5 Management of Complications of Surgery of the Median, Ulnar, and Radial Nerves in the Forearm
Erik Walbeehm and Dominic Power
Abstract
Keywords: median nerve, ulnar nerve, radial nerve, nerve transfer, tendon transfer, neuroma, neuroma in continuity, neuropathic pain, neurolysis, paralysis
5.1 Introduction
Reconstruction following a traumatic peripheral nerve surgery is straightforward. Following a thorough physical examination and applying anatomical knowledge, most primary nerve pathologies in the forearm and hand can be diagnosed and treated. If a nerve is transected it can be repaired. Simple apposition of the two nerve ends with a number of microsurgical sutures and perhaps fibrin glue augmentation will suffice in most cases. If there is nerve tissue loss and too much tension, then consider a conduit as a bridging device or for longer gaps a nerve graft. This algorithm is easy to follow. However, unlike for a microvascular anastomosis, the final outcome following a nerve reconstruction must be awaited. The primary surgery must be well planned, promptly delivered, and performed with technical expertise. The local pathoanatomy dictates the potential for recovery and the expert clinician will be prepared for the challenges afforded by proximal nerve injuries, delayed presentation, long gaps, and poor surgical beds, offering adjunctive distal nerve or tendon transfers at the time of primary surgery or early in the recovery phase.
Failure of the repair can result in sensory loss, paralysis, and pain. Poor results may not be apparent for months and the opportunity for revision missed, leaving only salvage options. The clinician must anticipate recovery, monitor for any adverse signs, and be prepared to intervene if recovery is delayed.
5.2 Optimizing the Nerve Repair
An injured nerve should be recognized early and promptly explored. The management depends on the location and severity of the injury as well as associated injury. Debridement must be adequate, repairs should be tension free. Conduits may be used to de-tension repair sites or to leave short gaps up to 5 mm as a method of de-tensioning.1 Theoretical advantages of adjunctive wrapping of the repair site or protecting a neurorrhaphy with a conduit to prevent axonal escape and extrinsic scar tether remain unproven. Longer gaps will generally require a nerve graft; however, a concomitant humeral fracture may be shortened and plated, avoiding the need for a graft. Avoiding two coaptation sites has obvious advantages in minimizing the risks of hold-up or misdirection of the regenerating axons. In the forearm, skeletal shortening is generally not advisable due to the loss of rotation; however, in a complex mangling injury, judicious use of this technique can assist with management of the soft tissues, including nerve reconstruction. Allograft reconstruction as an alternative is attractive, minimizing donor morbidity; however, the comparative efficacy to autologous graft in main trunk mixed nerve injuries is not known.
Recognizing the challenging injury perhaps due to a delay to diagnosis, proximal location, large gap, or poor surgical bed may allow the treating surgeon to introduce adjunctive primary tendon or nerve transfers into their primary treatment algorithm. Establishing that a repaired nerve is not progressing in line with expectation may enable prompt secondary interventions.
Following surgery, preparing your patient for a period of months before a return of function or sensation helps to manage patient expectations, prepares them for what is to come, mitigates the effects of neuropathic pain, and improves engagement, thereby preventing trophic complications.2 This chapter focuses on problems and complications in the weeks to months following primary surgery, with the assumption that the primary surgery has been adequately performed.
5.3 Normal Recovery
Physiologically it is impossible to achieve accurate reconnection of all axons, even with acute repair of a sharp transection injury to a peripheral nerve. The mechanism of injury determines the survival of the axon population at the injury site. Proximal lesions are associated with more apoptosis, although surviving axons may have more vigorous axonal regeneration than distal injuries with a similar mechanism. Progressive loss of the sensory and motor neuron population in the proximal nerve trunk follows trauma, necessitating early intervention.
Following repair, regenerating axons may fail to cross the coaptation due to scar. Grafts provide two coaptation sites to navigate with less robust axonal regeneration to the distal stump than for a single repair site without tension. Each regenerating axon has multiple small exuberant projections that explore the local environment. Contact with surface proteoglycans in the endoneural tubes and neurotrophic gradients provide positive guidance signals. Axons failing to receive positive stimuli are pruned, allowing redistribution of regenerate efforts. In the absence of a strong positive signal, the multiple axon projections will form a disorganized microfascicular array, typical of a neuroma. The varied array of fiber types in a mixed nerve is a challenge. A mismatch of motor and sensory axons at the coaptation can cause fibers to enter the wrong type of Schwann cell sheath, failing to reach an appropriate target organ and eventually resulting in a nonfunctional recovery and subsequent cell death. Small fibers may regenerate more vigorously, with large fibers requiring mature myelin sheaths, taking longer to grow to the target and become functional. Brushart described preferential motor reinnervation.3 Those axons that do reach their correct target organ will try to reconnect and regain function. Although the regenerated axons are fewer in number than the original pool, the human body has amazing adaptive physiology. Regenerated motor axons may innervate more muscle fibers than in the natural preinjury state, creating functional, albeit larger, motor units. This process of “adoption” of denervated muscle fibers by collateral sprouting from adjacent innervated muscle fibers was described by Brunelli4 and explains the giant motor spikes measured by EMG following muscle reinnervation. Large motor units, coupled with loss of matched motor afferents, contribute to the loss of fine motor skills following recovery from injury to a motor nerve.5
Similarly, there is a resprouting of epidermal axons in the skin. Interestingly, this is a dynamic process. In rats following a sciatic nerve transection and reconstruction, collateral sprouts from adjacent intact fibers within the saphenous nerve spread to the denervated tibia nerve domain of the plantar foot. After 10 to 12 weeks, these fibers recede, when the regenerating tibial nerve fibers reach that area.6,7,8,9 The adjacent collateral sprouting may reduce the impact of sensory loss after nerve injury, even without a complete recovery10 and coupled with synaptic changes in the central nervous system, may contribute to the marginal hypersensitivity in cases where there is poor reinnervation in the injured nerve territory.
5.3.1 Timing of Normal Recovery
The rate of regeneration and functional recovery is dependent on a number of factors. Proximal injuries have faster initial axonal growth due to the proximity of the injury to the cell body. However, the long reinnervation distance to the distal targets leads to a prolonged period of denervation. Axonal growth is approximately 1 mm per day following a complete nerve injury transection and repair, although lower grades of axonopathy may recover more rapidly. Regeneration is less robust in more complex trauma when fewer cells survive the initial injury. Younger patients may have more resistance to apoptosis following injury, shorter limbs enable functional recovery more quickly than in adults, and the cortical plasticity potential in children enables better functional use when there is axonal misdirection following regeneration from a proximal injury. Clinical recovery may be monitored using the rate of progression of Tinel’s sign after repair. The tender muscle sign may predate successful motor reinnervation and polyphasia may be detected on electromyography.11
5.4 Problems with Recovery
A surgical complication is any undesirable, unintended, and direct result of an operation affecting the patient which would not have occurred had the operation gone as well as could reasonably be hoped.12 Nerve recovery after injury is frequently problematic, with neuropathic pain affecting the majority and trophic ulceration affecting denervated skin and stiff joints from disuse. Defining a complication is challenging. The Clavien-Dindo classification may be used to define variations from the planned postsurgery rehabilitation phase, with different degrees of severity indicated by unplanned therapy interventions, additional medication requirements, unplanned hospital admissions, or reoperations.13,14
5.4.1 Neuropathic Pain
Neuropathic pain follows disease or damage to the somatosensory nervous system. Pain is an inevitable consequence of peripheral nerve injury. There is normal nociceptive stimulation due to the injury and inflammatory response. However, disruption of both sensory and motor afferent signaling leads to modulation of the normal nociceptive pathways, increasing responsiveness to normal nociception. Maladaptive cortical plasticity leads to central sensitization, such that spontaneous pain may be experienced in the absence of nociceptive stimulation. Peripheral sensitization is associated with repair site neuromas and the abundant axonal sprouting results in chaotic immature microfascicles that are a source of both spontaneous pain and evoked pain from mechanical stimulation.15 Marginal hypersensitivity results from collateral sprouting of intact cutaneous nerve ends in adjacent innervated skin in addition to modulation of normal sensory responses within the central nervous system. Scar tether of the repaired nerve may lead to neuropathic pain triggered by movement, known as neurostenalgia.
Chronic pain is associated with a significant emotional impact with high rates of anxiety and depression. Coupled with the physical impact of the nerve injury, the socioeconomic impact of a nerve injury is profound. In a group of 31 patients, followed for 12 months following median and/or ulnar nerve repair, 21 patients were pain free. The remainder, with neuropathic pain, demonstrated greater impairment on tests of sensorimotor nerve conduction, worse sensory and motor recovery, as well as different personality structures and belief structures.16 In a rodent peripheral nerve injury model, an unrepaired tibial nerve leads to the development of neuropathic pain characteristics. Delayed nerve repair is associated with neuropathic pain chronicity and poorer outcomes in humans. Undoubtedly, pain has a strong association with poor quality of life measures and also impedes functional recovery.17 Davis and Curtin provide an overview of management of pain in complex nerve injuries.15
5.4.2 Scar
Scar is a normal consequence of injury and surgery. Scar within the nerve may follow under debridement, poor coaptation technique, excessive tension across the repair site or dehiscence of the repair site. Intraneural scar blocks axon regeneration resulting in a neuroma-in-continuity. Rupture of the repair may result in an end neuroma at the proximal stump. Nerves rely on a delicate paraneurium to allow physiological glide during functional movement. Nerves are flexible and can lengthen up to 8% strain before onset of irreversible changes to function. Following injury the nerve may scar within the surgery bed. Scar is more common in complex trauma with extensive tissue damage, poor vascularity, contamination, or following infection. Scar may constrict the nerve, resulting in ischemia, impaired conduction, and pain.18,19,20 Scar may tether the nerve, preventing glide and producing neurostenalgia (neuropathic pain on passive stretch). Controlling scar at the site of nerve repair is a focus of contemporary research. Pharmacological manipulation of the intraneural milieu has demonstrated promise in preclinical studies. Atkins et al demonstrated in M6PR/IGF2 null mice, which produce significantly less scar, that collagen staining decreased, compound action potentials increased, conduction velocities increased, and fiber counts distal to the repair site increased. This was in contrast to normal operated control mice and IL-4/IL-10 null mice, which produce increased scar.21 To date, minimizing scarring, with anti-TGF β, has shown promise in rats. Tacrolimus administration has demonstrated enhanced axonal regeneration and reduced collagen formation. These results have not been translated to nerve repair protocols in humans, although studies evaluating the use with local delivery to minimize systemic side effects are gaining momentum. The effect of scar on the injured nerve has attracted attention from the biomedical device industry and innovation has resulted in the marketing of nerve connectors, using conduits as adjunctive detensioning devices for suture coaptation, entubulation of repair with suture-less coaptation,22,23,24 and the use of biological and synthetic antiadhesion barriers.25 Following re-exploration for neurolysis for repair site scar, autologous adipofascial tissues may be raised as vascularized pedicled flaps to resurface or even wrap a section of scarred nerve. Harvest site morbidity may be avoided by using commercially available wraps. Biological wraps are available with bovine, porcine, or equine origins and synthetic bioresorbable polymers provide alternative solutions. A porcine extracellular collagen-layered matrix from bowel submucosa has demonstrated increased vascularity in the nerve environment, allowing restoration of the normal mesoneurium. Antiadhesion gels containing carbohydrates or hyaluronic acid are commercially available, although there is no robust evidence to support their use currently.
5.4.3 Neuroma Formation
Following a transection of a peripheral nerve, damaged axons will repair their damaged cell membranes protecting them from further damage. Upregulation in the cell body prepares for regeneration. Axoplasmic transport carries new materials to the injury site. Surviving axons will expand and commence sprouting with multiple small filopodia. The growth cone represents this regenerative front, exploring the local microenvironment and responding to contact stimulation and neurotrophic feedback. Successful biofeedback will guide and strengthen regeneration. Without feedback, uncontrolled and disorganized proliferation of axons in scar creates a neuroma with histologically an array of microfascicles containing small and unmyelinated axons. Green’s definition of a neuroma is “the inevitable, unavoidable, and biologic response of the proximal stump after it has been divided in situations where regenerating axons are impeded from re-entering the distal stump.”26 Therefore in every transected nerve, which is not reconstructed, there will be a fusiform swelling, or a neuroma, at the end of the proximal stump of a nerve. Every transected and unreconstructed nerve will form a neuroma, but this does not automatically follow that it will be symptomatic. Following a partial nerve injury with transection of some fascicles or a traction injury with some preservation of the nerve sheath, a neuroma-in-continuity may form at the site of injury. Following a repair, aberrant axon sprouting and scar will create a repair site neuroma. Typically, some axons will successfully populate the distal nerve segment and the recovery is incomplete. The need for secondary intervention on the postrepair neuroma-in-continuity will be determined by the proportion of axons reaching their targets, the site of the neuroma, and any scar tether. Superficially located neuromas are more commonly symptomatic due to their susceptibility to mechanical stimulation.
Symptomatic Neuroma
A clinically symptomatic neuroma is determined by spontaneous (basal and spikes) and evoked pain with a positive Tinel’s sign at the site of injury. The pain is frequently described as having burning, shooting, electric shocks, pins and needles, crushing, or pressure characteristics. Local contact will evoke pain and this forms the basis of the Tinel’s sign, reproducing unpleasant sensory perceptions in the territory of the injured nerve when tapping gently at the site of the suspected neuroma. Hyperalgesia and allodynia may be present with partial recovery through a neuroma-in-continuity; however, in an end-neuroma, the presence of these features in the distal cutaneous territory may be a sign of marginal hypersensitivity. There may be additional features typical of any nerve injury including cold sensitivity and a basal aching pain. Confirmation of the diagnosis is through pain reduction following a diagnostic local anesthetic nerve block.27 Imaging with ultrasound or magnetic resonance neurography may have a limited role in confirming the site of neuroma; however, symptomatic neuroma remains a primarily clinical diagnosis.
Van den Avoort et al demonstrated that in only 7% of digital amputations neuromas become painful28 and the rate of repair site neuroma in repaired digital nerves is approximately 5%.29 Every neuroma may have the potential to produce pain. Perhaps the resilience of the individual has a role, or the reconfiguration of the spinal synaptic connections that rapidly follow nerve injury acts to suppress the pain successfully in the majority of cases. Smits et al identified that patients reporting cold intolerance demonstrated a disordered conditioned pain modulation (CPM) system30 which is an inherent mechanism for pain suppression at the spinal cord level, tested by assessing whether a pain stimulus remains painful on provision of a strong contralateral limb pain stimulus. Neuromodulation therapy may reduce the pain severity and impact of a neuroma in some cases. Coupled with cognitive pain management strategies during the reinnervation phase and neurorehabilitation with mirror therapy, patients may avoid surgery.
5.4.4 Surgical Management of Symptomatic Neuromas
The surgical management of the painful neuroma depends on whether it is an end neuroma, a neuroma-in-continuity without useful distal function, or a neuroma-in-continuity with useful intact or recovered distal function.
Active Reconstruction Techniques
This term defines restoration of the original nerve gap allowing effective axonal regeneration to the original targets with the expectation of return or sensory and motor function.
Autologous Nerve Grafts
End neuromas, neuromas-in-continuity without function, and failed neurolysis for a neuroma-in-continuity require excision and reconstruction. The ideal technique is an active reconstruction procedure restoring physical continuity of the original nerve, reinnervating the distal targets, and restoring afferent signaling along the original neural pathway. Nerve autografts or allografts may be used in such cases. Typically, the resection gap is too long for bridging with a conduit in such salvage cases. The recipient site must be debrided to demonstrate fascicular structure, vascularity, and absence of intraneural scar to expose a cut nerve face capable of regeneration. There is no gold standard for debridement; however, neurotomes allow the nerve to be grasped without injury and the guillotine blade may be used to cut the nerve without crushing. Serrated microsurgical scissors are adequate for small diameter nerves; however, the main trunk nerves with dense scar are best managed with a blade. The nerve gap between the proximal and distal stumps must be measured in the anatomical position. Autograft requires harvest of an expendable cutaneous nerve to reconstruct the missing or damaged segment. There is a diameter mismatch and several cables of autograft will be used to build up the nerve gap to match the volume of the missing nerve. As such the length of autograft is often considerable and one or both sural nerves may need to be harvested from the lower limbs. The autologous graft cables are sutured into position and augmented with a fibrin glue. Coaptation sites may be wrapped; however, there is no evidence of additional efficacy to date in this type of reconstruction. The nerve graft must revascularize from the ends and additionally from the surgery bed. The high surface area conferred by the cable technique may aid this process. Animal studies have demonstrated that the main route of revascularization is through longitudinal growth from the proximal stump.
Nerve Allografts
Processed nerve allografts offer an attractive proposition for reconstruction of the failed nerve repair with a neuroma in continuity.31 In a patient with established pain sensitization, the risk of donor site complications and symptomatic neuroma formation is considerable. There is no doubt that the published evidence supports the use of allograft in the management of symptomatic sensory nerve neuromas. However, to date, the evidence to support allograft for motor and mixed nerve reconstruction is limited. Allograft can be offered subject to appropriate consent and may have additional value in cases where lower limb surgery and general anesthesia is contraindicated. At this stage, it is recommended that allograft is used when functional reconstruction is not anticipated such as the longstanding lesion or in cases where an adjunctive distal motor reconstruction is planned with nerve/tendon transfer and therefore the prime objective of the allograft reconstruction is pain resolution and protective sensation. The current upper limit of gap length for allografts is 70 mm, although for sensory nerve reconstruction the robust evidence remains in gaps up to 50 mm only. In large nerve trunks, allograft can be used in a cabled configuration with individual allografts available in diameters between 1 and 5 mm.
Extra-anatomic Reconstruction Techniques
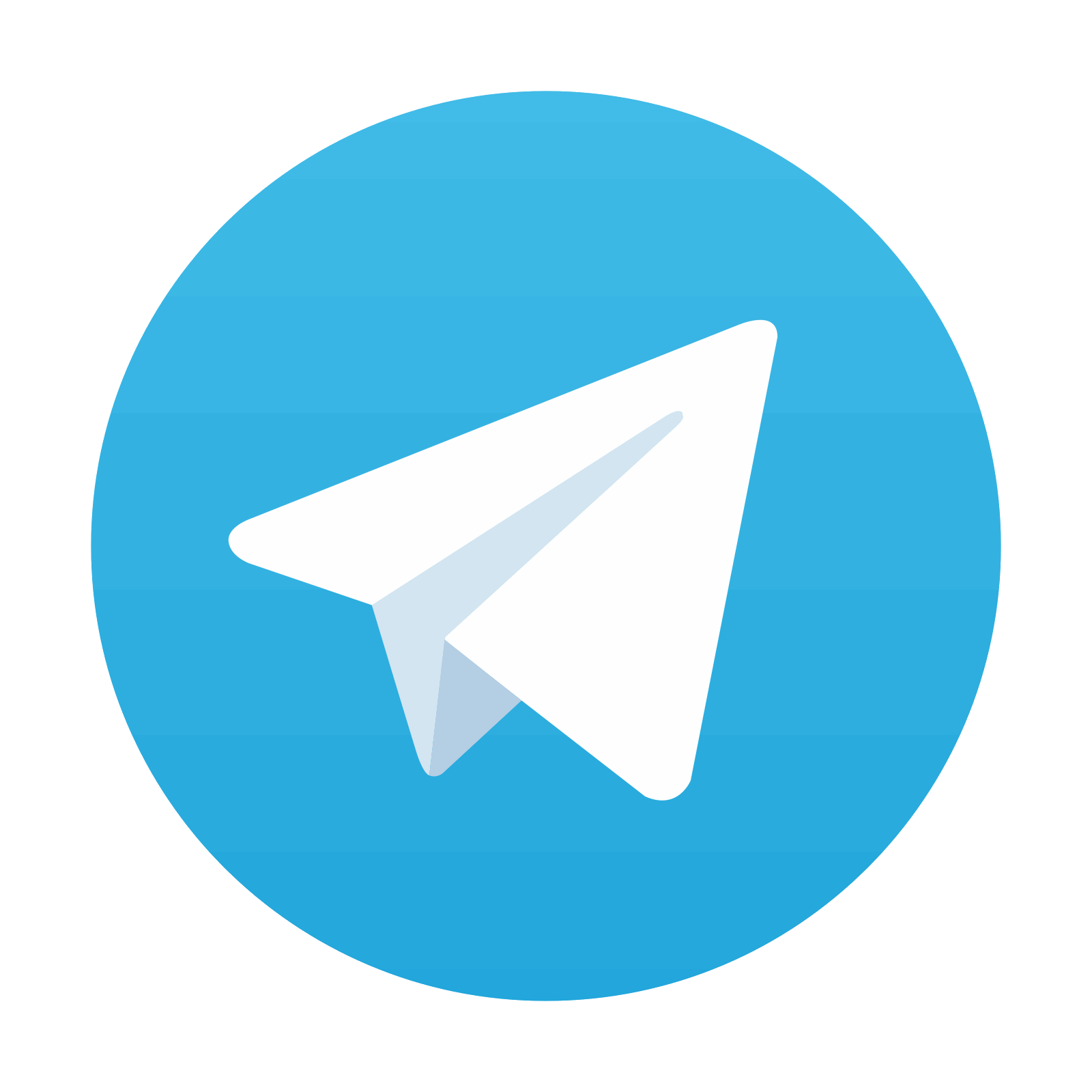
Stay updated, free articles. Join our Telegram channel

Full access? Get Clinical Tree
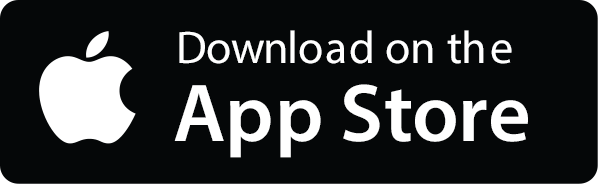
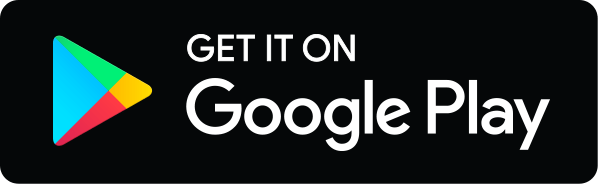