Restrictive lung disease occurs commonly in patients with neuromuscular disease. The earliest sign of respiratory compromise in the patient with neuromuscular disease is nocturnal hypoventilation, which progresses over time to include daytime hypoventilation and eventually the need for full-time mechanical ventilation. Pulmonary function testing should be done during regular follow-up visits to identify the need for assistive respiratory equipment and initiate early noninvasive ventilation. Initiation of noninvasive ventilation can improve quality of life and prolong survival in patients with neuromuscular disease.
- •
Restrictive lung disease occurs commonly in patients with neuromuscular disease.
- •
The earliest sign of respiratory compromise in the patient with neuromuscular disease is nocturnal hypoventilation, which progresses over time to include daytime hypoventilation and eventually the need for full-time mechanical ventilation.
- •
Pulmonary function testing should be done during regular follow-up visits to identify the need for assistive respiratory equipment and initiate early noninvasive ventilation.
- •
Initiation of noninvasive ventilation can improve quality of life and prolong survival in patients with neuromuscular disease.
Introduction
Normal breathing depends on the intact function of the ventilator pump, which consists of the central respiratory control centers, the bony rib cage, and the muscles of breathing. In progressive neuromuscular diseases (NMDs), the ventilator pump is often impaired and leads to a predictable pattern of respiratory compromise beginning with normal or near normal unassisted gas exchange early in the disease, adequate daytime gas exchange with nocturnal hypoventilation during mid-stage disease, and chronic and/or acute respiratory failure requiring full-time assisted ventilatory support for survival in late-stage disease. In addition, normal defense of the lung depends on adequate secretion management and the precise timing of and force generated by the activities of the ventilator pump to produce an effective cough. Pneumonia, respiratory failure, and, ultimately, death can all occur as a consequence of ventilator pump dysfunction. For many NMDs, breathing disorders are recognized as the leading cause of mortality. However, appropriate screening with timely intervention and use of assistive respiratory devices can prevent complications and prolong life in those in whom NMD compromises their respiratory system.
Cause of respiratory failure in NMD
Normal Breathing
Breathing is an active, primarily involuntary process requiring work. Inspiration occurs as the diaphragm and external intercostal muscles contract and expand the thorax, causing the intrapulmonary pressure to decrease and pull air into the lungs. Air enters the upper airway against resistance and travels via bulk flow, like a water faucet, through to the terminal bronchioles. As the cross-sectional area of the lung dramatically increases in the respiratory zone, the alveolated region of the lung, the forward velocity of air dramatically slows and diffusion becomes the chief mode of ventilation. After air exchange, quiet exhalation occurs passively, propelled by energy stored during inspiration from the elastic properties of the tissues of the rib cage and lung. The recoil of the rib cage and lung tissues increases intrapulmonary pressure, reverses airflow, and expels CO 2 abundant air from the lungs. During stressed breathing, the elastic recoil is not sufficient to cause rapid expiration and the abdominal and internal intercostals participate.
The work of breathing depends on both the elastic and viscous forces of the lung. Under normal conditions, the work of breathing is quite small and requires less than 5% of total resting O 2 consumption. In restrictive lung physiology caused by NMD, however, the work of breathing increases. Respiratory failure results from several factors that either increase or are influenced by the increased work of breathing, including (1) respiratory muscle weakness and fatigue, (2) alteration in respiratory system mechanics, and (3) impairment of the central control of respiration.
Respiratory Muscle Weakness and Fatigue
Respiratory muscle weakness and fatigue are frequent contributors to ventilator failure in the patient with NMD. Respiratory muscle weakness, the inability of the respiratory muscles to generate normal levels of pressure and flow during inspiration or expiration, occurs because of lack of appropriate neural stimulation of muscle fibers, as in spinal cord injury and amyotrophic lateral sclerosis (ALS), or intrinsic muscle disease, as in the muscular dystrophies. Weakness of the muscles of inspiration (the diaphragm, intercostals, and accessory muscles) results in inadequate lung expansion, with subsequent microatelectasis, leading to ventilation/perfusion mismatch and consequent hypoxemia. Compensatory tachypnea, with small tidal volumes, exacerbates the atelectasis and further reduces the compliance of the respiratory system, increasing the mechanical load on already weakened respiratory muscles. Progressive muscle weakness and fatigue lead to restrictive lung disease with hypoventilation, hypercarbia, and respiratory failure. As opposed to the chronic progressive changes in pulmonary function caused by respiratory muscle weakness, respiratory muscle fatigue causing ventilator failure usually occurs after impairment of respiratory muscle strength has been reduced to 30% of predicted values.
Measures of respiratory muscle strength
There is continued investigation into the best measures of respiratory muscle strength for the patient with NMD and how these measures offer prognostic information and predict onset of nocturnal hypoxemia and respiratory failure. Historically, the maximal inspiratory pressure (MIP) and maximal expiratory pressure (MEP) have been used as measures of respiratory strength. Several studies have identified the sniff nasal inspiratory pressure (SNIP) as a consistent measure with good prognostic value in patients with NMDs. In ALS, the SNIP showed greater consistency across disease severity compared with forced vital capacity (FVC), MIP, or MEP in patients with severe bulbar dysfunction. Studies have demonstrated a linear decline in SNIP as ALS progresses and a strong correlation between low SNIP measure and nocturnal hypoxemia. In a study of 98 patients with ALS, an SNIP of less than 40 cm H 2 O had a sensitivity of 97% and a specificity of 79% for death within 6 months.
Spirometry with FVC measurement has been commonly used as a measure of respiratory strength and function. Supine positioning eliminates the affects of gravity and may be a better marker of diaphragmatic strength, and in ALS, supine FVC is the best predictor of survival. Spirometry alone is not sufficient to predict early physiologic respiratory failure with nocturnal hypoventilation. In the setting of Duchenne muscular dystrophy (DMD), FVC values have no ability to predict nocturnal elevation in end-tidal CO 2 .
What has become clear is that there is no single screening test that can accurately predict the development of nocturnal hypoventilation or survival. Multiple modalities testing has been shown to be a more effective strategy. In DMD, a forced expiratory volume in 1 second of less than 40% predicted, a Pa co 2 45 mm Hg or greater, and a base excess greater than 4 mmol/L are factors that indicate the development of sleep-disordered breathing. In ALS, the use of 5 tests at each visit allowed for early initiation of noninvasive ventilation (NIV) and was associated with improved survival. The panel of tests included upright and supine FVC, upright and supine MIP, and overnight oximetry.
When using lung function testing to assess cough and need for airway clearance, the mentioned testing strategy has not been as effective. Peak cough flow testing using a peak flowmeter instead of a spirometer can accurately predict the need to initiate airway clearance (<270 L/min) or the potential need for tracheotomy (<160 L/min).
Alteration in Respiratory Mechanics
In addition to effects on muscle contraction, NMD increases elastic and resistive loads on the respiratory muscles. Both types of loads increase the work of breathing and hasten ventilator failure. Increases in elastic loads are a consequence of abnormal stiffness of both the lungs and the chest wall. Finally, kyphoscoliosis, which is frequently associated with NMD, results in deformation of the thoracic cage, altering the biomechanics of the respiratory muscles and compromising their ability to operate effectively against the increased elastic and resistive loads, further increasing the work of breathing. Thoracolumbar scoliosis is almost universal in boys, with DMD with the most profound effect on ventilation occurring in those in whom the curves have an early onset. Vital capacity may be disproportionately reduced in this case, but even in adolescents with severe scoliosis, the impact on vital capacity is correlated with the magnitude of the curve.
Impairment of Control of Ventilation
Disorders of central control of respiration frequently are associated with NMD processes. Defects in control of respiration may be caused by central factors as in bulbar poliomyelitis or ALS. Central factors associated with control of ventilation during sleep impact respiratory function even in primarily muscular disorders. The first indicator of disordered respiratory control typically occurs in association with sleep. The supine sleep position increases work of breathing by increasing resistance from the chest and abdomen. Slow-wave sleep reduces both tidal volume and respiratory rate, whereas stage rapid eye movement further stresses the system by causing atonia in accessory muscles. In a system already stressed, these changes can drive the development of hypoventilation with hypoxemia and CO 2 retention.
Symptoms and signs of nocturnal hypoventilation include air hunger, snoring, choking, orthopnea, cyanosis, restlessness, insomnia, daytime hypersomnolence, morning headaches, drowsiness, fatigue, depression, and impaired cognition. Significant nocturnal decreases in partial pressure of oxygen (Pa o 2 ) and elevations in arterial partial pressure of carbon dioxide (Pa co 2 ) have been reported. These changes most commonly begin to occur during rapid eye movement sleep when a pattern of rapid shallow breathing develops as a result of supraspinal inhibition of the alpha motor neuron drive, maximizing hypotonia to prevent motor activity during dreams. Nocturnal hypoventilation sometimes is associated with apneic episodes, leading to additional hypercapnia. Hypercapnia or hypoxemia occurring at night may have a role in reducing daytime central respiratory drive by depressing central drive centers directly and by increasing the “bicarbonate pool.” This latter effect blunts the stimulus to increase respiratory rate generated by respiratory acidosis and perpetuates the hypercapnic state. In addition, as the work of breathing increases, patients with restrictive lung disease reach a threshold at which increasing Pa co 2 will no longer drive the homeostatic response to increase respiratory rate and CO 2 accumulates.
Presentation of Respiratory Failure and Variable Practice Patterns Regarding Management
Respiratory failure typically present in 1 of 3 ways in patients with NMD: (1) as acute respiratory failure such as that occurring in high-level spinal cord injury, Guillain-Barre syndrome, and tick paralysis; (2) as an acute respiratory decompensation in a chronic disease such as ALS or myasthenia gravis; or (3) as a chronic worsening of a gradually progressive disease such as DMD.
Treatment of acute respiratory failure frequently involves endotracheal intubation and positive pressure ventilation (PPV) in the intensive care unit. Often tracheostomy tube placement is required. In this situation, unfortunately, there are few choices of methods of ventilation. Under certain circumstances, it is possible to wean a patient from invasive PPV by using a variety of noninvasive ventilator techniques. Thus, the tracheostomy tube, which some patients find objectionable, is not necessarily permanent.
The more common presentation, with insidious onset of respiratory failure in patients with NMD, can be improved by early intervention to prevent respiratory complications and prolong life. Noninvasive forms of both positive and negative ventilation and the rocking bed have been used effectively in reversing, at least temporarily, progressive chronic respiratory failure. Initially, patients may require ventilator support for only part of the day. In these cases, nocturnal ventilatory support has been shown to be of great value. Daytime ventilation either full-time or for prescribed periods can be used as muscular weakness progresses. Thus, monitoring a patient’s pulmonary function becomes of paramount importance in directing treatment. A survey of ALS clinic directors published in 1999 was a focused query to determine common pulmonary practices. This study found that 85% of ALS clinics performed pulmonary function tests (PFTs) every 3 months during clinic visits and decisions to initiate NIV were based on PFT results. Despite this, a later study revealed that only 15.9% of patients with ALS were using therapy with NIV.
Development of Consensus Guidelines for Pulmonary Management of NMDs
Several consensus practice parameters have been developed to outline disease-specific pulmonary monitoring and management recommendations.
ALS
A consensus statement, based on a systematic review of the literature, was recently published with recommendations for pulmonary management in ALS. The American Academy of Neurology (AAN) consensus document acknowledges that management of the patient with ALS is best accomplished with multispecialty care teams. Respiratory care providers play a key role in these teams. Their focus includes 3 areas: (1) lung function assessment, (2) prevention of chest infections, and (3) ventilatory support.
Lung function assessment is used to help in the timing of percutaneous endoscopic gastrostomy tube (PEG) placement, initiation of NIV, and considerations for end-of-life care. Nutritional support has been found to be beneficial in improving survival and quality of life for both patients and care-givers. The placement of PEG tubes includes risk resulting from anesthetic and procedural complications such as aspiration, pneumonia, and ventilator failure. The lower the lung function, the greater is the risk of the procedure. The AAN recommendations suggest PEG placement at an FVC greater than 50% and placement should be performed with great caution if the FVC is less than 30%.
When using lung function testing to assess need to initiate NIV, the AAN guidelines discuss the use of testing with an MIP greater than –60, SNP less than 40, or FVC less than 50% as the time to initiate therapy ( Fig. 1 ). The guidelines from Centers for Medicare and Medicaid Services have additional suggestions for initiation of NIV including oxygen saturation of 88% or less for longer than 5 minutes or a Pa co 2 less than 45. Last, the AAN guidelines suggest the use of lung function testing to assess survival. Survival of less than 3 months is noted with SNIP of less than 30 cm H 2 O. Daytime saturation less than 95% or nocturnal saturation less than 93% had similar findings.
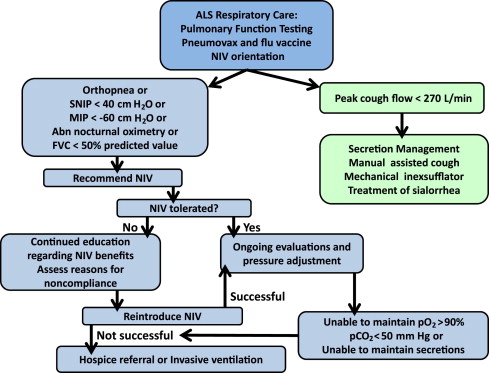
The AAN recommends a proactive approach to prevent chest infections as these represent a high source of morbidity and mortality in the ALS patient group. The AAN recommends performing vaccination with both pneumovax and influenza vaccination. Airway clearance techniques should be initiated when the peak cough flow testing is less than 270 L/min. Artificial cough support is most strongly recommended using a mechanical inexsufflation (MIE) device ( Fig. 2 ). These devices support both the inhale and exhale components of cough with delivery of positive pressure followed by negative pressure through either a mask or mouthpiece. Although there are limited data, the use of high-frequency chest wall oscillation may be beneficial. These devices, wrapped around the torso, provide vibratory force through the chest wall to the lung to help keep mucus mobile, preventing atalectasis ( Fig. 3 ). Nebulized medications are frequently used in conjunction with these techniques but there are no data to support their use.
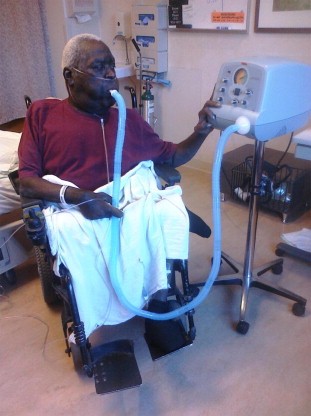
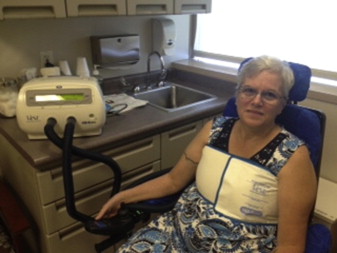
Ventilation is strongly supported. NIV is recommended to both improve disease outcomes and improve the quality of life for both patients and care-givers. There are no specific recommendations as to the type of NIV device or settings to be used. It should be noted, however, that the recommendations from the Centers for Medicare and Medicaid Services suggest the use of an NIV device with a back-up rate instead of a spontaneous breathing device. Tracheostomy with mechanical ventilation (TIV) is suggested in the AAN guidelines, as it has been shown to have equal quality of life and acceptance by patients compaired to NIV. Those with cognitive impairment, bulbar disease, or lowest lung function are more likely to require TIV.
DMD
In 2004, the American Thoracic Society published guidelines for respiratory care in DMD. Their recommendations were as follows: Each child with confirmed DMD should undergo an evaluation of respiratory status early (between ages 4 and 6), and tests of respiratory function should be performed at every clinic visit thereafter. Regular cardiac evaluations should start at school age and patients should be seen by a pulmonologist twice a year beginning at age 12 or when their FVC deteriorates to 80% of normal. Care by a pulmonologist should be increased to every 3 to 6 months after the initiation of assisted ventilation or an airway clearance device. Cough effectiveness should be evaluated regularly, and patients should be instructed on the use of a manual cough assist maneuver or an assisted coughing device, as well as taught how to use an oximeter at home to measure blood oxygen levels. Last, these recommendations addressed the importance of maintaining good nutrition with use of a gastrostomy tube if necessary.
In 2010, the Centers for Disease Control and Prevention (CDC) sponsored the development of consensus care considerations for DMD using experts in multiple disciplines including pediatric and adult pulmonary medicine. A RAND methodology was used.
The aim of respiratory care is to allow timely prevention and management of complications. The experts concluded that a structured, proactive approach to respiratory management that includes use of assisted cough and nocturnal ventilation has been shown to prolong survival. Patients with DMD are at risk of respiratory complications as their condition deteriorates as a result of progressive loss of respiratory muscle strength, including ineffective cough, nocturnal hypoventilation, sleep disordered breathing, and, ultimately, daytime respiratory failure.
The care team must include a physician and therapist with skill in the initiation and management of NIV and associated interfaces, lung-volume recruitment techniques, and manual and mechanically assisted cough.
Assessments and interventions will need to be reevaluated as the patient’s condition changes ( Tables 1–3 ). In the ambulatory stage, minimum assessment of pulmonary function (eg, measurement of FVC at least annually) allows familiarity with the equipment and the team can assess the maximum respiratory function achieved. The main need for pulmonary care is in the period after the loss of independent ambulation. A respiratory action plan should be enacted with increasing disease severity.
DMD Patient Status | Recommended Clinical Assessments | Assessment Frequency |
---|---|---|
Ages ≥6 y: Ambulatory | Sitting FVC | No less than annual evaluation |
Non-ambulatory |
| No less than every 6 mo |
| Capnography measuring: awake et CO 2 | No less than annual evaluations and with each respiratory infection in a patient with FVC <50% predicted |
DMD Patient Status | Strongly Recommended Assessment |
---|---|
| Pulse oximetry |
| Pulse oximetry |
| Measure gas exchange during sleep c |
DMD patient status | Assessment to be strongly considered |
| Measure gas exchange during sleep a |
a All specified threshold values of peak cough flow and MEP apply to older teenage and adult patients.
b Signs/symptoms of hypoventilation include fatigue, dyspnea, morning or continuous headaches, sleep dysfunction (frequent nocturnal awakenings [>3], difficult arousal), hypersomnolence, awakenings with dyspnea and tachycardia, difficulty with concentration, frequent nightmares.
c Dual-channel oximetry-capnography in the home is strongly recommended, but other recommended methods include home oximetry during sleep and polysomnography, the method of choice being determined by local availability, expertise, and clinician preference.
Step 1: volume recruitment/deep lung inflation technique | Volume recruitment/deep lung inflation technique (by self-inflating manual ventilation bag or mechanical insufflation–exsufflation) when FVC <40% predicted |
Step 2: manual and mechanically assisted cough techniques |
|
Step 3: nocturnal ventilation |
|
Step 4: daytime ventilation |
|
Step 5: tracheostomy |
|
a All specified threshold values of peak cough flow and MEP apply to older teenage and adult patients.
b Recommended for nocturnal use: NIV with pressure-cycled bilevel devices or volume-cycled ventilators or combination volume-pressure ventilators. In bilevel or pressure support modes of ventilation, add a back-up rate of breathing. Recommended interfaces include a nasal mask or a nasal pillow. Other interfaces can be used and each has its own potential benefits.
c Recommended for day use: NIV with portable volume-cycled or volume-pressure ventilators; bilevel devices are an alternative. A mouthpiece interface is strongly recommended during day use of portable volume-cycled or volume-pressure ventilators, but other ventilator-interface combinations can be used depending on clinician preference and patient comfort.
d However, the panel advocates the long-term use of NIV up to and including 24 h/d in eligible patients.
Although the expert panel recognized that assisted ventilation via tracheostomy can prolong survival, the care considerations advocate strongly for the use of noninvasive modes of assisted ventilation. Particular attention to respiratory status is required around the time of planned surgery.
Immunization with 23-valent pneumococcal polysaccharide vaccine is indicated for patients aged 2 years and older. Annual immunization with trivalent inactivated influenza vaccine is indicated for patients 6 months of age and older. Neither the pneumococcal vaccine nor the influenza vaccine is a live vaccine, and thus they can be administered to patients treated with glucocorticoids, but the immune response to vaccination might be diminished. Up-to-date and detailed information on immunization indications, contraindications, and schedules can be obtained from various sources, including the American Academy of Pediatrics and the CDC.
During an established infection, in addition to the use of manually and mechanically assisted cough, antibiotics are necessary, regardless of oxygen saturation if positive evidence of an infection is established on culture and regardless of culture results if pulse oximetry remains at less than 95% in room air. Supplemental oxygen therapy should be used with caution because oxygen therapy can apparently improve hypoxemia while masking the underlying cause, such as atelectasis or hypoventilation. Oxygen therapy might impair central respiratory drive and exacerbate hypercapnia. If a patient has hypoxemia as a result of hypoventilation, retained respiratory secretions, and/or atelectasis, then manual and mechanically assisted cough and NIV support are necessary. Substitution of these methods by oxygen therapy is dangerous.
Spinal muscular atrophy
Similar to patients with other neuromuscuolar disorders, children with spinal muscular atrophy (SMA) are at high risk of pulmonary related morbidity and mortality. Notably, children with SMA have not only diaphragm weakness but also a more prominent issue with a greater involvement of expiratory and intercostal muscles. In SMA type 1 and type 2, this causes a bell-shaped chest and sternal deformity. In SMA type 3, kyphoscoliosis adds to the chest wall restriction issues.
Lung function assessment has not been well studied in SMA. Recommendations have been derived by expert opinion only. There is a trend to adjust the type and frequency of lung function assessments by age and phenotype. Of those with SMA type 1, patients (nonsitters) are young and therefore unable to participate in standard lung function testing. High respiratory rates and paradoxic breathing are important physical examination findings. Pulse oximetry less than 94% supports a high likelihood of hypoventilation. Although many other tools are available and can be used, there are no formal recommendations for the frequency of use of transcutaneous CO 2 , overnight oximetry, full polysomnography, chest radiography, or swallow evaluation. SMA type 2 patients (sitters) are similar to SMA type 1 patients except lung function testing becomes possible as the child ages and symptom assessment is easier. Symptoms should be used to drive assessment choices. SMA type 3 (walkers) patients have relatively late onset of respiratory failure – even into adulthood, but regular assessment with spirometry and polysomnography is recommended. The intervals for testing are not fixed and should be driven by symptom assessment.
Respiratory interventions should be driven by the integration of a pulmonary physician in to the formal SMA team. This professional will need to coordinate care for hospitalization, for instance, around a surgery or pneumonia. In addition, pulmonary support should ensure that preventative care is performed. such as immunizations.
Airway clearance on a routine basis is important as age appropriate and could include the use of the high-frequency chest wall oscillation, MIE, or nebulized medication. The family should be guided in an anticipatory manner to set very clear clinical goals with the pulmonary team member, addressing options for noninvasive, or invasive mechanical ventilation as well as alternatives for hospice care.
Congenital muscular dystrophies
Congenital myopathies are a large group of disorders that include many different genetic abnormalities and phenotypes. These disorders vary from other NMDs because muscle weakness alone is not responsible for respiratory impairment. Central nervous system lesions and cognitive dysfunction contribute to abnormalities in control of ventilation, swallowing, and cough. The presence of mutations including abnormalities in laminin, collagen, etc, can be present, causing scoliosis and/or spinal rigidity. The involvement of these structural elements can allow for respiratory failure to occur as a result of loss of spine and core rigidity, before the development of global weakness. As a group, these patients develop early-onset respiratory failure and require aggressive airway clearance and ventilator support. There are no trials that address these techniques directly in the setting of congenital myopathies. Expert consensus has recommended :
- •
Perform full spirometry with every visit.
- •
Obtain one overnight oximetry examination if the patient shows an abnormal physical examination with increased work of breathing such as tachypnea or retractions. The patient may have signs of sleep disruption such as restlessness during sleep or decreased functioning during the day. Last, obtain overnight oximetry examination if there are recurrent chest infections, poor weight gain, morning headache, or FVC less than 60% or if a greater than 20% difference is observed between sitting and supine FVC if sitting FVC is less than 80%.
- •
Obtain one-night CO 2 monitoring if oximetry has a low baseline of less than 94% on room air when patient is awake or asleep and/or oximetry drops to less than 90% on room air for longer than 5 minutes with a low of at least 85% or greater than 30% of total sleep time spent at less than 90% saturation.
- •
Obtain a blood gas analysis if there is an acute onset of respiratory distress, if noninvasive CO 2 monitoring is not available, or to correlate with et CO 2 and transcutaneous measurements obtained.
- •
Obtain one-night full polysomnogram (with end-tidal CO 2 ) for suspected gas exchange abnormalities caused by hypoventilation. Hypoventilation is present if greater than 25% of the total sleep time is spent with CO 2 greater than 50 mm Hg; also, polysomnography may be helpful to titrate best settings for NIV.
Respiratory considerations for patients with NMD undergoing general anesthesia and surgery
The CDC DMD Care Considerations Working group published specific respiratory management issues for patients undergoing general anesthesia and surgery. Respiratory interventions are intended to provide adequate respiratory support during induction of, maintenance of, and recovery from procedural sedation or general anesthesia. In particular, they are designed to reduce the risk of postprocedure endotracheal extubation failure, postoperative atelectasis, and pneumonia. These goals can be achieved by providing noninvasively assisted ventilation and assisted cough after surgery for patients with significant respiratory-muscle weakness, as indicated by subthreshold preoperative PFT results.
Preoperative training in and postoperative use of NIV is strongly recommended for patients with a baseline FVC of less than 50% predicted and necessary with an FVC of less than 30% predicted. Incentive spirometry is not indicated owing to potential lack of efficacy in patients with respiratory muscle weakness and the availability of preferred alternatives, such as mechanical insufflation–exsufflation. After careful consideration of the risks and benefits, patients with significant respiratory-muscle weakness might be eligible for surgery, albeit with increased risk, if these patients are highly skilled preoperatively in the use of NIV and assisted cough.
Cause of respiratory failure in NMD
Normal Breathing
Breathing is an active, primarily involuntary process requiring work. Inspiration occurs as the diaphragm and external intercostal muscles contract and expand the thorax, causing the intrapulmonary pressure to decrease and pull air into the lungs. Air enters the upper airway against resistance and travels via bulk flow, like a water faucet, through to the terminal bronchioles. As the cross-sectional area of the lung dramatically increases in the respiratory zone, the alveolated region of the lung, the forward velocity of air dramatically slows and diffusion becomes the chief mode of ventilation. After air exchange, quiet exhalation occurs passively, propelled by energy stored during inspiration from the elastic properties of the tissues of the rib cage and lung. The recoil of the rib cage and lung tissues increases intrapulmonary pressure, reverses airflow, and expels CO 2 abundant air from the lungs. During stressed breathing, the elastic recoil is not sufficient to cause rapid expiration and the abdominal and internal intercostals participate.
The work of breathing depends on both the elastic and viscous forces of the lung. Under normal conditions, the work of breathing is quite small and requires less than 5% of total resting O 2 consumption. In restrictive lung physiology caused by NMD, however, the work of breathing increases. Respiratory failure results from several factors that either increase or are influenced by the increased work of breathing, including (1) respiratory muscle weakness and fatigue, (2) alteration in respiratory system mechanics, and (3) impairment of the central control of respiration.
Respiratory Muscle Weakness and Fatigue
Respiratory muscle weakness and fatigue are frequent contributors to ventilator failure in the patient with NMD. Respiratory muscle weakness, the inability of the respiratory muscles to generate normal levels of pressure and flow during inspiration or expiration, occurs because of lack of appropriate neural stimulation of muscle fibers, as in spinal cord injury and amyotrophic lateral sclerosis (ALS), or intrinsic muscle disease, as in the muscular dystrophies. Weakness of the muscles of inspiration (the diaphragm, intercostals, and accessory muscles) results in inadequate lung expansion, with subsequent microatelectasis, leading to ventilation/perfusion mismatch and consequent hypoxemia. Compensatory tachypnea, with small tidal volumes, exacerbates the atelectasis and further reduces the compliance of the respiratory system, increasing the mechanical load on already weakened respiratory muscles. Progressive muscle weakness and fatigue lead to restrictive lung disease with hypoventilation, hypercarbia, and respiratory failure. As opposed to the chronic progressive changes in pulmonary function caused by respiratory muscle weakness, respiratory muscle fatigue causing ventilator failure usually occurs after impairment of respiratory muscle strength has been reduced to 30% of predicted values.
Measures of respiratory muscle strength
There is continued investigation into the best measures of respiratory muscle strength for the patient with NMD and how these measures offer prognostic information and predict onset of nocturnal hypoxemia and respiratory failure. Historically, the maximal inspiratory pressure (MIP) and maximal expiratory pressure (MEP) have been used as measures of respiratory strength. Several studies have identified the sniff nasal inspiratory pressure (SNIP) as a consistent measure with good prognostic value in patients with NMDs. In ALS, the SNIP showed greater consistency across disease severity compared with forced vital capacity (FVC), MIP, or MEP in patients with severe bulbar dysfunction. Studies have demonstrated a linear decline in SNIP as ALS progresses and a strong correlation between low SNIP measure and nocturnal hypoxemia. In a study of 98 patients with ALS, an SNIP of less than 40 cm H 2 O had a sensitivity of 97% and a specificity of 79% for death within 6 months.
Spirometry with FVC measurement has been commonly used as a measure of respiratory strength and function. Supine positioning eliminates the affects of gravity and may be a better marker of diaphragmatic strength, and in ALS, supine FVC is the best predictor of survival. Spirometry alone is not sufficient to predict early physiologic respiratory failure with nocturnal hypoventilation. In the setting of Duchenne muscular dystrophy (DMD), FVC values have no ability to predict nocturnal elevation in end-tidal CO 2 .
What has become clear is that there is no single screening test that can accurately predict the development of nocturnal hypoventilation or survival. Multiple modalities testing has been shown to be a more effective strategy. In DMD, a forced expiratory volume in 1 second of less than 40% predicted, a Pa co 2 45 mm Hg or greater, and a base excess greater than 4 mmol/L are factors that indicate the development of sleep-disordered breathing. In ALS, the use of 5 tests at each visit allowed for early initiation of noninvasive ventilation (NIV) and was associated with improved survival. The panel of tests included upright and supine FVC, upright and supine MIP, and overnight oximetry.
When using lung function testing to assess cough and need for airway clearance, the mentioned testing strategy has not been as effective. Peak cough flow testing using a peak flowmeter instead of a spirometer can accurately predict the need to initiate airway clearance (<270 L/min) or the potential need for tracheotomy (<160 L/min).
Alteration in Respiratory Mechanics
In addition to effects on muscle contraction, NMD increases elastic and resistive loads on the respiratory muscles. Both types of loads increase the work of breathing and hasten ventilator failure. Increases in elastic loads are a consequence of abnormal stiffness of both the lungs and the chest wall. Finally, kyphoscoliosis, which is frequently associated with NMD, results in deformation of the thoracic cage, altering the biomechanics of the respiratory muscles and compromising their ability to operate effectively against the increased elastic and resistive loads, further increasing the work of breathing. Thoracolumbar scoliosis is almost universal in boys, with DMD with the most profound effect on ventilation occurring in those in whom the curves have an early onset. Vital capacity may be disproportionately reduced in this case, but even in adolescents with severe scoliosis, the impact on vital capacity is correlated with the magnitude of the curve.
Impairment of Control of Ventilation
Disorders of central control of respiration frequently are associated with NMD processes. Defects in control of respiration may be caused by central factors as in bulbar poliomyelitis or ALS. Central factors associated with control of ventilation during sleep impact respiratory function even in primarily muscular disorders. The first indicator of disordered respiratory control typically occurs in association with sleep. The supine sleep position increases work of breathing by increasing resistance from the chest and abdomen. Slow-wave sleep reduces both tidal volume and respiratory rate, whereas stage rapid eye movement further stresses the system by causing atonia in accessory muscles. In a system already stressed, these changes can drive the development of hypoventilation with hypoxemia and CO 2 retention.
Symptoms and signs of nocturnal hypoventilation include air hunger, snoring, choking, orthopnea, cyanosis, restlessness, insomnia, daytime hypersomnolence, morning headaches, drowsiness, fatigue, depression, and impaired cognition. Significant nocturnal decreases in partial pressure of oxygen (Pa o 2 ) and elevations in arterial partial pressure of carbon dioxide (Pa co 2 ) have been reported. These changes most commonly begin to occur during rapid eye movement sleep when a pattern of rapid shallow breathing develops as a result of supraspinal inhibition of the alpha motor neuron drive, maximizing hypotonia to prevent motor activity during dreams. Nocturnal hypoventilation sometimes is associated with apneic episodes, leading to additional hypercapnia. Hypercapnia or hypoxemia occurring at night may have a role in reducing daytime central respiratory drive by depressing central drive centers directly and by increasing the “bicarbonate pool.” This latter effect blunts the stimulus to increase respiratory rate generated by respiratory acidosis and perpetuates the hypercapnic state. In addition, as the work of breathing increases, patients with restrictive lung disease reach a threshold at which increasing Pa co 2 will no longer drive the homeostatic response to increase respiratory rate and CO 2 accumulates.
Presentation of Respiratory Failure and Variable Practice Patterns Regarding Management
Respiratory failure typically present in 1 of 3 ways in patients with NMD: (1) as acute respiratory failure such as that occurring in high-level spinal cord injury, Guillain-Barre syndrome, and tick paralysis; (2) as an acute respiratory decompensation in a chronic disease such as ALS or myasthenia gravis; or (3) as a chronic worsening of a gradually progressive disease such as DMD.
Treatment of acute respiratory failure frequently involves endotracheal intubation and positive pressure ventilation (PPV) in the intensive care unit. Often tracheostomy tube placement is required. In this situation, unfortunately, there are few choices of methods of ventilation. Under certain circumstances, it is possible to wean a patient from invasive PPV by using a variety of noninvasive ventilator techniques. Thus, the tracheostomy tube, which some patients find objectionable, is not necessarily permanent.
The more common presentation, with insidious onset of respiratory failure in patients with NMD, can be improved by early intervention to prevent respiratory complications and prolong life. Noninvasive forms of both positive and negative ventilation and the rocking bed have been used effectively in reversing, at least temporarily, progressive chronic respiratory failure. Initially, patients may require ventilator support for only part of the day. In these cases, nocturnal ventilatory support has been shown to be of great value. Daytime ventilation either full-time or for prescribed periods can be used as muscular weakness progresses. Thus, monitoring a patient’s pulmonary function becomes of paramount importance in directing treatment. A survey of ALS clinic directors published in 1999 was a focused query to determine common pulmonary practices. This study found that 85% of ALS clinics performed pulmonary function tests (PFTs) every 3 months during clinic visits and decisions to initiate NIV were based on PFT results. Despite this, a later study revealed that only 15.9% of patients with ALS were using therapy with NIV.
Development of Consensus Guidelines for Pulmonary Management of NMDs
Several consensus practice parameters have been developed to outline disease-specific pulmonary monitoring and management recommendations.
ALS
A consensus statement, based on a systematic review of the literature, was recently published with recommendations for pulmonary management in ALS. The American Academy of Neurology (AAN) consensus document acknowledges that management of the patient with ALS is best accomplished with multispecialty care teams. Respiratory care providers play a key role in these teams. Their focus includes 3 areas: (1) lung function assessment, (2) prevention of chest infections, and (3) ventilatory support.
Lung function assessment is used to help in the timing of percutaneous endoscopic gastrostomy tube (PEG) placement, initiation of NIV, and considerations for end-of-life care. Nutritional support has been found to be beneficial in improving survival and quality of life for both patients and care-givers. The placement of PEG tubes includes risk resulting from anesthetic and procedural complications such as aspiration, pneumonia, and ventilator failure. The lower the lung function, the greater is the risk of the procedure. The AAN recommendations suggest PEG placement at an FVC greater than 50% and placement should be performed with great caution if the FVC is less than 30%.
When using lung function testing to assess need to initiate NIV, the AAN guidelines discuss the use of testing with an MIP greater than –60, SNP less than 40, or FVC less than 50% as the time to initiate therapy ( Fig. 1 ). The guidelines from Centers for Medicare and Medicaid Services have additional suggestions for initiation of NIV including oxygen saturation of 88% or less for longer than 5 minutes or a Pa co 2 less than 45. Last, the AAN guidelines suggest the use of lung function testing to assess survival. Survival of less than 3 months is noted with SNIP of less than 30 cm H 2 O. Daytime saturation less than 95% or nocturnal saturation less than 93% had similar findings.
