Fig. 6.1
(a) Axial image acquired at the level of the thigh with a T1-weighted (morphological) sequence showing a large haematoma due to complete ischiocrural muscle rupture. (b) Image acquired using a high-contrast sequence like STIR. The functional information and the hyperintense fluid component are very clearly depicted
T2-weighted sequences, which generate high-contrast images, afford functional assessment by clearly depicting inflammatory processes, fluid, or overtly liquid components in muscle (Fig. 6.1b).
An especially useful sequence in assessing muscle is the fat-suppression sequence, which removes the signal from adipose tissue, thus providing accurate information on lipomas.
6.2.1 Contrast-Enhanced MRI
Contrast agents are employed when very high definition is needed. They can contribute to differential diagnosis, particularly in the case of degenerative conditions, by differentiating among benign neoplasms or, more importantly, malignancies (Fig. 6.2a–b)


Fig. 6.2
(a) New, space-occupying, malignant formation involving the medial distal third of the thigh, where unenhanced T1-weighted sequences afford poor definition of the mass compared with adjacent muscle. (b) Same lesion as in A. Marked though inhomogeneous enhancement in the peripheral and central portion of the lesion obtained with a contrast-enhanced sequence
Paramagnetic contrast agents better tolerated than non-iodinated agents, especially where anaphylactic shock is concerned.
6.2.2 Magnetic Resonance Angiography
Magnetic resonance angiography (MRA) provides highly detailed images of the arterial and venous network. Its limited invasiveness is highly valuable to assess upper and lower limb muscle pathology.
6.2.3 Fusion Technology
This approach allows MRI scans to be superimposed on images acquired with other modalities such as US and CT.
Fusion technology is particularly valuable in the follow-up of malignancies and post-traumatic conditions, since it affords accurate monitoring of lesion resolution and progression (Fig. 6.3).


Fig. 6.3
Fusion technology allows overlaying MRI, CT, and US scans also when using colour and power Doppler or, in this case, elastography
6.2.4 Diffusion MRI
This approach detects the abnormal motion of water molecules, providing very clear images of trauma-induced muscle lesions, such as haemorrhage and haematoma, and critical information on signal changes related to metabolic muscle disorders.
The method provides two techniques: diffusion-weighted imaging (DWI) and diffusion tensor imaging (DTI).
With DWI, the more widely used, ADC maps enable a more clear visualization of tissue pathology (Fig. 6.4).


Fig. 6.4
DWI with ADC map provides high-definition images of muscle lesions based on the motion of hydrogen molecules
DTI (tractography) provides a detailed image of the whole course of structures such as peripheral nerves (Fig. 6.5).


Fig. 6.5
With DTI, the course of individual nerves can be extrapolated from surrounding structures using image subtraction sequences
6.2.5 MR Elastography
A further application of the MR technology, MR elastography, uses fast DWI sequences to depict muscle during contraction and relaxation.
6.2.6 Upright MRI
Even though upright MRI has been available for some years, manufacturers are still few.
The method has considerable added value in the examination of musculoskeletal conditions, since it affords accurate assessment of structures, especially in relation to patient posture, which clearly is mostly upright.
In particular, upright MRI allows resolving some differential diagnostic queries that cannot be decided on scans obtained with standard MRI.
6.2.7 Dynamic MRI
Our diagnostic imaging department has been the first to develop dynamic MRI at the international level, using the ESAOTE G-scan Brio magnet (Genova, Italy). The method is proving highly successful both in orthopaedic and neurosurgical applications.
The generation of dynamic scans while the patient is making movements in slow motion (internal and external rotation, flexion, and extension, to mention just a few) allows ideal assessment of the movement of each joint and muscle group, as dynamic US has been doing for some years. The panoramic views offered by MRI are a major advantage that enables very accurate diagnoses.
Our radiology technicians, Alessia Principe and Filippo De Carli, have been working to develop this important innovation with great professionalism and enthusiasm.
6.3 Muscles
6.3.1 Trauma-Related Conditions
Trauma-induced muscle injuries are among the most common musculoskeletal conditions.
Traumas can be divided into minor and major.
6.3.1.1 Minor Traumas
Injury to subcutaneous adipose tissue (crush injury) can be divided into lymphoedema, fat necrosis, and fascial shearing injury. The latter condition induces subcutaneous and suprafascial haematomas and is known as Morel-Lavallée syndrome (grade 1, 2, and 3, respectively) (Fig. 6.6).


Fig. 6.6
Grade 3 Morel-Lavallée lesion involving the subcutaneous fat adjacent to the tensor fasciae latae muscle of the right thigh; the underlying muscle is unaffected
MRI is indicated only in patients with extensive Morel-Lavallée syndrome, since US examination is easier to perform and sometimes conclusive.
Muscle injuries include contracture, elongation, and contusion.
Contractures and elongations are induced by an indirect mechanism—they thus lack tears of intrinsic muscle fibres and are characterized by oedema.
Contusions induce muscle changes due to direct trauma; the main injury often involves the deep muscle plane, where the propagation of the force vector is halted by bone.
In patients with contractures and elongations, MRI can depict changes in signal intensity related to oedema formation. Its characteristic high signal intensity and inhomogeneous distribution are easier to see using high-contrast sequences.
A typical minor muscle injury, delayed-onset muscle soreness (DOMS), is due to perifascial oedema. Again, high-contrast sequences, especially STIR, are the most suitable (Fig. 6.7).


Fig. 6.7
Clear involvement of myofascial components in a patient with DOMS
6.3.1.2 Major Traumas
Major muscle traumas are divided by their severity into partial and complete rupture.
Injury grading and the definition of distraction should be reserved to clinical settings, whereas the MRI report should describe the lesion in terms of extent, compartment, and extra-compartmental extension, as appropriate.
Partial ruptures are often fairly difficult to diagnose if they involve minor injury. MRI is eminently suitable to monitor haematoma evolution. Haematomas are found in partial as well as complete lesions, because they are related to fibre tearing.
Haematomas form in the first few hours after the trauma, in the hyperacute phase, which lasts ca. 12 h, and show inhomogeneous signal intensity due to the erythrocyte fraction (oxyhaemoglobin phase).
The acute phase, marked by erythrocyte lysis, usually takes place from 24 to 48 after the injury (deoxyhaemoglobin phase).
The subacute stage—from ca.48 to 72 h post-trauma—is related to complete colliquation of the haematoma (methaemoglobin phase) (Fig. 6.8).


Fig. 6.8
Subacute phase of the lesion, assessed about 72 h from the trauma (methaemoglobin phase). Complete colliquation of the haematoma allows appreciating its actual extent also in view of its evacuation
Finally, the last stage (chronic phase) involves organization of the haematoma, if it is not treated, due to haemosiderin degradation processes.
In this phase, the haematoma may undergo calcification, which in the most severe cases may evolve to metaplastic ossification.
The main advantages of MRI over US in this setting are related to site, extension, signal, course, and morphology.
Site: muscle lesions may be found in anatomical areas that are difficult to assess by US, in particular the pelvic muscles such as the internal and external obturators, the iliopsoas, and the quadratus femoris, which are not clearly visualized by US.
Extension: it relates to the multiplanar ability and, especially, the panoramic views offered by MRI.
Signal: MRI accurately depicts the changes related to the trophism of muscle components, particularly fatty replacement of muscle fibres, which may be due to trauma or, more often, neuromuscular disease (Fig. 6.9).


Fig. 6.9
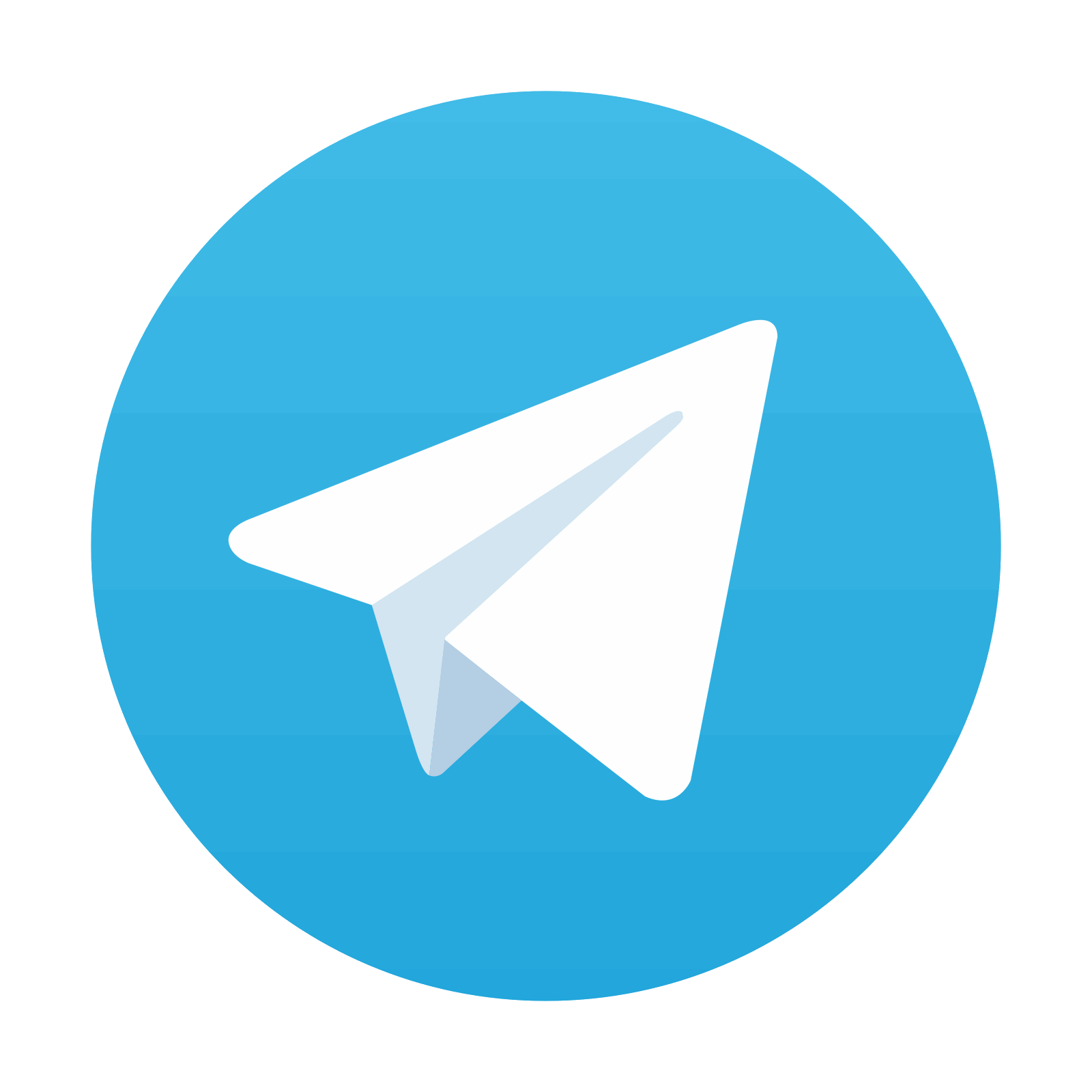
MRI provides optimal definition of the interface among different tissues, in particular muscle and fat, as in this giant cell tumour, whose fibroadipose tissue component is clearly outlined against adjacent muscle
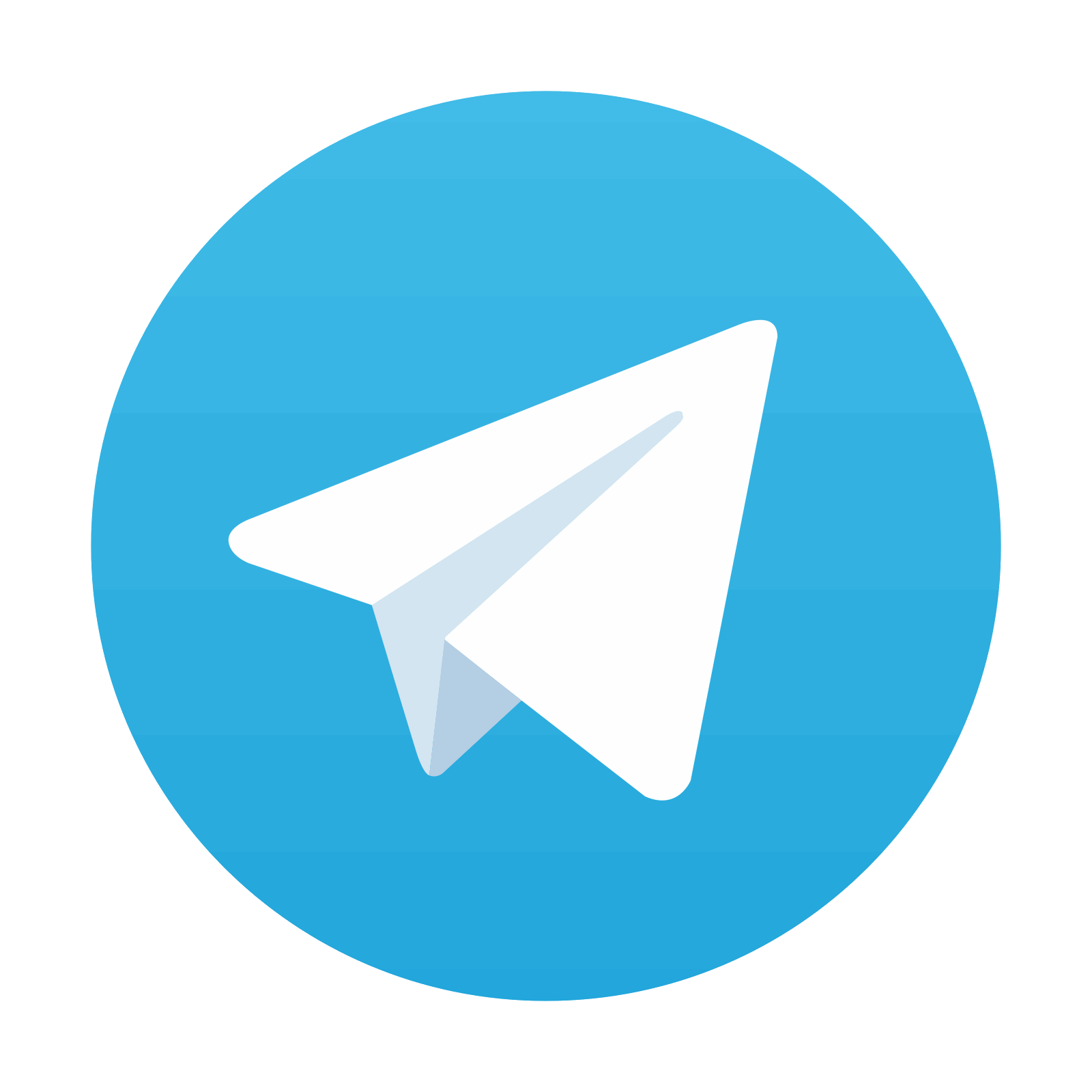
Stay updated, free articles. Join our Telegram channel

Full access? Get Clinical Tree
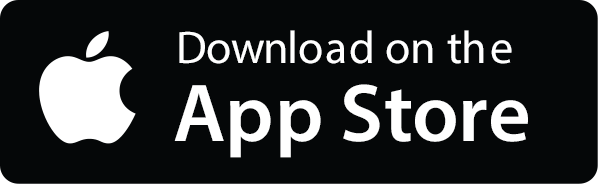
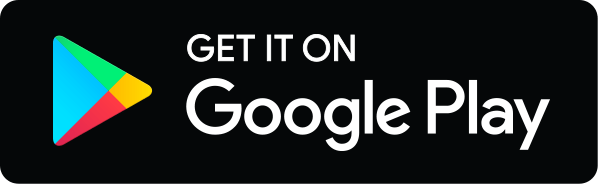
