© ISAKOS 2017
Alberto Gobbi, João Espregueira-Mendes, John G. Lane and Mustafa Karahan (eds.)Bio-orthopaedics10.1007/978-3-662-54181-4_2929. Meniscus Scaffolds: 30 Years of Experience
(1)
Center for Regenerative Sports Medicine, Steadman Philippon Research Institute, 181 West Meadow Drive, Suite 1000, Vail, CO 81657, USA
(2)
Collagen Matrix, Inc., Oakland, NJ, USA
29.1 Introduction
For many years, the meniscus was a forgotten structure, or perhaps worse, it was maligned as a useless evolutionary vestige. During that period, it was commonplace and the preferred procedure to perform a complete meniscectomy for almost any meniscus lesion. In recent decades, it has been shown unequivocally that the menisci are critical structures and play a major role in the overall health of the knee. We now know that the menisci protect the underlying articular cartilage through their load bearing, load and stress distribution, and shock absorption functions. Furthermore, the menisci also enhance joint stability by increasing joint congruency and provide lubrication that aids in joint motion. As is well documented, one of the key difficulties in repairing the meniscus is that the tissue does not have the ability to repair itself in the avascular (or the white) zone once it is injured [1]. Thus, partial meniscectomy is still the most common modality in treating meniscus tears. The effect of losing meniscus on the load distribution and the subsequent development of osteoarthritis of the knee is well established [2, 3].
Having this understanding of the importance of the menisci has led to many efforts to preserve the meniscus through repair, reconstruction, or replacement. The advanced development of knee arthroscopy has aided greatly these meniscus preserving procedures. Additionally, the advent and refinement of magnetic resonance imaging (MRI) has exponentially increased our diagnostic capabilities to identify lesions of the meniscus much more accurately and sooner so that many meniscus lesions now better lend themselves to repair. Earlier treatment of meniscus lesions also allows better protection and preservation of the underlying articular cartilage.
One of the earliest efforts to reconstruct or replace the meniscus involved the use of allograft tissue. About 30 years ago, Milachowski et al. [4] reported their experience with the first free meniscus allograft transplantations in human patients. They reported on 23 patients who received either lyophilized or deep frozen meniscus allografts. Since that time, many new allograft preservation techniques and surgical implantation procedures have been developed. However, use of allograft tissue raises concerns regarding cost, adequate supply, and availability, as well as the possibility of disease transmission, albeit rare. Also, meniscus allograft transplantation is typically indicated only for total or near-total replacement of the damaged or missing meniscus. Meniscus allograft tissue has not been used routinely for partial or segmental meniscus reconstruction or replacement.
Because of these and other concerns, the idea of developing a meniscus scaffold, either permanent or resorbable, or a regeneration template was conceived. The idea generally was to use the scaffolds for partial or segmental replacement of lost or damaged tissue, not to use these scaffolds for total replacement of the entire meniscus.
The aims of meniscus replacement are: (1) to reduce the pain experienced by many patients after even partial meniscus resection; (2) to prevent the degenerative changes of articular cartilage and the changes in subchondral bone frequently observed after meniscectomy; (3) to avoid or reduce the risk of osteoarthritis after meniscus resection; and (4) to restore the optimal mechanical properties of the joint after meniscus resection. If these aims are accomplished, the knee can function more normally and the patient can return to the desired level of activity. The meniscus replacement or repair or newly regenerated tissue that has been stimulated to grow after loss of meniscus tissue does not necessarily need to recapitulate the normal meniscus exactly. It must, however, function enough like meniscus tissue that it permits the patients to regain the levels of activity to which they aspire.
29.1.1 The Past
As arthroscopic surgical techniques and instrumentation evolved and improved, many meniscus lesions were found to be treatable with partial meniscectomy. While fortunately total meniscus removal became far less common, surgeons were still faced with the dilemma that partial loss of the meniscus led to altered joint mechanics and often to degenerative osteoarthritis. It was also observed that the speed and degree of chondral degeneration was directly related to the amount of meniscus tissue removed. Consequently, our group and others in the later part of the 1980s set out to develop a meniscus scaffold or regeneration template to use to treat partial or segmental meniscus loss.
The possibility of using synthetic artificial materials to replace the meniscus cartilage is attractive because of the availability of the materials and the ability to manipulate these materials in their form and composition. Teflon, polyester, and carbon fibers were used most frequently by investigators in early attempts to replace the meniscus with synthetic materials. Toyonaga et al. [5] used a Teflon-net prosthesis to replace the lateral meniscus in dogs following total meniscectomy. The TefIon-net device was rolled and folded to mimic the shape of the lateral meniscus, and then it was sutured to the joint capsule. The authors reported slowly progressive degenerative changes and osteoarthrosis of the tibial articular cartilage and similar but less severe degenerative changes of the femoral articular cartilage over the 12 months of the study. The Teflon-net prosthetic meniscus was forced laterally from the joint by the femoral condyle, and without the protective mechanism of a suitable meniscus substitute, the classical degenerative changes observed following total meniscectomy were also noted in this study. Following extrusion of the prosthetic device from the joint, there were significant adhesions between the Teflon-net material and the popliteal muscle that were severe enough to impede normal knee joint motion. The authors also reported the presence of chronic inflammatory cells, fibroblasts, and foreign body giant cells around the Teflon-net fibers as early as 3 months after the initial implantation. A similar histologic appearance was still present at 12 months. Nonetheless, these authors concluded that the Teflon-net prosthesis might be ideal for meniscus replacement because of its pliability, softness, and histocompatibility; its potential ability to protect the joint surfaces; and its ability to support migration and infiltration of cells into its interstices. In spite of their positive comments about this artificial material, nothing further was published regarding its use.
A poly(l-lactide)-reinforced carbon fiber material was used by Leenslag et al. [6] as a porous composite both for replacement and for reconstruction of the meniscus. This prosthetic device lacked the ability to prevent degenerative arthritis of the knee joint, and early instability of the implanted joints was observed. As this prosthetic device degraded, free carbon fiber particles were released into the joints and produced a significant synovitis, which complicated the interpretation of the results. A group composed of some of these same investigators [7] next looked at experimental meniscus lesions reconstructed with a similar carbon fiber-poly(l-lactide) material but with segmented polyurethane included in the formulation. They produced large wedge-shaped lesions in the menisci of dogs, then reconstructed the menisci with their carbon fiber-polyurethane-poly(l-lactide) graft material. The prostheses were removed at 4 or 8 weeks after implantation. The authors observed that the implant was almost completely invaded by fibrous tissue that, in some cases, appeared similar to hyaline cartilage. They also reported that the problem of the carbon fiber-induced synovitis was still present and needed to be resolved prior to additional investigations with this material.
Because of the carbon fiber synovitis, Klompmaker [8] and Klompmaker et al. [9] omitted the carbon fibers in a prosthetic device that he designed, which was composed of polyurethane and poly(l-lactide) that was further reinforced with poly(l-lactide) fibers. He also designed another device consisting of polyurethane only. Again, the experimental model was the menisci of large dogs. Klompmaker [8] and Klompmaker et al. [9] found that about half of the implants composed of polyurethane and poly(l-lactide) supported fibrous tissue ingrowth and healing of the meniscus lesions. The majority of the nonhealing implants resulted from being dislocated out of the joint. When the implants of polyurethane only were used, 10 of 15 showed acceptable healing. Klompmaker [8] and Klompmaker et al. [9] concluded that these implants guided the ingrowth of the vascular repair tissue into the defect within the meniscus and that the fibrous tissue eventually transformed into fibrocartilage.
Wood et al. [10] developed a polyester-carbon fiber bioprosthesis composed of concentrically stacked hoops of carbon fibers and sheathed by woven, high-tenacity polyester fibers. The polyester fibers were extended from either end of the prosthetic device and used for anchorage through drill holes in the tibia. The device was used to replace the medial meniscus of 18 rabbits, which were then evaluated at 3 or 6 months. The device had been displaced from its location in most of the knees at the time of final evaluation. There was also a high incidence of carbon fiber-induced synovitis similar to that reported by other authors. These authors also reported that, in nearly all cases, the polyester fibers appeared to produce a marked inflammatory response with no significant ingrowth of cells into the carbon fibers and essentially no biologic regeneration of a morphologically acceptable meniscus in any of the experimental knees. Consequently, the normal functional properties of the meniscus were not supported or provided by the prosthetic device.
A patent was issued to Wall [11] in 1985 for an artificial meniscus made of “pliable” materials such as rubber or Teflon. The patent also indicated that the pliable materials would be reinforced with nylon or stainless steel. We are not aware of any published studies related to use of this patented invention.
Sommerlath and Gillquist [12] evaluated a meniscus prosthesis made of dacron with a polyurethane coating. They replaced the medial menisci of rabbits with this prosthesis and then conducted gross and histologic evaluation 3 months after surgery. They compared their results to sham-operated knees, knees with only a peripheral incision of the medial meniscus, and knees with a total medial meniscectomy. They observed that the knees with the dacron-polyurethane prosthesis had the same frequency of cartilage changes on the femur as did knees with total meniscectomy, but the prosthesis-implanted knees had fewer cartilage changes on the tibial surface. They also observed that there was a high rate of osteophyte formation in both the prosthetic device-implanted knees and the total meniscectomy knees, but the osteophytes were significantly larger following implantation of the prosthetic device. Nearly all of the joints implanted with the prosthesis developed synovitis, but the authors stated that no loose dacron particles were found, and there was no apparent foreign body reaction. Overall, the dacron-polyurethane prosthesis knees showed the same negative findings as did the knees with total meniscectomies. Knees with only the peripheral meniscus incision were similar to the sham-operated knees, and both of these groups were near normal.
Because of the shortcomings of the aforementioned devices, our group decided a better approach would be to use a naturally occurring protein that would be completely resorbed over time. Our working hypothesis for generation and growth of new meniscus tissue was that the native meniscus has the intrinsic ability to regenerate if the biological environment is suitable for regeneration. Thus, for any tissue-engineered product to function in a load-bearing application, one must first be certain that the design requirements for that product are met. Among the three components of a tissue-engineered product for growing new meniscus tissue, we considered the extracellular matrix to be the most critical element of the three since the extracellular matrix serves the biomechanical function of the meniscus [13, 14]. Hence, the initial biomechanical properties of the extracellular matrix and the subsequent biomechanical properties of the regenerated and remodeled tissue must be adequate for the device to function as intended. Furthermore, the extracellular matrix must be cytoconductive for cells growing into the scaffold as well as permeable to nutrients. The biological signal and the cells, the other two components of a tissue-engineered product, can accelerate the overall regeneration and remodeling process and may provide a more ideal biological environment for cellular infiltration and matrix synthesis [13, 14].
29.1.2 The Present
The field of regenerative medicine has made noteworthy progress during the past three decades, particularly in designing and engineering resorbable scaffolds made of natural or synthetic polymers for guiding the various types of tissue regeneration, including the meniscus [15–35]. Among the materials chosen for design and engineering the scaffolds, Type I collagen has been the most pursued biomaterial for these applications due to its availability in large quantities, established methods of isolation and characterization, and biocompatibility properties for human implantation. A variety of Type I collagen-based scaffold implants have been marketed in recent years to treat various human lesions including peripheral nerves [36], orthopedic and sports injuries [23, 26–28, 31, 35, 37], dermal injuries [38], and others [39–42]. Among these various types of tissue repair and regeneration, meniscus has been one of the most challenging areas and is the subject of this review and discussion.
In the 1990s, the present authors with others developed a Type I collagen-based meniscus implant for meniscus reconstruction and regeneration [23, 27, 30]. Animal and clinical studies demonstrated the utility of this implant after partial meniscus removal or previous meniscus loss with success, i.e., the collagen scaffold supported the new meniscus tissue regeneration and improved knee function [26, 28, 35, 43, 44]. Long-term clinical follow-ups have been published with respect to the efficacy of the repair with the collagen meniscus implant [26, 29, 35, 43–45]. The product has been marketed outside the United States in much of the world since 2001 and was recently cleared by the US Food and Drug Administration for sale and clinical use in the United States.
The other meniscus scaffold that is available for clinical use in Europe is an acellular polyurethane scaffold that is resorbed slowly over a period of several years [21, 32, 33]. This scaffold has high flexibility and high suture pullout strength. Verdonk et al. [33] observed that at 1-year relook nearly all of the implanted scaffolds showed integration with the native meniscus and all biopsy specimens showed fully vital material with no signs of cell death or necrosis. At 2 years, there was continued improvement in the same group of patients, but there was a failure rate of about 17% [32]. The authors concluded that at 2 years after implantation, safety, and clinical outcome data from their study supported use of the polyurethane scaffold for the treatment of irreparable, painful, partial meniscus defects. Kon et al. [21] reported their 2-year results with this polyurethane scaffold in 18 patients. They observed no adverse events and clinical improvement over the 2 years in their cohort of patients. They concluded that the polyurethane scaffold was safe and potentially an effective procedure to treat partial meniscus loss with encouraging results at short-term follow-up [21]. Because the polyurethane scaffold has been available for less time than the collagen meniscus implant, there are fewer published articles on the polyurethane scaffold [46].
29.1.3 The Future
Despite the initial success of this Type I collagen meniscus implant, which was the first regenerative product on the market for human use, the authors believe that there are areas for further improvement, particularly with respect to the biomechanical function during the initial period of meniscus healing. To that end, improving the biomechanical properties and the healing rate, shortening the rehabilitation time, and rapidly returning the knee to its full function are still the goals to accomplish. Consequently, future generations of implants must be developed.
Tissue engineering is a special branch of science that combines life science, engineering, and clinical science to develop biological substitutes to improve, restore, and maintain the targeted tissue and organ function. Tissue engineering generally encompasses three key components, namely, the extracellular matrix (ECM) scaffold, cells, and biological signaling factors. Each of these components will be discussed in the following sections with respect to the development of a next generation implant for meniscus reconstruction and regeneration.
We believe that a tissue engineering approach can provide the best solution to regenerative medicine, particularly for tissues and organs such as the meniscus that are difficult to repair once they are damaged from sports or disease. Below we discuss the strategy to develop a new generation of tissue-engineered implants for meniscus repair and regeneration.
Our initial design of the Type I collagen-based meniscus implant scaffold was based on purified Type I collagen fibers isolated from bovine Achilles tendon [23, 24]. These fibers satisfied many design parameters, including dimension which limits the boundary of tissue regeneration, pore structure for cell ingrowth, initial mechanical strength for implantation, and biocompatibility. Due to the stringent biomechanical requirements of the meniscus, the post-surgery rehabilitation program had to be strictly followed to permit the anticipated biological activities to occur, including cell infiltration from the vascular zone, new tissue deposition and maturation, etc. In this respect, the rate of implant resorption, the rate of new tissue deposition and subsequent tissue remodeling and maturation had to be balanced properly and maintained such that the return of knee function could be achieved.
Since the collagen meniscus implant was introduced for clinical use in 2001, extensive work has been done on the feasibility of development of tissue engineering products for meniscus repair and regeneration [15, 17–20, 32–34, 47–53]. While other materials are described, our focus is on using Type I collagen as a material for the construction of ECM scaffolds and incorporation of scaffolds with cells and biological factors. Since a Type I collagen-based scaffold for meniscus repair has provided us with invaluable information for the advancement of meniscus repair and regeneration, we believe it is prudent and essential to take this scaffold to the next level of development, i.e., developing a new tissue-engineered collagen meniscus implant. We believe that the general discussion below applies to the scaffolds developed from other materials as well.
We propose approaching the tissue engineering technology from two different concepts. The first concept is to incorporate cells and biological factors into the scaffold at the time of surgical implantation of the scaffold. The other concept will be to develop a tissue-engineered product in a cell culture system/tissue reactor wherein cells are infiltrated into a scaffold. The cells then would grow and produce new extracellular matrix prior to implantation. If stem cells are used in tissue engineering, then the cells should differentiate into the phenotype of the specific tissue to be repaired in the in vitro system by using specific biological and mechanical factors as stimulants for cell differentiation [54–56, 87].
There are advantages of each concept. With respect to the former, the implant can be developed at a faster rate in terms of the translational process including various issues of manufacturing and regulatory requirements. It is a common observation that to develop a tissue engineering product for tissue repair (cells, growth factors, scaffolds, or any combination), it will take 15–20 years, depending on the type of tissues to be repaired. It is important to understand that about one million meniscus surgeries are performed each year, most of which are partial meniscectomies [57]. In future years, a great number of these patients likely will develop osteoarthritis if some type of meniscus regeneration or replacement is not employed. The goal of developing a treatment strategy must take that into consideration. The second advantage of the first concept is that the surgical procedure is straightforward with minimal additional training of today’s skilled arthroscopists.
The second concept is still at the early research stage. Many questions related to the cell process, including cell growth within the scaffold, culture conditions, phenotype identification and implant characterization, manufacturing reproducibility, etc. must be addressed and resolved. The initial regeneration of the meniscus will have been partly completed at the in vitro cell culture stage prior to implantation; therefore, the subsequent healing process in vivo including tissue remodeling and maturation will be more predictable.
However, we believe that one can develop a tissue-engineered meniscus implant based on the first concept within a more definitive and financially feasible time frame. As the science and technology advance in the field of tissue engineering, the second concept will emerge later, and the more complicated issues can be addressed and hopefully resolved at a faster rate.
The goal, therefore, will be to expand the scope of the initial ECM concept to the tissue engineering approach. Thus, the objectives of developing a next generation meniscus implant are the following: (1) Improve the initial biomechanical stability of the scaffold; (2) Increase the rate of healing and regeneration of the reconstructed meniscus; (3) Reduce the rehabilitation time post-surgery; and (4) Accelerate the return of function.
The above objectives are all interrelated. The first objective is directly related to the biomechanical properties of the scaffold. Due to the lack of control of certain biological parameters and human factors including age, health and knee conditions, gender, and compliance of the patients with respect to the post-surgery rehabilitation activities, the initial strategy will be focused on the scaffold design. The original Type I collagen scaffold using the reconstituted collagen fibers developed in the 1990s satisfied the initial biomechanical requirements including suturability to the adjacent meniscus rim, permitting passive movement since the size and shape of the implant mimicked the size and shape of the human meniscus, allowing a certain degree of compression and shear stress, and permitting cell ingrowth and vascularization for nutritional supply. Even with the satisfaction of these key design requirements, there was still some degree of re-tear of the repaired meniscus tissue during the important period of meniscus healing and regeneration [26, 35, 45].
To achieve and enhance the above goals, the design strategy should be focused on designing a collagen scaffold that will be more durable during the first few months of meniscus healing. Firstly, the collagen fibers should be aligned in a manner that mimics the native meniscus, along the stress direction of the fibers. One advantage of the fiber reconstitution technology is that the orientation of the fibers can be designed according to the biomechanical requirements. Secondly, the lubricious properties of the surface of the implant can be improved. An improved lubricious surface can reduce the shear-related stress and possible tearing in patients particularly with poor quality articular cartilage and thus facilitate the load distribution when a large load is placed upon the implant. This improvement can be accomplished by incorporating lubricious hydrogel molecules into the implant. Effective lubricious molecules include many polyanionic or polycationic molecules. Polyanionic molecules include hyaluronic acid, chondroitin sulfates, and the like. Polycationic molecules include chitosan, polylysine, and the like. These molecules can be incorporated into the collagen scaffold implant by co-precipitation during the engineering process of the scaffold implant [23]. It is important that the molecules are physically, mechanically, and electrostatically entrapped within the collagen fibers without being chemically linked to collagen via covalent bonding. The entrapped molecules will slowly diffuse out from the implant causing the surface to be lubricious to minimize the friction between the femoral condyle and the implant surface. The rate of release of the molecules can be controlled by the molecular weight of the molecules. The rate of molecular incorporation and release can also be controlled by chemical modification of the electrostatic interaction properties of the collagen fibers towards polyanionic or polycationic molecules [58].
The rate of healing can be enhanced by adding cells to the scaffold. Either meniscus fibrochondrocytes or mesenchymal stem cells can be used. The use of autologous cells may be preferred based on the time required to reach the clinical usage of the implant. Meniscus chondrocytes can be obtained via a biopsy from the red zone of the meniscus (vascular rim) as the tissue can regenerate in the vascular zone of the meniscus. The cells can be isolated, cultured, and characterized according to standard procedures [59, 60]. It is feasible to inject the cells into the porous collagen scaffold implant at the time of scaffold implantation.
Autologous stem cells can be isolated from several different tissues [61]. The common tissue used for isolating mesenchymal progenitor stromal cells is the bone marrow [62]. The isolation and culture of these cells is well known in the literature. Another source of stem cells can be isolated from fat tissues [63]. Recently, emphasis has been placed on skeletal muscle-derived stem cells for many applications [64, 65]. All mesenchymal stem cells share the common characteristics in that they are multipotent cells capable of differentiating into chondrocytes given the proper biological environment [61]. For example, at the meniscus site the stem cells will differentiate into chondrocytes and produce ECM that will have the structure to withstand the loads similar to the native meniscus [66].
Allogeneic stem cells isolated from umbilical vein tissue holds some promise for clinical application [67]. The isolation and culture of these cells have been published in past years [68–70]. The advantage of these cells is that many cells can be isolated from a single cord. With limited numbers of passages (generally from two to three), several million stem cells with multipotent cell phenotypes can be obtained for clinical use [69].
Regarding the incorporation of biological factors into the scaffold as described above, it is recognized that there are many bioactive molecules that have been evaluated in vitro in cell culture systems. Some of these molecules had a significant positive effect on the key parameters including proliferation, ECM deposition, cell migration, and scaffold contraction. At present, there are only two key growth factors on the market, BMP2 and PDGF. Both growth factors have been tested on meniscus cells and showed positive effects [71–73]. BMP2 is a potent osteogenic factor and has been shown to be chondrogenic [71]. PDGF has been tested for a variety tissue repair studies including meniscus and soft tissue wound healing [72, 73].
The strategy would be to obtain these biological factors from the autologous source or from the human recombinant source that have been approved for clinical use for accelerated entry of the implant for clinical application. In this area, a large amount of information has been accumulated regarding the sources of autologous tissue growth factors that can be applied to enhance the meniscus repair and regeneration. One autologous source to obtain the biological factors is bone marrow. Various studies have shown that bone marrow contains mesenchymal stem cells and numerous bioactive molecules including PDGF and BMP2 that can be isolated and used for tissue engineering applications. It has been shown recently that platelet-rich plasma (PRP) isolated from blood has a positive effect on the healing of ACL repair when a collagen-based scaffold is used to retain the PRP at the wound site for prolonged activity in situ [74]. In this respect, it might be beneficial to use the collagen meniscus scaffold as a carrier of PRP for the meniscus repair and regeneration. The PRP can be incorporated into the scaffold either post scaffold implantation via injection or pre-saturated with PRP prior to implantation.
29.2 Specifics of Tissue Engineering
The following section provides specific details of each of the components of tissue engineering as they apply to the future development of the next generation of an enhanced collagen meniscus implant. To reiterate, the three essential components are the extracellular matrix (ECM), the cells, and the tissue-specific signaling factors.
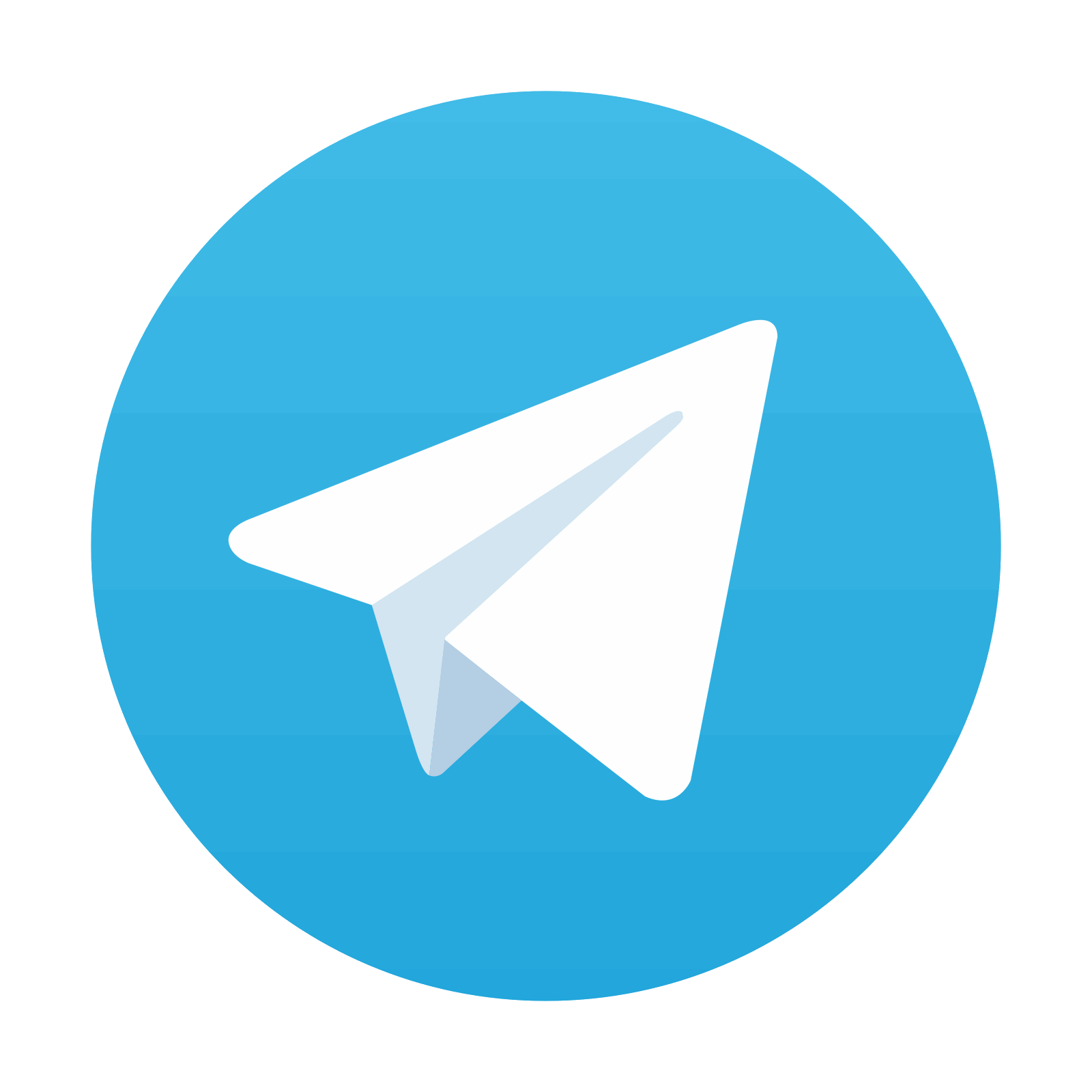
Stay updated, free articles. Join our Telegram channel

Full access? Get Clinical Tree
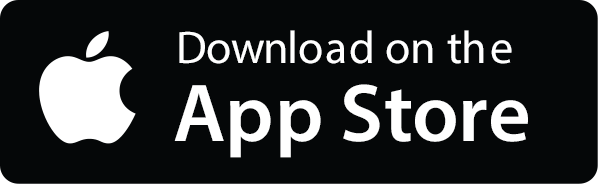
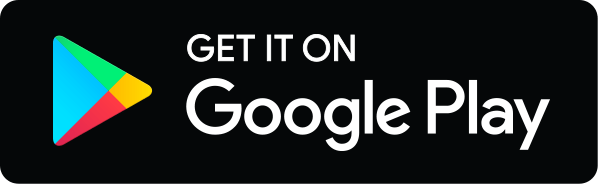