8
Magnetic Resonance Imaging in Multiple Sclerosis
Christopher J. Karakasis, Aliye O. Bricker, and Stephen E. Jones
KEY POINTS FOR CLINICIANS
• MRI remains a mainstay for diagnosis and disease monitoring for multiple sclerosis (MS), with the goal of therapy to achieve no evidence of disease activity (NEDA).
• Current trends are to standardize MS imaging protocols, reporting, and acquisition and analysis of quantitative data, in particular incorportating volumetric sequences, such as T1 and fluid-attenuated inversion recovery (FLAIR).
• 7 T MRI is useful for delineation and evaluation of perivenous lesions, while the analysis paradigm of these lesions continues to evolve. Magnetic resonance fingerprinting (MRF) is a recently invented technique that may further advance MS imaging in the near future.
• Progressive multifocal leukencephalopathy (PML) may be monitored in the setting of monoclonal antibody therapies for MS.
Like many diseases, MS was well known long before the introduction of MRI in the early 1980s. Although MS is fundamentally a clinical diagnosis supported by detailed history, careful neurological examination, and often additional bloodwork and sometimes cerebrospinal fluid (CSF) assessment, MRI has had a growing impact on accurate and early diagnosis. It is also now an integral part of monitoring disease activity (1,2). MRI of the central nervous system (CNS) can support, supplement, or even replace some clinical criteria necessary for the diagnosis (3–6). MRI can clearly demonstrate multiple aspects of known MS pathology, such as dissemination in time (DIT) and dissemination in space (DIS). The former is shown by changes in serial MRI imaging, or the simultaneous appearance of lesions with different ages (e.g., some enhancing with gadolinium and some not enhancing). The latter is shown by multiple and widespread lesions. For example, estimates show more than 95% of patients with clinically definite MS having multifocal cerebral white matter lesions on brain MRI (1). MS is known to have an early inflammatory phase, which is shown by enhancement after intravenous administration of gadolinium contrast, resulting from breakdown of the blood–brain barrier. Subsequent to the acute phase, localized loss of oligodendrocytes and axonal fibers manifests on MRI as chronic white matter lesions, which slowly accumulate over time. In addition, the disease enters a degenerative phase, causing volume loss of both white and gray matter. Although MS is classically considered as a demyelinating disease involving white matter of the brain and spinal cord, gray matter involvement is also prevalent but more difficult to image. Newer imaging techniques including postprocessing and high field strength imaging may help in demonstrating this involvement. In addition, the growing development of volumetric brain measurement techniques may reveal the subtle progressive volume loss associated with the degenerative phase, which is not readily detected on routine visual inspection.
RELEVANT MRI PHYSICS
The strong magnetic field created by an MRI scanner causes hydrogen nuclei throughout the body’s water molecules to align. By perturbing the magnetic field using the application of magnetic field gradients and radiofrequency electromagnetic radiation, whose orchestration is termed a “sequence,” the alignments of these hydrogen nuclei can be altered in subtle ways to produce a detectable signal. Sophisticated analysis algorithms can use these signals to reconstruct images, where the grayscale contrast takes on different meanings depending on the details of the sequence. Furthermore, different tissue types such as fat, fluid, muscle, and the gray and white matter in the brain, all have different hydrogen nuclei concentrations and relaxation properties. These influence the depicted MRI image, for example, ranging from dark to bright signal 65intensity. MRI is sensitive to the details of water concentration and is an exquisitely sensitive modality for detecting focal abnormalities, particularly in the white matter.
Different MRI sequences have been developed to best emphasize certain tissue characteristics. For example, T1-weighted sequences cause water to be dark, or have low signal intensity, while fat demonstrates bright signal. Conversely, T2-weighted sequences cause water and tissues with high water concentration to show bright signal intensity. The fluid-attenuated inversion recovery (FLAIR) sequence is a variant of a T2-weighted sequence in which free water, such as CSF, is suppressed and becomes dark. The FLAIR technique is valuable as it improves the visibility of T2 bright lesions located near bright CSF structures, such as sulci and ventricles. Proton density (PD) sequences display a grayscale that is directly proportional to the concentration of hydrogen nuclei (the higher the number of protons in a given unit of tissue, the brighter the signal). This allows for excellent contrast between the central deep gray nuclei of the brain and brain stem (which have fewer protons and therefore darker signal) and inflammatory lesions containing higher proton content and therefore bright signal. PD sequence images are also particularly valuable in evaluating the cerebellum.
CHARACTERIZATION OF MS ON CONVENTIONAL MRI
The standard MRI protocol for detection of MS lesions and disease activity includes axial T2-weighted images (sometimes including PD images obtained using a dual echo sequence), as well as both axial and sagittal FLAIR images to better identify callosal and pericallosal lesions. Precontrast T1-weighted images (usually axial) identify T1 dark signal intensity lesions or “black holes.” Postcontrast T1-weighted images identify areas of enhancement thought to correspond to areas of active demyelination. MRI protocol has contined to evolve, with the most recent recommendations for a standardized MRI protocol of the brain described in Table 8.1 (7). Note that newer three-dimensional (3D) FLAIR sequences obtained in sagittal plane have near-isotropic voxels that can be reformatted into any plane, including axial. In addition, the utilization of 3D sequences permits quantitative analysis of lesions, with the prospect of automated processing. However, newer sequences may not be available on all scanners. There are differences across different vendors, relative to field strength (i.e., 1.5 vs. 3 T), and with specific sequence parameters. For these reasons, it is best, if possible, to consistently perform follow-up imaging with the same MRI machine and imaging protocol if possible to allow the most accurate comparison.
Central to the diagnosis and management of patients with MS is the concept of lesions disseminated in both space and time. When the first disease-modifying treatments for MS were introduced in the 1990’s, the primary goals of treatment were the reduction of relapse rate and the attenuation of disease progression. Today however, with the availability of new effective MS therapies, the goal of treatment moves toward “no evidence of disease activity” (NEDA) (8). This concept encompasses clinical parameters such as absence of relapse or progression on expanded disability status scale (EDSS), psychological and cognitive factors, with the pertinent imaging data being new T2/FLAIR lesions or postcontrast enhancing lesions. Imaging also allows for the detection of clnically silent lesions.
TABLE 8.1 Revised Standardized MS Protocol Brain MRI Sequences
SEQUENCE | PLANE | FEATURES |
FLAIR | 3D Sagittal | Axial reconstruction: Supratentorial lesions Sagittal reconstruction: Callosal/pericallosal lesions |
T2 | Axial or 3D | Helpful for infratentorial lesions |
DWI | Axial | To assess for PML |
T1 precontrast | Axial or 3D | Black holes; necessary to compare with postcontrast |
T1 postcontrast | Axial or 3D | Active lesions |
PD (optional) SWI (optional) | Axial Axial | Helpful for infratentorial lesions or subtle lesions To identify central vein associated with T2 lesions |
3D, three-dimensional; DWI, diffusion weighted image; FLAIR, fluid-attenuated inversion recovery; PD, proton density; PML, progressive multifocal leukencephalopathy; SWI, susceptibility weighted imaging.
MS lesions can be located anywhere throughout the brain and spinal cord. Since MRI lesions can be nonspecific, or rather that many non-MS forms of neuropathology also produce similar lesions, it is important to classify lesions in terms of their signal characteristics, location, and morphology. Imaging criteria for MS diagnosis using recent guidelines are seen in Table 8.2. Note that older criteria requiring lesion counting are no longer used, and the criteria for DIT and DIS have both been simplified.
TABLE 8.2 2010 International MRI Criteria for Demonstration of DIS
DIS can be demonstrated by one or more T2 lesions in at least two of four areas of the CNS: Periventricular Juxtacortical Infratentorial Spinal cord |
Gadolinium enhancement of lesions is not required for DIS |
If a patient has a brain stem or spinal cord syndrome, the symptomatic lesions are excluded from the criteria and do not contribute to the lesion count. |
CNS, central nervous system; DIS, dissemination in space.
Source: Polman CH, Reingold SC, Banwell B, et al. Diagnostic criteria for multiple sclerosis: 2010 revisions to the McDonald Criteria. Ann Neurol. 2011;69:292–302.
66MRI Signal Characteristics and Morphology
Lesions within the brain are generally small, 5 to 10 mm, with a rounded or ovoid shape. Signal characteristics of the lesions can be divided into early or acute, and late or chronic (see Figure 8.2).
Acute lesions have borders that are somewhat fluffy and ill defined on T2 and FLAIR sequences (Figure 8.3), while chronic lesions have sharply defined borders (Figure 8.4). For both acute and chronic lesions, the signal intensity is bright on T2, FLAIR, and PD sequences. Regarding T1 sequences, acute lesions may be the same or darker than the adjacent normal white matter. Occasionally, hyperacute lesions may even show a thin rim of bright signal on T1 images. Chronic lesions on T1 sequences show well-defined dark regions (so-called “black holes”) with associated volume loss (Figure 8.5), likely representing long-standing disease with axonal destruction, and show some correlation with overall disease progression or disability (9,10).
In the acute setting, an actively demyelinating lesion typically enhances following intravenous contrast administration, reflecting inflammation-related localized breakdown of the blood–brain barrier. Enhancement patterns are often initially solid, subsequently enlarging radially and developing a classic, “incomplete ring” pattern (Figure 8.6), before dissipating after 2 to 6 weeks. The natural time course of enhancement of an acute MS lesion can be rapidly altered with treatment by steroids or immunosuppressants, specifically with the rapid resolution of enhancement, often within days (9). These acute, actively demyelinating lesions are often bright on diffusion weighted images (DWIs), likely reflecting high cellularity due to infiltrative inflammatory cells. Typically, MS lesions show minimal mass effect on the adjacent brain, or at least the degree of mass effect is disproportionately low compared to the size of the lesions, as might be expected for a glioma, for example. Note that large “mass-like” lesions can also occur, although infrequently, with an imaging appearance termed tumefactive MS (Figure 8.7).
Location
The classical pattern of MS disease shows multiple lesions that are bilaterally scattered along periventricular margins, mostly elongated and oriented perpendicular to the ventricles (Figure 8.8). Lesions are commonly located within the corpus callosum or at the “callososeptal junction” along the midline at the septum pelucidum. The elongated periventricular lesions correspond to the classic description of “Dawson’s fingers” representing collections of inflammatory cells clustered around perpendicularly oriented small veins or venules (11). When viewed in the sagittal plain, the characteristic appearance of rounded and ovoid white matter lesions scattered along the ependymal margin or “under-surface” of the corpus callosum and extending into the pericallosal white matter and calloseptal junction has been coined as the “dot-dash” sign, shown to have 90% to 95% sensitivity and specificity for the diagnosis of MS (12,13) (see Figures 8.1 and 8.8). Other common lesion locations in the brain include the juxtacortical white matter, anterior temporal lobes, brachium pontis, and cerebellum (Figure 8.8).
Although the typical MS patient will have relatively fewer lesions in the spinal cord compared to the brain, likely reflecting the relatively large volume of the brain compared to the cord volume, spinal cord lesions are usually more symptomatic. In fact, as many brain lesions can be clinically silent, cord lesions often cause the initial symptoms that persuade a patient to seek medical care. Thus, any cervical MRI demonstrating a spinal cord lesion with features suspicious for MS should prompt follow-up imaging of the brain. As in the brain, these lesions are bright on T2 or PD images, often located in the periphery of the cord, are generally less than two vertebral body segments in length, and encompass less than one-half of the cross-sectional area of the cord (Figure 8.8I). Also similar to the brain, acute or actively demyelinating lesions typically show enhancement following contrast administration. In addition, acute cord lesions can be mildly to moderately expansile, enlarging the overall diameter of the spinal cord at that level. In distinction, chronic cord lesions are associated with focal volume loss, or myelomalacia. This may impart a scalloped shape to the surface of the cord visible on sagittal images. In addition, cord lesions not uncommonly disappear entirely on imaging after months or years.
DISSEMINATION IN SPACE
Dissemination of lesions in space can be demonstrated on MRI by identifying one or more T2 bright lesions in at least two of four locations considered characteristic for MS: periventricular white matter, juxtacortical white matter, posterior fossa, or in the spinal cord (5) (see Figure 8.8).
DISSEMINATION IN TIME
DIT can be demonstrated in its simplest form by identifying new lesions on consecutive follow-up MRI (see Figure 8.9). Fulfillment of DIT on a single MRI examination is the simultaneous presence of lesions of different ages. Specifically, the criterion requires the presence of an asymptomatic gadolinium-enhancing (or lesions with an acute pattern of imaging characteristics) and nonenhancing lesions (or lesions with chronic imaging pattern) simultaneously on a single MRI study in a patient with typical clinical presentation, provided there is no non-MS pathology (5,14,15).
With progression of disease, there is an accumulation and coalescence of chronic lesions, many containing frank axonal destruction. Long-standing MS patients typically demonstrate a pattern of brain parenchymal volume loss that bears the stigmata of recurrent inflammatory damage. For example, given the preferential distribution of MS lesions throughout the corpus callosum and callososeptal junction, chronic MS patients eventually develop striking callosal volume loss. Deep white matter lesions and periventricular lesions lead to marked enlargement of the ventricles (see Figures 8.5, 8.10, and 8.11). The degenerative phase of MS leads to cortical volume loss with apparent widening of the sulci.
67
FIGURE 8.1 Example of typical MS protocol. Axial FLAIR (A) and sagittal FLAIR (B) images both demonstrate callosal and pericallosal lesions with a perpendicular orientation along the callososeptal margin more conspicuous on the sagittal plane image (B). Axial T2-weighted image (C) demonstrates additional periventricular and deep white matter lesions in both hemispheres—slightly less obvious than on the FLAIR. Bottom row: axial PD image (D) is useful to identify central deep white matter lesions. Axial precontrast T1-weighted (E) and axial postcontrast T1-weighted (F) images are used to identify areas of enhancement correlating to areas of active demyelination.
FLAIR, fluid-attenuated inversion recovery; MS, multiple sclerosis; PD, proton density.
FIGURE 8.2 Time course of MRI change in MS lesion. Note early development of contrast enhancement and T2-weighted change and later development of T1 “black hole” changes.
MS, multiple sclerosis.
Variations in Appearance and Potential Look-Alikes
Like many tissues, when subjected to pathological insult, the brain can only respond macroscopically in several ways. Since MRI typically images a tissue’s macroscopic response rather than the pathology itself, many different pathologies or diseases can have a similar appearance. Specifically, MRI exquisitely measures water concentration within tissues, which can be altered in two ways: (a) vasogenic edema which allows excess water to enter the brain parenchyma from leaky vessels, or (b) the destruction or alteration of myelin (which is hydrophobic) which allows excess water to enter the now more-hydrophilic lesion. Thus, although extremely sensitive in the detection of white matter pathology, unfortunately many MRI abnormalities common in MS are nonspecific and can be seen in a variety of other disease processes, which are difficult to distinguish by imaging alone (see Figure 8.7). An additional difficulty is that the number, size, and overall burden of brain and spinal cord disease can vary dramatically depending on the stage at which an MS patient is imaged and the severity of their disease.
68
FIGURE 8.3 A 39-year-old woman with moderate disease burden MS. Axial FLAIR image (A) shows numerous patchy foci of FLAIR bright signal throughout the deep periventricular and pericallosal white matter of both hemispheres. The largest lesion in the left frontal lobe shows relatively fluffy, ill-defined margins on the FLAIR image (arrow, A), corresponding dark signal on precontrast T1 image (arrow, B), and avid enhancement following contrast administration (arrow, C) consistent with a new or acute lesion with active demyelination.
FLAIR, fluid-attenuated inversion recovery; MS, multiple sclerosis.
FIGURE 8.4 Coronal FLAIR (A) and axial T1 (B) images in this patient with known, long-standing MS show bilateral deep white matter lesions with sharp, well-defined margins and corresponding profoundly dark T1 signal consistent with late or chronic lesions. These T1 dark foci are commonly referred to as “black holes.”
FLAIR, fluid-attenuated inversion recovery; MS, multiple sclerosis.
NONSPECIFIC WHITE MATTER DISEASE
The most common differential diagnostic consideration for multiple nonenhancing foci of bright T2 or FLAIR signal in the supratentorial white matter without associated mass effect is nonspecific white matter disease (NSWMD), which are multiple small foci of white matter injury thought secondary to the sequelae of chronic, clinically silent, microvascular ischemia. These white matter changes are often associated with generalized, diffuse brain parenchymal volume loss frequently noted in patients over 50 years old (16), and can be accentuated in patients with chronic hypertension or diabetes. An example of nonspecific, likely chronic microvascular ischemic changes is included in Figure 8.7.
69
FIGURE 8.5 Upper row axial FLAIR (A) and sagittal FLAIR (B) images from a middle-aged woman with advanced MS demonstrate severe burden, patchy and near confluent bright signal abnormality throughout the periventricular and subcortical white matter with marked associated thinning of the corpus callosum (arrows, B) and marked generalized brain parenchymal volume loss greater than expected for age. Axial FLAIR (C) and sagittal FLAIR (D) images from a young, newly diagnosed patient with MS demonstrate relatively fewer white matter lesions with volume of the corpus callosum and overall brain parenchymal volume intact.
FLAIR, fluid-attenuated inversion recovery; MS, multiple sclerosis.
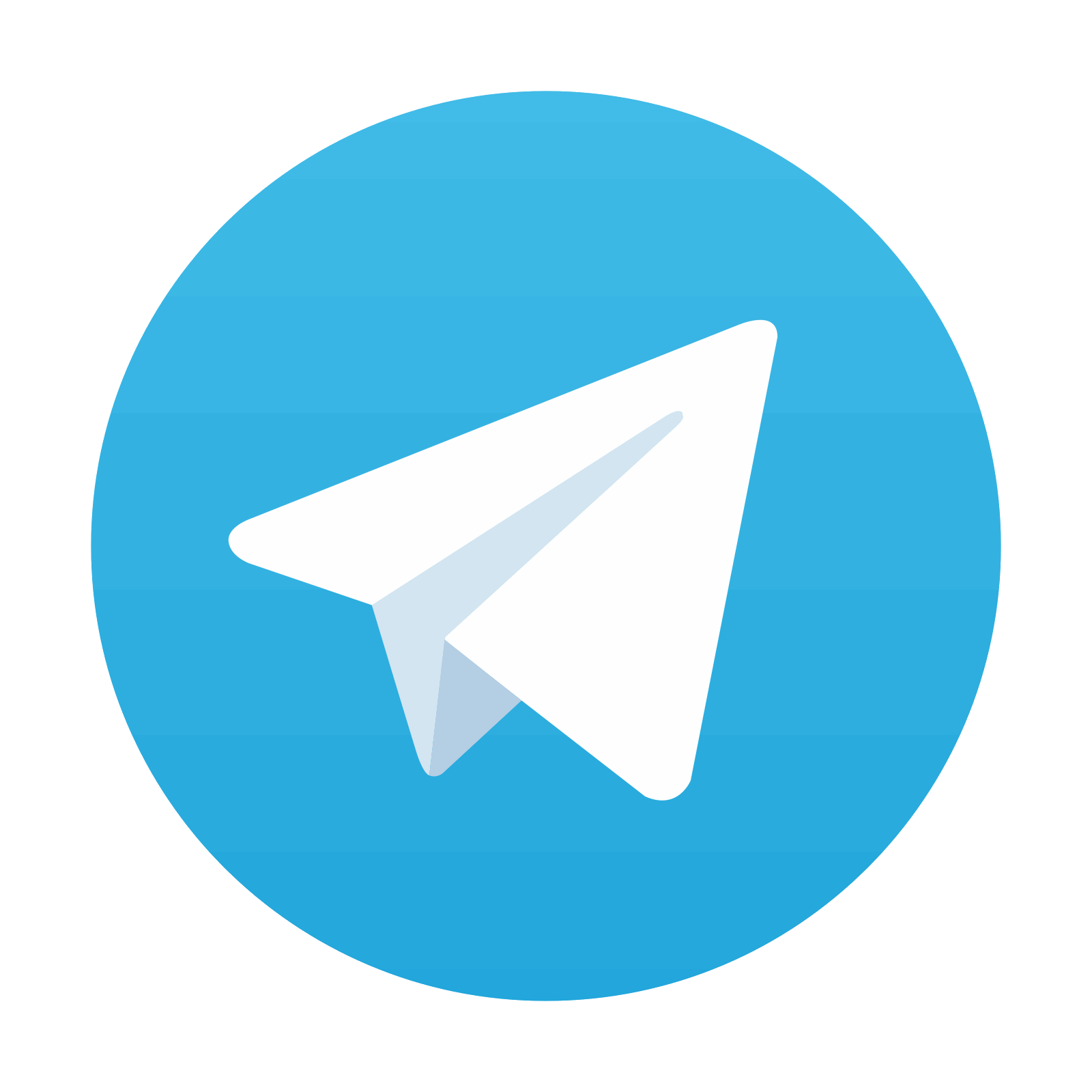
Stay updated, free articles. Join our Telegram channel

Full access? Get Clinical Tree
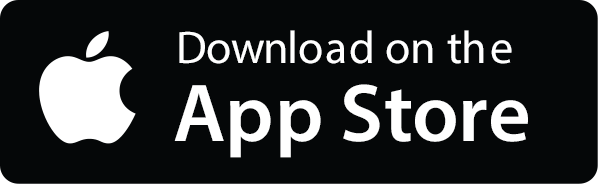
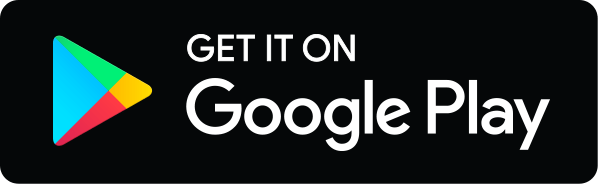