % VO2 max, with blood sampling before and after exercise. Each athlete repeated the testing at different time points. The amenorrheic athletes had higher cortisol levels at rest and greater increases in cortisol in response to exercise than did the eumenorrheic athletes, whose basal and post-exercise cortisol levels were independent of menstrual phase [52]. In a different study of amenorrheic versus eumenorrheic runners, pre-exercise cortisol levels were elevated and cortisol responses to both maximal and submaximal exercise (40 min at 80 % maximal oxygen consumption) were blunted in amenorrheic runners [53]. Perhaps the decrease in cortisol response of amenorrheic runners to exercise in this study was related to overreaching. Further exercise testing studies are needed in amenorrheic athletes to better characterize their response to various levels of activity and training.
In patients with anorexia nervosa, cortisol levels are higher than in normal-weight controls, and are inversely associated with fat mass [54]. Higher cortisol concentrations have also been reported in nonathletic women with FHA versus eumenorrheic controls [16]. Our group specifically examined overnight cortisol secretory parameters in relation to LH pulsatility in 14–21 year old amenorrheic and eumenorrheic athletes and eumenorrheic nonathletic controls. Whereas BMI did not differ among groups, cortisol pulse amplitude, mass, half-life, and AUC were highest in the amenorrheic athletes and were inversely associated with fat mass. There were significant inverse associations between cortisol and LH AUC. Although cortisol concentrations were not associated with levels of bone turnover markers in amenorrheic athletes, cortisol correlated positively with the bone resorption marker, carboxy-terminal telopeptide (CTX), in eumenorrheic athletes, and inversely with the bone formation marker, procollagen type 1 amino-terminal propeptide (P1NP), in nonathletes [55].
Metabolic and Appetite-Regulating Hormones
Insulin
Insulin is a polypeptide synthesized in the pancreas in the β-cells of the islets of Langerhans. It stimulates uptake of glucose from the bloodstream into the liver, skeletal muscle, and adipose tissue, and contributes to cellular growth and hypertrophy. In addition, in vitro, clinical and animal data suggest an anabolic role for insulin in bone. When exposed to physiological doses of insulin, cultured osteoblasts show increased rates of proliferation, collagen synthesis, alkaline phosphatase production, and glucose uptake. Exactly how insulin signaling might promote osteoblastogenesis is yet to be elucidated [56].
While there is a well-characterized increase in glucose transport during exercise, there is also evidence that exercise training decreases insulin concentrations and increases insulin sensitivity [57]. However, when amenorrheic athletes, eumenorrheic athletes, and eumenorrheic nonathlete controls have been compared, hypoinsulinemia was more pronounced in the amenorrheic versus eumenorrheic athletes, extended throughout the day, and was accompanied by reduced glucose increments in response to meals, not observed in the eumenorrheic athletes [40]. When sedentary subjects with ovulatory cycles were compared with recreational runners with ovulatory cycles and those with luteal phase defects, insulin was lower in runners with luteal phase defects compared with the other two groups, similar to insulin decreases observed in amenorrheic athletes and other energy-deprived states [58].
Leptin
Leptin, a cytokine hormone produced by the obesity (ob) gene, is secreted primarily by adipocytes, and binds to leptin receptors, located throughout the body. Leptin is a key messenger of nutritional status and influences appetite, energy balance, and reproduction [59]. Leptin acts directly at the hypothalamus to decrease NPY mRNA and increase POMC mRNA in the arcuate nucleus. NPY is a potent stimulator of food intake, while alpha-melanocyte-stimulating hormone (α-MSH), a cleavage product of POMC, inhibits food intake. In general, leptin decreases when energy is restricted, leading to energy conservation and decreased thermogenesis [60]. Some of the complex interrelationships of leptin and other hormones are depicted in Fig. 6.3.
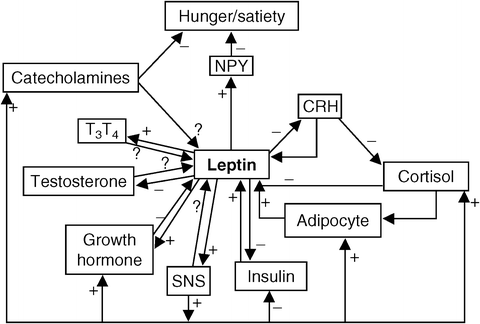
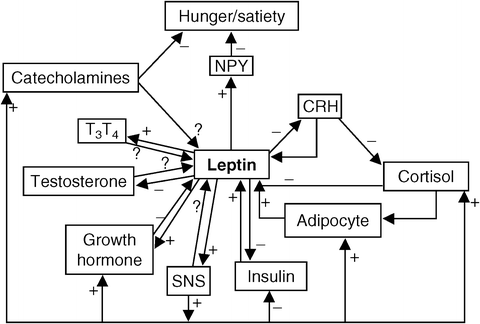
Fig. 6.3
The interrelationship between metabolic hormones, leptin, and hunger. CRH corticotropin-releasing hormone; NPY neuropeptide-Y; SNS sympathetic nervous system; T 3 thyroxine; T 4 triiodothyronine; – indicates pathway inhibits the activity of other homes; + indicates pathway enhances the activity of other hormones; ? indicates relationship has been proposes, but is not proven to exist (Reprinted from McMurray RG, Hackney AC. Interactions of metabolic hormones, adipose tissue and exercise. Sports Med. 2005;35(5):393–412, with permission from Springer Verlag)
Athletes have lower leptin levels than sedentary individuals, usually in the context of lower fat mass. However, independent effects of exercise training and increased energy expenditure on leptin, without changes in body fat content, have also been demonstrated [61–64]. It is now more widely held that increased exercise training needs to be accompanied by a state of energy deficit in order to affect leptin levels.
Desgorces et al. [65] found no changes in fasting leptin levels, body weight, percent body fat, or fat mass in rowers after an 8-month training season. Training volume increased during the 8 months, but so did energy intake, thus maintaining similar body composition. There was a positive correlation between leptin levels and energy availability 24 h after a controlled training session (90 min of ergometer rowing at 70–75 % VO2 max). After 24 h of recovery, leptin levels were lower when there was decreased energy availability [65]. This correlation between leptin level and energy availability was found regardless of the time during the 8-month training season that the athletes were tested. Further work suggests that leptin levels are only lowered after long-term exercise (≥60 min) or exercise causing energy expenditure ≥800 kcal [66].
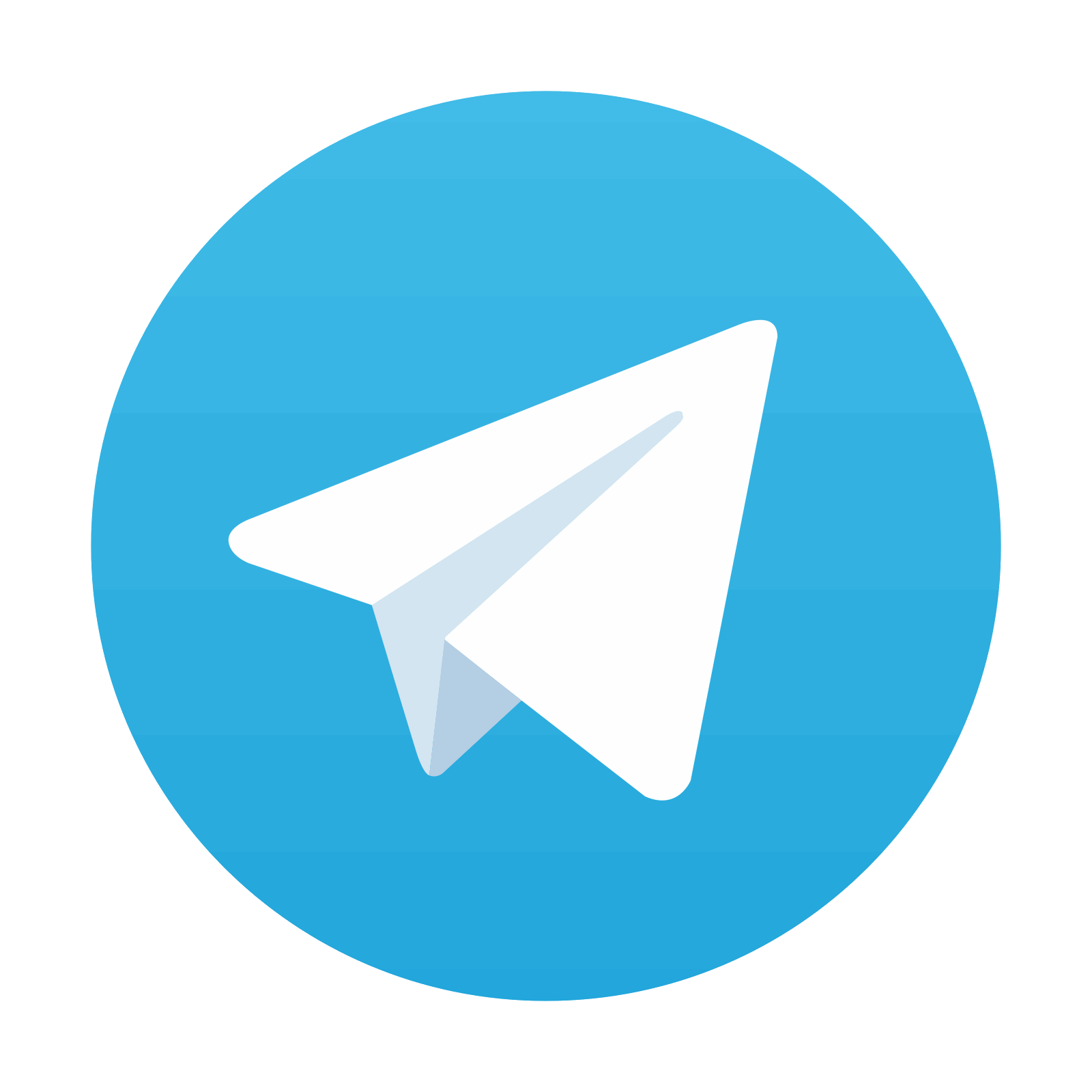
Stay updated, free articles. Join our Telegram channel

Full access? Get Clinical Tree
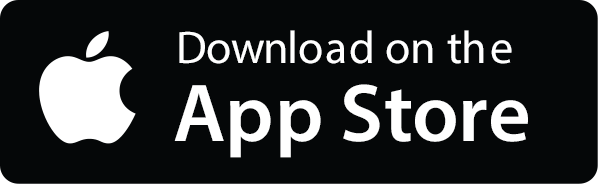
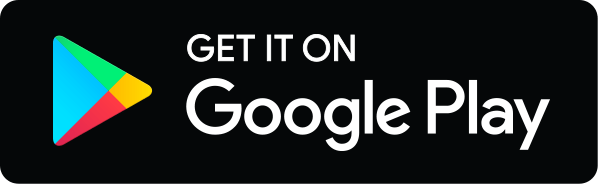