Figure 4.1
Algorithm of surgical management of patients with periprosthetic joint infection
Other authors prefer to classify PJI according to the pathogenesis of the infection in early postoperative (symptoms beginning within the first month after surgery), late chronic (infection developing 1 month or more after the placement of the arthroplasty, with an insidious clinical course), haematogenous (acute onset at any time after the placement of the prosthesis, in the context of a suspected or documented bacteraemia) and intraoperative positive cultures (late chronic infection detected on intraoperative cultures taken at the time of a revision arthroplasty or a one-stage exchange procedure) [3].
Intention to Cure with Prosthesis Retention: Management by Debridement, Antibiotics and Implant Retention
Retaining the orthopaedic device, also known as DAIR (debridement, antibiotics and implant retention), is an ambitious option that has several potential advantages:
Surgical debridement is theoretically a less complex surgery than removing the prosthesis, especially with early postoperative infections where the implant may be soundly fixed. Thus, recovery from this surgery is expected to be easier.
If successful, DAIR requires fewer surgeries compared with other strategies, such as two-stage exchange procedure, for example.
Saving the primary arthroplasty allows bone stock to be preserved. This may be important for young patients who still have many years ahead of them and who are expected to need a revision prosthesis in the future.
Finally, DAIR may also be economically advantageous in some scenarios [4].
While the retention of the foreign body significantly increases the antimicrobial treatment demand, cure rates may be comparable to prosthesis removal if patients are appropriately selected [5, 6]. When DAIR management fails, prosthesis removal is frequently needed, meaning that precious time and resources are lost. Also, compared with patients who undergo prosthesis exchange as the first-line therapy, some reports have suggested that patients have a worse outcome if they require exchange because of a DAIR management failure [7]. Therefore, to maximise the likelihood of success, candidates for DAIR must be chosen with care.
The algorithm for identifying suitable candidates for DAIR, as suggested by Zimmerli et al. [2] and adapted by the Infectious Diseases Society of America (IDSA) [8], is a useful tool for choosing patients with higher odds of benefiting from this strategy [9–11]. According to this algorithm, patients undergoing DAIR should meet the following criteria:
- 1.
The infection must be acute, with a short duration of symptoms. It may be either a haematogenous PJI, with a symptom duration not exceeding 21 days, or an early postoperative PJI, with debridement being performed within 1 month of prosthesis placement.
- 2.
Periprosthetic skin and soft tissues must be in good condition so the prosthesis may be safely covered after surgery.
- 3.
The presence of a sinus tract should discourage from management by DAIR.
- 4.
The prosthesis must be stable and soundly fixed.
- 5.
The infection should be caused by microorganisms susceptible to antibiotics with a good anti-biofilm profile.
While there is wide consensus in the utility of these conditions for considering DAIR management in a given case of PJI, some specific definitions and time limits should be put into context and warrant further consideration (see below The Indication Criteria for DAIR).
Intention to Cure with Prosthesis Removal
The removal of the prosthetic device, in contrast with its retention, facilitates the eradication of the infection by removing the foreign body and necrotic tissue that serve as foci of the infection, thus leading to enhancement of antibiotic efficacy. However, prosthesis removal usually requires complex surgery that may deplete bone stock and reduce joint function.
The attempted eradication of PJI by implant removal and antibiotic therapy may be performed with prosthesis replacement (in a two-stage or one-stage exchange procedure ) or without prosthesis replacement (arthrodesis or arthroplasty resection). Prosthesis removal, especially when conducted as a two-stage exchange procedure, is preferred in cases with chronic PJI, and it should be considered an elective strategy in cases of prosthetic loosening, when the surrounding skin and soft tissue are in poor condition and when no antibiotics are available with good activity against biofilm-embedded bacteria. This surgical strategy should also be considered when DAIR has been attempted but has failed, as well as in acute PJI when a DAIR strategy is unsuitable [12, 13].
Palliative Treatment with Prosthesis Retention and Suppressive Antimicrobial Treatment
In some instances, undergoing a curative surgery (either with prosthesis retention or prosthesis removal) may not be acceptable to the patient. This may be for one of several reasons. The patient’s condition may be so fragile that the odds of surviving surgery are too low. Also, there might be a clear indication for removing the prosthesis, but without a guarantee of further successful implantation. Else, the results after prosthesis exchange may be expected to be unsatisfactory. The absence of sufficient bone stock and cases where patients have a short life expectancy are good examples of situations where palliation is appropriate. In all these scenarios, the possibility of reducing the symptoms and signs of the infection while keeping a functional (although infected) prosthesis is to be considered.
Long-term suppressive antimicrobial treatment (SAT) is the main palliative option, but does not necessarily preclude performing non-curative surgery, as will be discussed. Actually, in some cases, SAT will be chosen as a salvage option to maintain a functioning arthroplasty when DAIR has failed. Long-term antibiotic therapy may also be prescribed when patients have undergone curative management (prosthesis exchange or DAIR), but where the odds of relapse are perceived as being very high if the antibiotics are withdrawn. This last clinical scenario is rather different, belonging to the ongoing controversy regarding the length of therapy (see below Length of Therapy).
In any case, the decision to use SAT is not straightforward and it deserves careful consideration. Along with the opinion of the orthopaedic surgeon and the ID physician, the patient’s personal values and priorities must be considered, because the quality of life with or without prosthesis removal is a subjective and personal issue.
Candidates for SAT must meet the following criteria :
The prosthesis must be stable and reasonably painless (except if the pain is considered to result from inflammation/infection).
The microorganisms responsible for the infection must be identified (isolated from reliable samples), so that antimicrobial treatment may be targeted.
Oral antibiotics with enough activity against the etiological agents of the infection and without long-term toxic effects must be available.
The patient must be followed up closely, so that any treatment-related toxicity can be rapidly diagnosed, the efficacy of SAT can be monitored and the risks and benefits may be reassessed regularly.
4.2.2 General Principles of Systemic Antimicrobial Therapy for PJI
Antibiotic therapy must be selected taking into account several particularities of foreign body infections, especially (1) the drug diffusion in bone, (2) the presence of bacterial biofilm and a phenotypical bacterial tolerance to antibiotics and (3) the functional abnormalities of phagocytic cells and the presence of intracellular bacteria.
Diffusion in bone is an important factor when considering antibiotic efficacy in the setting of PJI. Bone tissue has a composition with less vascularisation compared with other tissues. The measurement of antimicrobial concentration in the bone is not straightforward, with several techniques used not being standardised. Also, in many studies of uninfected bone samples, there is no information on the ratio of intracellular to extracellular bone or on the free fraction of the antibiotic [14]. Overall, there is some uncertainty on drug concentrations in bone tissue. Bearing these limitations in mind, certain drugs have better concentration in bone, including fluoroquinolones , macrolides and linezolid (serum: bone ratio 0.3–1.2), followed by cephalosporins and glycopeptides (0.15–0.30 ) and penicillins (0.10–0.30) [14].
The bacterial biofilm constitutes a community of microorganisms adhered to a surface that are included within a self-produced polysaccharide matrix. Bacteria within the biofilm exhibit phenotypic changes, which mainly produce the expression of tolerance to antibiotics [15, 16]. Standard antimicrobial susceptibility tests in laboratories, such as the minimal inhibitory concentration (MIC) or the minimal bactericidal concentration (MBC) , are performed using planktonic bacteria in a replicating state of growth, which fail to reflect the local environment of bacterial biofilm. These indices have therefore little predictive value of the antimicrobial efficacy against biofilm-embedded bacteria. The performance of adapted in vitro studies has given place to new microbiological indices, such as the minimal biofilm inhibitory concentration (MBIC) and the minimal biofilm eradication concentration (MBEC) , which would better reflect the difficulties of antibiotics in a biofilm-associated infection [17]. In addition, previous experimental studies suggest that the use of conventional pharmacodynamic parameters is more appropriate in relation to the stationary-phase MBC (MBCstat), i.e. the area under the curve (AUC)/MBCstat [18]. Overall, improving the evaluation of clinical antibiotic efficacy in this particular scenario is still needed, as well as defining the most appropriate pharmacodynamic parameters that correlate better with the in vivo efficacy.
In any case, the activities of each antimicrobial against biofilm-embedded bacteria are not impaired proportionally. While in some cases the MBIC, MBEC or MBCstat may exceed clinically achievable concentrations, in others these concentrations may be reached by high but non-toxic doses of systemic antimicrobials. For instance, for Escherichia coli ATCC 25922, Ceri et al. reported an MIC and MBEC for cefazolin of 1 mg/L and >1024 mg/L, respectively; by contrast, these figures were 0.004 mg/L and 8 mg/L, respectively, for ciprofloxacin [17]. This phenomenon has also been observed in vivo with the tissue cage infection model, where Chuard et al. found that bacteria recovered from the animal’s foreign body had MBC values more than 100-fold greater than standard MBC for several antimicrobials [19]. Among staphylococci, susceptibility to β-lactams, glycopeptides and aminoglycosides is significantly altered, whereas fluoroquinolones and rifampin remain more active.
Along with the difficulties in reaching the bone tissue and biofilms, antibiotics may have impaired diffusion within biofilm structures and can become trapped in the extracellular matrix. This depends largely on the antibiotic’s physical and chemical characteristics and on the presence of extracellular molecules that may impair antibiotic diffusion [16].
In addition, the intracellular location of invasive bacteria has also been described in foreign body-associated infection [20, 21], where it acts as a reservoir of infection [22]. Of note, bacteria surviving phagocytosis will neither be affected by the humoral immune system nor be reached by antibiotics that do not possess intracellular activity. Moreover, some antibiotics may penetrate cells, but lack the ability to reach specific intracellular compartments where bacteria hide [22]. As occurs within biofilm structures, intracellular organisms may also enter a quiescent or other adaptive state, such as small colony variants [21–23], which increase their resistance to antibiotics.
Bearing these factors in mind, we can conclude that antibiotics for PJI should have the following properties:
Good diffusion in bone tissue
Good diffusion in biofilms
Good activity against biofilm-embedded bacteria
Good activity against intracellular bacteria
A good safety profile that allows long-term administration
4.3 DAIR Management
The true likelihood of achieving cure and retention of prosthetic devices, where the infection is managed by DAIR, is not really known. Many case series have been published, with cure rates reported as low as 18% [24] and as high as 90% in series with very well-selected patients [11, 25]. The success of this complex management strategy depends on several variables. Of these, strict application of the IDSA guideline s and the experience of the surgical team appear to be very important.
Patient’s baseline conditions also significantly affect therapeutic outcomes. Chronic renal insufficiency, rheumatoid arthritis, immunosuppressant drug uses and infection of a revision prosthesis are each associated with unfavourable outcomes [26–28]. Clinical presentation is also important, with haematogenous infections (especially in the case of staphylococcal PJI), bacteraemia and high C-reactive protein (CRP) levels associated with worse prognoses [3, 27, 29–31]. Finally, the infectious aetiology and the antibiotic susceptibility profile have a considerable influence on therapeutic outcomes.
In the following sections, we will discuss the peculiarities of DAIR management, focusing on key aspects of surgical debridement and antimicrobial therapy based on aetiology of the infection.
4.3.1 The Indication Criteria for DAIR
As mentioned, ensuring that patients meet the IDSA criteria for DAIR can increase the likelihood of treatment success. The rationale for these criteria is to select patients with acceptable local conditions at the joint prosthesis/bone interface, without a chronic infection or mature biofilm and with bacteria that may be treated with antibiotics with a good anti-biofilm profile. However, the specific cut-offs and definitions of some of these criteria are somewhat arbitrary, and some observational studies have shown that the violation of these rules does not lead unequivocally to treatment failure.
The definition of what constitutes an acute infection is controversial, because PJI may be considered acute according to two clinical criteria: (a) the duration of symptoms and signs (i.e. time from onset of symptoms to surgical debridement) and (b) the age of the prosthesis (i.e. time from the prosthesis placement to surgical debridement). The first may be difficult to establish because the onset of symptoms can be subtle, especially for postoperative cases when symptoms and signs may be masked by the expected pain and inflammation of the postoperative period. Duration of symptoms is therefore preferred for haematogenous PJI, where the onset is usually more evident.
To this end, a 21-day symptom limit was established, though it was not based on comparative studies. This cut-off was established on the maximal time that the patients included in Zimmerli’s pivotal trial were submitted to debridement [32]. However, it remains unclear whether patients could still have benefited from rifampin plus a fluoroquinolone if they had undergone surgery later. In fact, several studies have addressed this issue and suggested limits other than 21-day symptom duration. For instance, in a cohort of patients with staphylococcal PJI treated mainly with β-lactams , Brandt et al. observed that a debridement delay of more than 2 days was associated with a higher likelihood of failure [33]. However, this time could not be confirmed by later studies using rifampin and fluoroquinolones [27, 31]. Also, in a recent cohort of streptococcal PJI managed by DAIR, a long symptom duration was associated with a poor result, but the 21-day cut-off did not discriminate patients with a better prognosis [34]. Many other case series have also reported that, although a long symptom duration was associated with an unfavourable outcome, no precise and reproducible cut-offs could be provided [24, 35–40].
In this regard, it must be noted that symptom duration may be a surrogate marker of severity. Patients with sepsis, bacteraemia or purulent wound discharge may require surgery earlier than stable patients, yet a short time to debridement would paradoxically be associated with a worse prognosis because of the higher likelihood of failure in this setting [27, 31].
To conclude, no specific time limit has consistently defined a worse prognosis. In this regard, we are certain that candidates for DAIR should be submitted to debridement as early as possible, but we are unable to support the 21-day time limit unreservedly.
By contrast, the age of the prosthesis may be a more objective parameter for postoperative infections. Optimal candidates for DAIR are those with an early postoperative PJI, but again the specific definition is not without controversy. Indeed, the concept of early postoperative PJI has evolved over time in several landmark publications, ranging from 1 month [3, 8] to 2 or 3 months [2, 32]. While most physicians will agree that 3 months is a reasonable limit for PJI to be considered as chronic, thereby excluding patients from treatment by DAIR, the debate continues to focus on patients in the timeframe between the first and third month. According to the IDSA guidelines , patients with a prosthesis that is older than 1 month should not receive DAIR, yet a large observational cohort study of staphylococcal PJI showed conflicting results. In that study, the long-term prognosis of patients with a postoperative infection and prostheses aged 1–3 months was similar to that for prostheses aged 1 month or less, whereas patients with a prosthesis older than 3 months had significantly worse prognosis (Fig. 4.2) [27]. These observations were also reproduced in a large cohort of patients with streptococcal PJI [34]. Together, these findings challenge the conventional wisdom that the limit for considering DAIR for postoperative infection should be prosthesis age of 1 month.


Figure 4.2
Survival curves of postoperative (non-haematogenous) cases of staphylococcal periprosthetic joint infection managed by DAIR according to the age of the prosthesis. The figure shows a similar prognosis for 207 cases, with symptoms beginning within the first 30 days after the placement of the prosthesis (black continuous line, 38% failures) and 46 patients with onset of symptoms between 31 and 90 days after the index surgery (grey continuous line, 41% failures), whereas the outcome of 26 patients whose symptoms began after 90 days was worse (black discontinuous line, 62% failures) (long-range test, P = 0.052) (Adapted from Lora-Tamayo et al. [27], with permission)
In summary, while DAIR should certainly be considered for patients meeting the IDSA criteria, decisions should be made by a multidisciplinary team with expertise in PJI and therapy individualised to the complexities of each patient’s condition. On balance, decisions should focus on the stability of the prosthesis, the condition of the surrounding soft tissue and the clinical presentation as the most important criteria. In this regard, DAIR should be performed as early as possible and in cases of haematogenous or postoperative PJI with a prosthesis no older than 30–90 days.
4.3.2 Surgical Debridement
The mechanical removal of macroscopic (and microscopic) infection is essential to the success of DAIR management. A suboptimal debridement will probably lead to treatment failure, regardless of the quality of antibiotic treatment and medical management. Therefore, it should be performed by specialist orthopaedic surgeons who are experienced with surgery for infectious complications.
Surgical debridement must be thorough, complete, early and aggressive. It should begin by reopening the previous wound and excising any scar tissue or permanent sutures. All necrotic material, purulent collections and debris must be removed, along with the synovial tissue. Fixation of the prosthesis should be confirmed, and all inert surfaces should be generously irrigated. After this lavage, some authors recommend replacing the surgical field and instruments before wound closure [41].
The replacement of removable components is strongly recommended. This allows not only for the biofilm burden to be reduced but also for greater access to all areas of the joint space. It also facilitates lavage of most component’s surfaces. For instance, debridement of the surface of the tibial component of a knee prosthesis cannot be done unless the polyethylene liner is removed, as is valid for the posterior knee compartment (Fig. 4.3). In hip prostheses, it is also recommended that the polyethylene liner and the femoral head be exchanged. Several studies have outlined the benefits of exchanging the removable components of the prosthesis during debridement [41, 42].


Figure 4.3
Surgical debridement o f a knee prosthesis. The arrow signals debris over the tibial component of the prosthesis, which were not visible until the polyethylene liner was removed
Bearing these factors in mind, most authors recommend debridement to be performed by open arthrotomy rather than arthroscopy. An open wound permits a wider surgical window, an access to remote joint spaces, the exchange of removable components, the excision of infected scar tissues and the repair of damaged tissues. Supporting this approach, a large observational study of more than 100 patients with PJI managed by DAIR showed that debridement by arthroscopy was independently associated with failure (HR 4.2, 95% confidence interval [CI] 1.4–12.5) [26]. Some expert groups have published conflicting data, with good experience in selected patients with infected knee prostheses [43, 44]. In our opinion, the efficacy of arthroscopy for managing PJI needs further evidence before its use can be advocated.
4.3.3 Foreword on Antimicrobial Treatment for DAIR Management
Logically, the recommended antibiotic for a given PJI depends mainly on the microorganisms responsible for the infection and, therefore, on the need for a reliable microbiological diagnosis. The general regimens recommended by the aetiology of the infection may be found in Table 4.1. Before offering further insight into these particular recommendations, we should first consider some generic questions regarding the metabolic status of the bacteria involved in acute PJI and also regarding the total length of therapy.
Table 4.1
Treatment regimens for patients with periprosthetic joint infection undergoing debridement, antibiotics and implant retention (DAIR)
Bacteria | Timeb | First-line treatmentd | Alternative treatmentd | |
---|---|---|---|---|
Staphylococci | Initially | Daptomycin + cloxacillin | Cloxacillin, daptomycin or vancomycin | |
After | Rifampin + levofloxacin | Rifampin + | Daptomycin or fosfomycin | |
Rifampin + | Clindamycin, co-trimoxazole, fusidic acid or linezolid | |||
Daptomycin + | Cloxacillin, fosfomycin or levofloxacin | |||
Linezolid | ||||
Streptococci | Initially | Ceftriaxone | Levofloxacin | |
After | Ceftriaxone ± rifampin | Levofloxacin ± rifampin | ||
Amoxicillin ± rifampin | ||||
Enterococci | Ampicillin + ceftriaxone | Vancomycin or teicoplanin; alternatively, daptomycin | ||
Amoxicillin | Linezolid | |||
Gram-negative bacilli | Initially | β-Lactama,c | Ciprofloxacin or co-trimoxazole | |
After | Ciprofloxacinc | β-Lactama (consider continuous infusion) ± colistin | ||
Co-trimoxazole |
Planktonic and Stationary State of Growth of Bacteria in Acute PJI
The presence of bacterial biofilm, which involves nongrowing and stationary bacteria, is a major characteristic of PJI and other foreign body infections. Management requires appropriate surgery, and antimicrobials are usually administered in combination and at high doses. In addition, the preferred antibiotic classes can be different to those usually prescribed for common infections where bacteria in the planktonic phase of growth are predominant (i.e. bloodstream infection). In particular, the efficacy of and reliance on β-lactams are significantly reduced in the context of biofilm-associated infection, while rifampin for staphylococci (and maybe for other Gram-positive bacteria, too) and fluoroquinolones for Gram-negative bacilli acquire much greater prominence.
However, acute PJIs, which are usually suitable for DAIR, may also present with a significant component of planktonic bacteria. These infections would be characterised by the presence of bacteraemia, fever, purulent wound discharge, inflammation, high CRP levels and high leukocyte counts. In several large series of PJI with different aetiologies that were managed by DAIR, these parameters were independently associated with the treatment failure, especially in the first weeks after debridement [27–29, 31, 34].
Also of importance, the possible emergence of antibiotic resistance must be considered. In the setting of acute PJI, this is more likely in the presence of high bacterial inoculum in a high replicative state of growth, such as the days before debridement or just thereafter, when the surgical drains are still present. The risk is also higher for some antibiotic classes such as the fluoroquinolones and rifampin [45].
Thus, in addition to surgical debridement, which is key to reducing the inoculum and fighting the planktonic component of the infection, the initial antimicrobial therapy should include antibiotics with good activity against bacteria in a planktonic state of growth and with a low risk of producing early resistance. In this regard, β-lactams are probably ideal. The likelihood of resistance developing is low, and their ability to clear bacteraemia and replicating bacteria is excellent. Once this initial phase is over, a specific antibiotic regimen may be prescribed that has activity against biofilm-embedded bacteria.
Length of Therapy
Long courses are recommended for PJI managed by DAIR. Current guidelines suggest treatment durations of 3 months (hip prostheses) to 6 months (knee prostheses) [2, 8]. However, many observational studies have shown that such long treatments are probably unnecessary to achieve similar cure rates [46–48]. In this regard, the most robust information exists in the treatment of PJI caused by S. aureus and Gram-negative bacilli, using the rifampin –fluoroquinolone combination and the fluoroquinolone monotherapy, respectively. Hypothetically, there may be a time point beyond which extending treatment would not add further benefit. Byren et al. reported a higher risk of failure in the weeks or months after antibiotic withdrawal, but also observed that the risk was not higher for patients treated for shorter periods [26]. Unfortunately, using biological parameters like the CRP does not seem to be a reliable aid when deciding whether to continue or to stop antibiotics [49].
The only clinical trial assessing the comparative efficacy of short and long antibiotic treatment has been recently performed by our multicenter group (REIPI). In this study, 63 patients with acute staphylococcal PJI were managed by DAIR and were randomised to receive levofloxacin plus rifampin during for either 8 weeks (short schedule) or 3–6 months (long schedule). Unfortunately, the study was underpowered, and the non-inferiority hypothesis could only be confirmed in the intention-to-treat analysis, with cure rates of 58% and 73% for the long and short schedules, respectively. There was only a trend toward similarity in the per-protocol analysis (cure rate 95% and 91.7%, respectively) [50].
Overall, we believe that most patients with acute PJI managed by DAIR may be safely treated for 8 weeks with the use of an appropriate anti-biofilm therapy (rifampin-fluoroquinolones for staphylococci and fluoroquinolones for Gram-negative bacilli). Cases with suboptimal debridement or poor resolution of inflammation should be addressed separately.
4.3.4 Treatment Regimens for Staphylococcal Infection Managed by DAIR
Staphylococcus aureus is the most common pathogen associated with acute PJI and so has been the focus of many clinical and experimental studies. According to published case series, the success rate for DAIR management of PJI caused by S. aureus ranges from 13% to 90% [6, 25–27, 31, 33, 35, 38]. Our group previously reported a success rate of 55% (95% CI, 50–61%) in a large observational study of 345 patients. Acute infection caused by coagulase-negative staphylococci (CNS) is less frequent, but clinical management appears to be similar [51].
Experimental Research: The Tissue Cage Model
Randomised and prospective studies are very difficult to perform for bone and joint infections. Therefore, much of our knowledge about the activity of antimicrobials in staphylococcal foreign body infections come from the laboratory and animal experiments. In the last years, the tissue cage model , performed with either Wistar rats or guinea pigs, has played a main role to improve our knowledge about the particularities in the pathogenesis of biofilm-related infections [52–54].
The model is considered as a reliable tool to mimic these infections, and it has been widely used to test the activity of antibiotics against biofilm -embedded bacteria [55, 56], providing relevant information for the clinical practice. Indeed, there are other models of device-related infections that have produced very interesting information, such as osteosynthesis-associated osteomyelitis [57] or actual periprosthetic joint infection [58]. However, the tissue cage model has been validated and used by different research groups for more than 30 years, and it has been proven as a reliable easy method that is relatively less expensive.
Briefly, the tissue cage model involves subcutaneously implanting 2–4 perforated polytetrafluoroethylene (Teflon) tissue cages containing two polymethylmethacrylate coverslips. Once the wounds heal (usually 3 weeks later), a standard inoculum is introduced percutaneously (usually 1 × 104 to 8 × 105 colony-forming units). After a variable period (24 h to 3 weeks), which determines the chronicity of the infection, antimicrobial regimens are given during several days (usually 4–14 days). The inflammatory liquid in the tissue cages may then be sampled percutaneously, and viable bacteria measured quantitatively, allowing antibiotic activity to be tested and compared. Thereafter, tissue cages and coverslips may be recovered to perform quantitative counts of any biofilm-embedded bacteria [18, 59, 60]. Table 4.2 summarises the most important studies using this model for staphylococcal foreign body infection [18, 52, 59–81].
Table 4.2
Summary of antibiotic activity in the experimental tissue cage model for staphylococcal infection
References | Bact. | Chron/lengc | Antibiotics tested | Results and comments |
---|---|---|---|---|
Widmer [18]b | MRSE | 24 h/4 d | DAP, NET, TEI, VAN, CPX, RIF | Experimental evidence of the central role of rifampin-based regimens. RIF + CPX was the best treatment |
Lucet [59]a | MRSA | 14 d/7 d | RIF, FLX, VAN | RIF combinations were the most effective therapies |
Chuard [52]a | MRSA | 21 d/21 d | RIF, FLX, VAN | |
Schaad [61]a | MRSA MSSA | 21 d/7 d | TEI, VAN, RIF | |
Schaad [62]a | MRSA MSSA | 21 d/7 d | VAN, IMI, OXA | IMI performed better than OXA for MSSA, but was ineffective for MRSA |
Blaser [63]b | MSSA MRSE | 24 h/4 d | RIF, NET, CPX, FLX | Main role of RIF combinations |
Cagni [64]a | MRSA | 21 d/7 d | VAN, SPX, TMX, CPX | Evaluation of new fluoroquinolones (not currently available) |
Vaudaux [65]a | MRSA | 14 d/7 d | LVX, ALX, VAN | LVX was the most active treatment, with no development of resistance |
Vaudaux [66]a | MSSA | 14 d/7 d | DAP, VAN | DAP and VAN activities were alike |
Vaudaux [67]a | MRSA | 14 d/7 d | CFB, VAN | |
Schaad [68]a | MSSA | 21 d/7 d | DAP, VAN, OXA | DAP performed better than VAN and OXA |
Murillo [60]a | MSSA | 21 d/7 d | RIF, VAN, LVX (two dosages), LNZ, CLX | LVX at high doses proved similar activity as RIF |
Murillo [69]a | MSSA | 21 d/7–14 d | RIF, LVX | Antagonism of RIF + LVX at high doses |
Murillo [70]a | MSSA | 21 d/7–10 d | RIF, LNZ, CLX | |
Vaudaux [71]a | MRSA | 14 d/7 d | TIG, VAN | VAN and TIG showed similar activity |
Murillo [72]a | MSSA | 21 d/7 d | LVX, MXF (two dosages) | LVX and MXF showed similar activity |
Murillo [73]a | MRSA | 3 d/7 d | RIF, DAP, VAN, LNZ | DAP at high doses was as effective as RIF |
John [74]b | MRSA | 3 d/4 d | RIF, DAP, VAN, LNZ, LVX | LVX + RIF and DAP + RIF were the best treatments |
Garrigós [75]a | MRSA | 3 d/7 d | RIF, DAP, VAN, LNZ | DAP + RIF was the most effective combination |
Garrigós [76]a | MRSA | 3 d/7 d | RIF, VAN, TIG | |
Garrigós [77]a | MRSA | 3 d/7 d | RIF, CLX, DAP | Anti-MRSA comparative efficacy of DAP + CLX combination |
Garrigós [78]a | MRSA | 3 d/7 d | RIF, IMI, FOS, DAP | Remarkable efficacy of DAP + RIF and FOS + RIF combinations |
El Haj [79]a | MSSA | 7 d/7 d | RIF, CLX, DAP | Anti-MSSA comparative efficacy of DAP + CLX and CLX + RIF combinations |
El Haj [80]a | MSSA | 7 d/7 d | RIF, LVX, DAP | Comparative efficacy of DAP + RIF, LVX + RIF and DAP + LVX |
El Haj [81]a | MRSA MSSA | 3 d/7 d | RIF, CLR, DAP | CLR did not prove anti-biofilm effect |
Recommended Regimens for Staphylococcal Infection
The initial treatment of methicillin-susceptible S. aureus (MSSA) or methicillin-resistant S. aureus (MRSA) PJI after debridement should be based on either β-lactams (i.e. cloxacillin or cefazolin) or glycopeptides, respectively [2, 8]. There is a long experience with both antibiotic families for the treatment of staphylococcal planktonic infection, with the likelihood of treatment-emergent resistance being low [45]. However, the activity of cloxacillin may be impaired when the bacterial inoculum is high, and vancomycin’s efficacy is often suboptimal. Interestingly, combination therapy with daptomycin plus a β-lactam has shown synergism for both MSSA and MRSA in both in vitro and in vivo models [82, 83], with evidence of good activity against biofilm-embedded bacteria too [77, 79]. These results would encourage the use of daptomycin plus cloxacillin in the first days or weeks after debridement, although clinical experience is lacking.
After this initial period, a targeted anti-biofilm treatment may be started, which is determined by the addition of rifampin .
Schemes Including Rifampin
The activity of rifampin -based combinations against biofilm-embedded S. aureus has been shown in several animal models (Table 4.2). Importantly, rifampin resistance can be avoided by adding a second drug [18, 19, 59, 61, 63]. Widmer et al. reported an 84% success rate in a pilot study of patients with orthopaedic hardware-associated infection managed by implant retention and rifampin-based combination therapy [84]. In a similar study of ofloxacin plus rifampin, Drancourt et al. reported an overall cure rate of 74% and a cure rate of 57% among patients managed with implant retention [85].
In the context of this background, Zimmerli et al. published a landmark double-blind randomised clinical trial in 1998, comparing ciprofloxacin alone with ciprofloxacin plus rifampin for orthopaedic hardware-associated acute infection caused by MSSA managed by implant retention. The per-protocol analysis showed a significantly higher success rate for the combined therapy (100% (12/12) vs. 58% (7/12), P = 0.02). This pivotal study forms the clinical basis for using rifampin in staphylococcal PJI managed by DAIR [32].
Later observational studies have confirmed the benefits of rifampin. In a large study of staphylococcal PJI, Senneville et al. observed that fluoroquinolone plus rifampin was independently associated with a lower likelihood of failure (odds ratio 0.40, 95%CI 0.17–0.97) [6]. The benefits of rifampin also seem to apply to MRSA, as shown in experimental models [19, 59, 62, 74, 75]. Case series of staphylococcal PJI where the use of rifampin-based combination therapy was widespread have shown a similar prognosis for MRSA and MSSA episodes [27, 51].
The recommended dose of rifampin is another matter of controversy. In the landmark trial by Zimmerli et al., the dose was 450 mg twice daily [32]; by contrast, the IDSA guidelines use a wider dose range of 300–450 mg twice daily [8]. Other groups have also reported their experience with a dose of 600 mg once daily, similar to the regimen used for tuberculosis. In this regard, the area under the curve (AUC) in relation to the MIC seems to be the best pharmacokinetic/pharmacodynamic parameter for predicting efficacy [86]. It must be noted that the transport of rifampin in the liver is saturable, producing non-linear increases in the AUC for single doses exceeding 300–450 mg. Given this, it is likely that a single fasting dose of 600 mg causes AUCs similar to those seen for 450 mg twice daily [87, 88]. In addition, rifampin dose higher than 600 mg has not been associated with any improvements in outcome [89].
As mentioned, resistance to rifampin develops rapidly and predictably when administered alone, therefore necessitating the use of combination therapy. Beyond this, there is interest in evaluating the relative activities of specific combinations. In this regard, fluoroquinolones have a good anti-staphylococcal activity, bone diffusion and bioavailability [14, 90]. Clinical comparison of fluoroquinolone-rifampin regimens with other rifampin-based combinations is scarce, mainly because of the inherent limitations of retrospective studies. Still, there is some indirect clinical data suggesting that fluoroquinolone-rifampin combination therapy may be superior to other regimens. In our large study of staphylococcal PJI managed by DAIR, treatment with fluoroquinolones plus rifampin delayed the moment of failure until the antibiotics were stopped, whereas patients treated with other rifampin-based antimicrobial regimens did fail despite continued treatment [27].
Regarding the specific fluoroquinolone choice, there is wide consensus that newer fluoroquinolones such as levofloxacin or moxifloxacin are preferable to ciprofloxacin because of their higher anti-staphylococcal activity and the lower likelihood of resistance developing during treatment [90, 91]. On the basis of several clinical experiences, expert recommendations and our own opinion, the combination of levofloxacin plus rifampin is currently the best choice in this setting [2, 8]. Moxifloxacin has lower MIC values [90, 92], but the experimental animal model has failed to provide evidence of superiority over levofloxacin [72]. Also, the antimicrobial spectrum of moxifloxacin is unnecessarily wide for treating staphylococcal infection (i.e. it also includes anaerobic microorganisms). Finally, there is evidence that moxifloxacin levels may decrease when combined with rifampin [93].
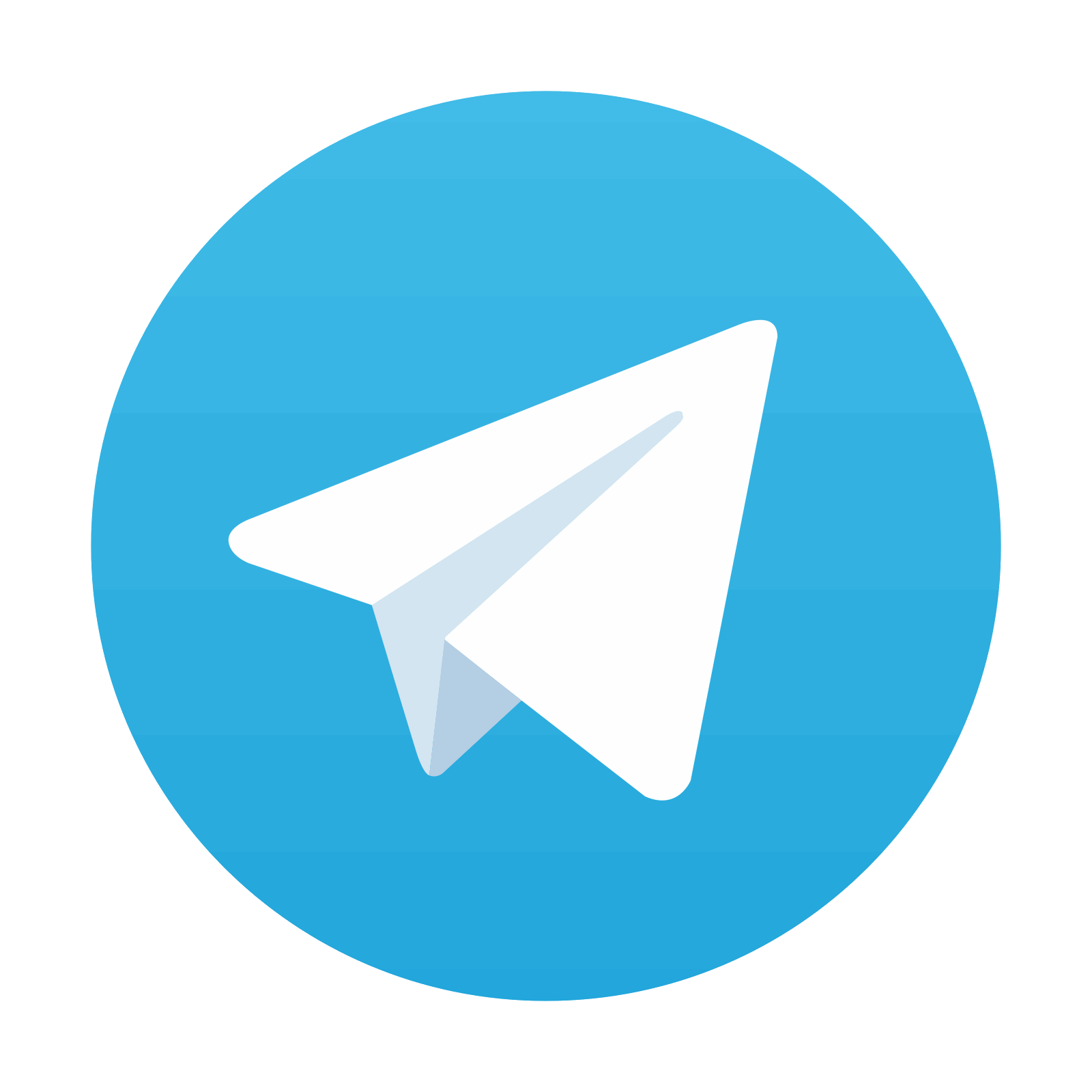
Stay updated, free articles. Join our Telegram channel

Full access? Get Clinical Tree
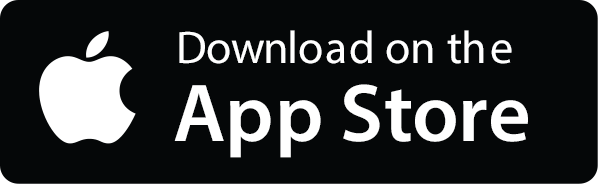
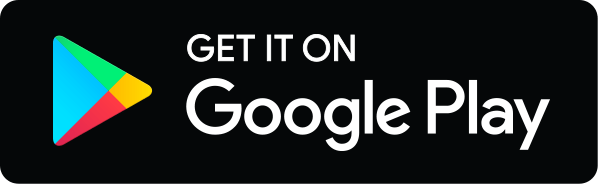