11
Lumbar syndrome
Definition and Prevalence
The term “lumbar syndrome” refers to disease manifestations caused by functional disturbances and degenerative changes of the lumbar motion segments.
These include local symptoms restricted to the lumbar spine (local lumbar syndrome) as well as pain radiating into the leg (lumbar root syndrome) and the symptoms and signs of deep, functionally transecting processes (cauda equina syndrome). The first two types of lumbar syndrome are very common.
Nearly two-thirds of all cases of disk disease affect the lumbar spine.
One in 12 patients presenting to a general practitioner, and every third patient presenting to an orthopedic surgeon, seeks medical attention because of a lumbar syndrome. These figures include only the patients who need treatment. Not all patients with sciatica or exercise-related low back pain go to a physician. The overall prevalence of low back pain, and its relative prevalence as a fraction of all types of illness, are thus presumably much higher than these figures indicate.
Age and sex distribution. In general, lumbar spinal processes affect men somewhat more often than women. The predominance of the male sex is attributable not only to a greater degree of functional mechanical stress, but also to sex-specific factors that are not yet well understood.
Lumbar syndrome is not only an important medical entity but also has a considerable effect on society at large, both because of its high frequency and because it usually arises in middle age and thus strikes patients at the high point of their occupational productivity. The symptoms most often arise in the patient’s late twenties and reach their highest age-related prevalence around age 40 (Fig. 11.1). This is also the age at which most operations for lumbar disk prolapses are required. As will be discussed in Chapter 12, the disks have a special biomechanical constellation at this age, in which the nucleus pulposus still has a high turgor pressure, but the mechanical resistance of the anulus fibrosus has already begun to decline. Because of these properties, centrally located disk tissue can become displaced outward. Further information on the point prevalence and the annual and lifetime prevalence of back pain (which can largely be equated with the epidemiology of lumbar syndromes) can be found in Chapter 3.
Etiology. The regularly observed statistical features of lumbar disk syndromes are few. Acute low back pain and sciatica often seems to develop spontaneously, without any recognizable external cause. The relationship between lumbar syndrome and heavy or light physical labor is discussed in Chapter 17. The incidence of certain other types of illness (e.g., ulcer disease) is known to vary seasonally; the incidence of lumbar syndrome is somewhat lower in the spring, but relatively constant at other times of the year.
Symptoms arise largely because of the biochemical and biomechanical processes that occur autonomously within the disk tissue as part of the involutional process of normal aging.
Fig. 11.1 The distribution by age and sex of outpatients treated for lumbar syndrome in Düsseldorf and Bochum (North Rhine-Westphalia, Germany) in comparison with the age distribution of the overall female and male population in the German state of North Rhine–Westphalia.
Special Anatomy of the Lumbar Motion Segments
Lumbar Disks
The lumbar spine generally has five freely mobile lumbar vertebral bodies, four lumbar intervertebral disks, and one disk each at the thoracolumbar and lumbosacral junctions. The lumbar disks increase in size from cranial to caudal, with the exception of the lowest (lumbosacral) intervertebral disk, which is about one-third thinner than the next disk above it. The biconvex shape of the disks is at its most pronounced in the lumbar region of the spine. The disks are higher ventrally than dorsally to an extent that depends on the degree of the lumbar lordosis. This ventral-to-dorsal difference is greatest in the lumbosacral (L5/S1) intervertebral disk, whose cross-section on a lateral image is trapezoidal in shape (Fig. 11.2).
Intervertebral Foramina
The anatomical relationships of the intervertebral disks and intervertebral foramina with the corresponding spinal nerve roots are of particular importance in the lumbar spine. As shown in Fig. 11.3, the intervertebral foramina are found at the level of the disk. The spinal ganglia and ventral roots lie more ventrally than in the thoracic region and are thus immediately adjacent to the disk. The bony border of the intervertebral foramen formed by the dorsolateral edge of the vertebra becomes more prominent superiorly, particularly in the upper portion of the intervertebral foramen, through which the nerve root travels. The caliber of the lumbar nerve roots increases as one moves caudally, with the L5 nerve roots being the thickest—approximately five times thicker than the L1 roots.
The intervertebral foramina of the lumbar spine are dorsally delimited by the facets of the intervertebral joints. The joint spaces of the L1–L4 joints are sagittally oriented, but the articular surfaces at the lumbosacral junction lie in the frontal plane, as in the thoracic spine. As a result, the lumbosacral intervertebral foramen is smaller than the rest. Variations and differences between the two sides are common. Changes at the joint facets and altered positions of the intervertebral joints can narrow the intervertebral foramen from its dorsal aspect.
All of these anatomical properties create unfavorable initial conditions for the nerve roots of the lower lumbar motion segments. The roots are at risk of mechanical compression if the disk contour changes or if the vertebral bodies shift in position through slackening of the disks.
An increasing degree of importance is currently being ascribed to the width of the lumbar spinal canal in the frontal and sagittal planes as a contributing factor in the generation of low back pain and nerve root irritation. If the lumbar spinal canal is narrow, either congenitally or for an acquired cause, even a mild alteration of the contour of the intervertebral disks dorsally delimiting the canal can produce symptoms.
Fig. 11.3 a–c Lateral sagittal section through the lumbar spine.
a, b The intervertebral foramina are located at the level of the intervertebral disks.
c MRI: in the cranial portion of each intervertebral foramen, the exiting spinal nerve root can be seen in cross-section, appearing as a round dot.
Ventral Epidural Space
The ventral epidural space is located between the posterior edge of the vertebral body and the dorsal border of the disk (forming the anterior border of the space) and the ventral dura mater (forming its posterior border). This space is divided into right and left compartments by connective tissue strands running between the dura mater and the vertebral body in the midline (Hofmann 1898). These midline connective tissue strands attached to the dural sac (also called the medial dural suspension) prevent the movement of large free disk fragments across the midline to the opposite side.
The ventral epidural space of the lumbar region, lying between the posterior edge of the vertebral body and the ventral dura mater, contains a thin peridural membrane that has veins running within it (Fick 1904, Dommisse 1974, Schellinger et al. 1990, Wiltse et al. 1993, Ludwig 2004). Extradiscal disk fragments (sequestra) can be displaced under this membrane; in such cases, discography reveals contrast medium occupying a round area in the epidural space, either cranial or caudal to the level of the disk, without any other epidural contrast medium. The MRI appearance is of a round shadow covered with a membrane. At operation, the surgeon must open this membrane to gain access to the disk fragment (Fig. 11.4).
The medial dural suspension and the ventral epidural membrane determine the direction of displacement of lumbar disk prolapses as well as their clinical course and prognosis.
The space between the ventral epidural membrane and the posterior edge of the vertebral body is delimited medially by the relatively tightly adjacent posterior longitudinal ligament and laterally at infradiscal levels by the pedicle. At supradiscal levels its lateral boundary is formed by the adherence of the connective tissue layer to the vertebral body.
Submembranous disk fragments lie paramedially.
Hematomas can collect in the ventral epidural space and can be confused with submembranous disk fragments (Wiltse et al. 1993) (Figs. 11.4, 11.5).
Fig. 11.4 A caudally dislocated, submembranous disk fragment (dislocation grade III) under the ventral epidural membrane (→).
Fig. 11.5 Myelodiscography (i.e., myelography immediately followed by discography) at L5/S1. The discogram reveals a cranially displaced, submembranous fragment (dislocation grade III) that is still covered by a thin layer of tissue, the ventral epidural membrane. At surgery, a beansized fragment was found compressing the shoulder of the S1 root under the ventral epidural membrane. The membrane was easily perforated with a blunt dissector.
Ligamentum Flavum
The ligamentum flavum (yellow ligament) is an important structure in disk surgery. It lines the posterior aspect of the spinal canal and runs from one vertebral arch to the next, spanning the intervertebral spaces. At surgery, it is easily separated from the vertebral arch and can then serve as an additional protective layer above the dura mater during a laminectomy or hemilaminectomy.
In the lumbar region, the ligamentum flavum has two layers (ventral and dorsal), which are separated by a cleft that can be demonstrated either during an anatomical dissection or at surgery. In a lateral (partial) flavectomy, the surgeon divides the dorsal layer of the ligamentum flavum sharply (i.e., with a scalpel) and the ventral layer bluntly (with a dissector) in order not to injure the dura mater, which lies beneath (Grifka et al. 1997, 1999).
The ligamentum flavum increases continually in thickness from the cervical spinal canal caudally. The ligamentum flavum and the interspinous ligaments help maintain the continuity of the posterior portions of the motion segment when the individual bends forward in maximum kyphosis or maintains an erect posture.
Venous System
The vessels of the vertebral venous system can also play a role in the generation or worsening of disk-related symptoms. The valveless veins of the spinal canal form an uninterrupted anastomotic chain running from the skull base to the sacrum. The degree of filling of the veins depends on the position of the body. The veins are tautly distended if the individual sits or lies prone. As our intraoperative measurements have shown, the degree of filling of the lumbar epidural veins depends on the central venous pressure (Ghazwinian and Krämer 1974). The degree of filling and the central venous pressure are lowest in the kneeling position. During lumbar disk surgery, the epidural venous plexus appears as a collection of flat strands containing only a small amount of blood; as a result, electrocoagulation is usually not necessary for hemostasis within the spinal canal. The studies of Clemens (1970) and Crock (1983, 1994, 2002) showed that the vertebral venous system not only plays a local role as the draining vasculature of the vertebral arches and the spinous and transverse processes, but also communicates with the interior of the skull by way of the emissary veins. The venous plexuses of the spine are also a venous pathway connecting the superior and inferior venae cavae. Together with the azygos system, they form a collateral venous circulation that operates beyond the local level, coming into play physiologically whenever the venous pressure is elevated in the thoracic, abdominal, or intracranial cavities—as when the individual coughs, sneezes, or presses. This partly explains why disk-related symptoms often worsen when these events occur.
Elevations of pressure in the chest and abdomen worsen disk-related pain because they increase the degree of filling of the epidural veins.
Numerical Variations and Transitional Vertebrae
The clinical importance of malformations and developmental anomalies of the lumbar spine and their causative role in disk-related symptoms are generally overstated. Numerical variations are of practical clinical importance, e.g., in the designation and identification of segments. The number of ribless lumbar vertebrae above the sacrum should be counted. If there are only four, the term “sacralization” is often used; if there are six, “lumbarization.” It is clear, however, that sacralization or lumbarization can only be determined from the enumeration of all vertebrae in a complete radiological image series. It is therefore preferable to avoid these terms and use only the designation “transitional vertebra.” The numbering of the spinal nerve roots in numerical variations of the lumbar vertebrae is shown in Fig. 11.6. In such cases, one should count downward from L1—the first ribless vertebra—rather than upward from the sacrum. For example, if there are six free lumbar vertebral bodies, a disk prolapse between L5 and L6 produces an L1 syndrome. On the other hand, if there are only four free lumbar vertebrae, then a prolapse between the fourth vertebra and the sacrum usually produces an L5 syndrome.
One should also be aware that rudimentary disks can appear differently in MRI and in plain radiographs.
Transitional vertebrae have characteristics of both of the neighboring regions. Lumbosacral transitional vertebrae may have free transverse processes, or they may be connected to the sacrum either rigidly or through joints. The intervertebral disk can take on many different transitional forms (Fig. 11.7).
As long as the transitional vertebra is symmetrical, having the same types of structures on both sides, no symptoms are to be expected. Abnormal mechanical statics of the spine arise only when the transitional vertebra is asymmetrical, e.g., when a free transverse process is present on one side and a rigid or articular connection to the sacrum is present on the other (Fig. 11.7). Asymmetrically shaped lateral masses of transitional vertebrae that have lumbosacral joints on both sides can also produce tilting of the transitional vertebra, resulting in a low-lying lumbar scoliosis. In such cases, the disks at higher levels are subject to an asymmetrical mechanical stress, by which they may become pathologically affected.
Fig. 11.7 Asymmetrical lumbosacral transitional vertebra in a 15-year-old girl with lumbar scoliosis.
Topographical Relationships Between the Spinal Nerve Roots and Disks in the Lumbar Spinal Canal
The fact that most cases of lumbar root syndrome arise in the two lowest motion segments is due both to the high degree of mechanical stress affecting this region of the spine and to the close contact between the nerve roots and the disks at these levels. In the cervical spine, the clinically relevant degenerative processes take place mainly outside the spinal canal, but in the lumbar spine they much more frequently narrow the lumen of the spinal canal through dorsal disk protrusions and prolapses. The lumbar spinal canal is delimited ventrally by the vertebral bodies and disks and dorsally by the ligamentum flavum and vertebral arches (laminae). The lateral (articular) masses and intervertebral foramina are located laterally. The spinal canal is a cylinder whose shape and volume change with every movement of the trunk.
The contents of the lumbar spinal canal include the dural sac, the nerve roots, and the peridural tissues, which in turn consist of the veins and fat that surround the nerve roots. The nerve roots are thus protected from the bony borders of the spinal canal even in extreme movements of the lumbar spine.
The distance between spinal cord segments and their corresponding motion segments in the vertebral column is greatest in the lumbar spine. Because the spinal cord terminates at L1/L2, the spinal nerves, which exit the spinal canal at more caudal levels by way of their correspondingly numbered intervertebral foramina, must travel a considerable distance in the subarachnoid space. At levels below the conus medullaris, the nerve roots are mainly located laterally, so that they are generally not injured in the course of a medial lumbar puncture, myelography, or transdural disk puncture.
Paramedian disk protrusions and prolapses can compress spinal nerves intrathecally and produce nerve root syndromes at lower levels than the level of the disk itself.
The direction in which the nerve roots run after exiting the dural sac depends on the level of the segment. The further caudally the nerve root must travel to reach the corresponding intervertebral foramen, the more acute the angle it makes with the dural sac as it exits from it. It follows that the topographical relationship between the nerve root and the disk is different in each lumbar motion segment (Fig. 11.8). The origin of the L4 nerve root is found at the level of the L3 vertebral body. The L5 root, however, exits the dural sac at the level of the lower edge of the L4 vertebral body, and the S1 root at the lower edge of the L5 vertebral body. An L4/L5 disk prolapse generally impinges on the L5 nerve root. The L4 root is affected only if the prolapse is very large and laterally displaced, because the L4 root runs above the level of the intervertebral disk.
A lateral prolapse of the L5/S1 intervertebral disk can simultaneously impinge on the L5 and S1 nerve roots even if the prolapse is small. The L5 nerve root, as it passes through the upper portion of the L5/S1 intervertebral foramen, is immediately adjacent to the outer lamellae of the intervertebral disk. The root has very little freedom to move within the foramen. Only the lowest two lumbar nerve roots, which lie in the lowest two lumbar motion segments, are in direct contact with the corresponding intervertebral disks. It is here that the danger of compression by disk tissue is greatest.
Fig. 11.8 a, b The lumbosacral dura mater and nerve roots and their topographical relationship to the disks.
a Schematic diagram.
b Dissection of the lumbosacral spine of a 32-year-old woman. The laminae have been removed as far laterally as possible (to the lateral masses).
Radiological/Surgical Subdivisions of the Lumbar Motion Segments
A topographical anatomical subdivision of the lumbar motion segments that takes the spatial relationships of the spinal nerves, disk, pedicles, and intervertebral foramina into account is a useful conceptual aid for the proper identification of anatomical structures and their pathological alterations in plain radiographs and CT and MR images, as well as for orientation in the operative field. The prognosis and clinical course of sciatica, too, depend not only on the size of a disk prolapse but also on its position in relation to its anatomical surroundings. Such spatial relationships are best assessed not just in the three conventional radiological anatomical planes—anteroposterior (frontal), lateral (sagittal), and transverse (horizontal, axial)—but also in a surgical anatomical perspective, with an oblique AP view of the spine. McCulloch (1989, 1998) analogized the motion segment to a three-storey house with the level of the disk constituting the first (lowest/most caudal), the infrapedicular level the second, and the level of the pedicles the third (highest/most cranial) storey. Wiltse et al. (1992) added a suprapedicular level to this subdivision. A subdivision with reference to the disk, rather than the pedicles, seems more practical, as the pedicles are not always precisely localizable in the operative field (Krämer 1995, Willburger et al. 2005).
Frontal View (AP Projection)
The AP projection provides the best topographical overview of a lumbar motion segment. It also corresponds to the surgeon’s view, albeit rotated by 90°. The successive transverse anatomical planes, from cranial to caudal, are called levels, and the successive sagittal planes, from medial to lateral, are called zones (Fig. 11.9).
Levels. The levels are defined from caudal to cranial in relation to the borders of the intervertebral space and the pedicles on the anterior wall of the spinal canal. Practically speaking, the intervertebral disk is the main orienting structure in radiology and disk surgery, because it is easy to identify both on radiological images and in the operative field.
The discal level is delimited by the upper end plate of the vertebral body below the disk in question and by the lower end plate of the vertebral body above it.
The supradiscal level is above the discal level and extends upward to the lower edge of the pedicles, i.e., to approximately the level of the middle of the upper vertebral body.
The infradiscal level is below the discal level and extends downward to the lower edge of the pedicles of the lower vertebral body.
A cranially dislocated fragment is supradiscal, and a caudally dislocated one is infradiscal.
Zones. The division of a lumbar segment as seen on a transverse radiological image (CT, MRI) into successive sagittal zones, from medial to lateral, differs depending on the craniocaudal level. At the discal level, the segment is divided into medial, paramedial, and lateral zones, without any further landmarks for orientation.
The medial zone has a right and a left half. The center of the paramedial zone corresponds to the central point of the interlaminar operative field after removal of the ligamentum flavum. All pathological findings in the immediate vicinity are described as paramedial, those nearer to the midline are medial and those further from the midline are lateral. The transition from the paramedial to the lateral zone is indicated by the medial border of the pedicles of the vertebral bodies above and below.
The same division is operative at supra- and infradiscal levels. The medial and paramedial parts of the vertebra lie between the pedicles, and the lateral part of the vertebra is the part lying lateral to the medial border of the pedicle. Thus, the pedicle itself and the infrapedicular zone corresponding to the upper portion of the intervertebral foramen lie in the lateral zone of the vertebra.
A disk herniation cannot lie in the lateral zone at the infradiscal level, because the lateral zone is occupied here by the pedicle. Lateral disk herniations at discal and supradiscal levels are located within the intervertebral foramen.
Frontal aspect, with roots. Myelography displays the dural sac and the roots exiting from it in a frontal view, projected over the vertebral bodies and disks. A similar view can be obtained by MRI. These views correspond to the view into the operative field at surgery.
In the lower lumbar motion segments, each root traverses an intervertebral disk before exiting the spinal canal through the intervertebral foramen under the pedicle of the segment below. Each lumbar interlaminar window contains two roots (Fig. 11.10):
The traversing root lies in the paramedial zone at the discal level and is usually affected by paramedial disk prolapses and protrusions. The exiting root is found laterally, above (cranial to) the disk.
The exiting nerve root is derived from the segment above and exits the spinal canal under the correspondingly numbered pedicle. Cranially dislocated prolapses can compress the exiting root at the supradiscal (infrapedicular) level intraforaminally (i.e., in the lateral zone).
Extraforaminal prolapses are found further laterally at the discal and supradiscal levels.
Frontal view of the operative field. In a lumbar disk operation, the spine is seen lying transversely in front of the surgeon. After removing the ligamentum flavum laterally in the interlaminar window at either the L4/5 or the L5/S1 level, the surgeon encounters the traversing root and the paramedial zone of the intervertebral disk lying under it.
The exiting nerve root on either side lies craniolateral to the disk in the upper portion of the intervertebral foramen. This is where to look for cranially dislocated intraforaminal disk fragments; the intervertebral joint facets lying just above should be left undisturbed as much as possible. Disk fragments can migrate in practically any direction from the site where they perforate the anulus fibrosus in the paramedial zone of the disk.
The exiting root always lies craniolateral to the disk.
For the purposes of description and documentation of radiological and intraoperative findings, not only the cardinal directions cranial, caudal, medial, and lateral but also the intermediate directions craniomedial, craniolateral, caudomedial, and caudolateral sheould be used. Directions can also be designated using clockface notation (Fig. 11.11). In an operative report, not only the direction in which the disk fragment is located, but also its distance from the site of perforation should be mentioned.
Fig. 11.11 a, b Localization of a free fragment in the spinal canal. The reference point is the center of the paramedial zone on the level of the disk.
Most disk prolapses that require surgery migrate caudally (to the 9 o’clock position on the right side, or the 3 o’clock position on the left).
Even before surgery on a lumbar disk prolapse, the surgeon should know in which direction the spinal canal must be explored to find the fragment, to avoid opening the spinal canal any more widely than necessary. This important information is already visible on the CT or MRI scan.
Fig. 11.12 Craniolateral displacement with compression of the exiting nerve root. Disk fragments lying at the level of the disk or displaced caudally to it impinge on the traversing nerve roots rather than the root that exits at that level.
Lateral View
Sagittal MRI sections give the best view of prolapsed disk tissue. The tissue may lie at the level of the disk, or else it may be cranially or caudally dislocated to a greater or lesser extent or lie behind the vertebral body. It is usually possible to tell from which disk the fragment originated (the “donor disk” or disk of origin). One can also determine the position of the disk fragment along the medial-to-lateral axis by viewing sagittal sections taken in different planes. Laterally and craniolaterally dislocated fragments lie within the foramen and compress the exiting root. Fragments that are dislocated in any other direction compress the traversing root (Fig. 11.12).
The lateral (sagittal) view is useful not only for prolapse localization, but also for determination of the segment. The radiographic image that is normally taken intraoperatively with a needle lying between two spinous processes must be correlated with the preoperatively obtained MR image showing the prolapse in order to confirm that surgery is being carried out at the right level. Rudimentary and transitional disks look different in MRI than in a conventional lateral radiograph. Errors in counting can be avoided by using terms such as the “last” or “second last” disk, rather than “L5/S1,” etc.
Overlapping laminae. The laminae cover the discal level to an extent that increases as one goes up the spine. This fact must be borne in mind when operating on the lumbar spine via an interlaminar approach, with a view of the spine from the side. Thus, while flavectomy at the L5/S1 level immediately exposes the level of the disk. Flavectomy exposes only the infradiscal level at L4/5 and even more so at L3/4, etc., so that that the discal level comes into view only after removal of the lower portion of the upper lamina (Fig. 11.13).
Cranially dislocated prolapses therefore often require removal of some of the lower portion of the upper lamina, or perhaps all of it (hemilaminectomy).
Fig. 11.13 a, b A schematic view of the operative field in the interlaminar window at each of the lower lumbar motion segments. The level of the disk space is located between the laminae only in the L5/S1 segment. In successively higher segments, the lower edge of the upper lamina overlaps to an increasing extent with the level of the disk space.
Transverse View
Transverse (axial, horizontal) sections of the lumbar motion segments are obtained by CT or MRI (Fig. 11.14). One can determine the level of any section from the sequential alternation of disks and vertebral bodies (or of pedicles and intervertebral foramina) across the series of transverse sections; reference to a lateral scout view (“topogram”) is thus not necessary. The remaining posterior elements—joints, spinous processes, and transverse processes—have highly variable shapes and add nothing to segment localization except confusion. The two sides of a transverse section look different in the presence of scoliosis or if the section is improperly aligned (i.e., not really transverse).
A transverse section at the discal level displays the disk, possibly accompanied by partially sectioned portions of the upper and lower vertebral body end plates, as well as the intervertebral foramen. At the supradiscal level, the vertebral body and foramen are seen next to each other; an infradiscal section shows the vertebral body and the pedicle, but not the foramen.
At the infradiscal level, one sees the vertebral body and its continuous extension in the posterior arch (the pedicles and lamina). The lateral recess is found at the infradiscal level at the same position where one finds the intervertebral foramen at the discal and supradiscal levels. The lateral recess contains the traversing root next to the correspondingly numbered pedicle, e.g., the lateral recess of L5 contains the L5 root.
The division of the transverse sections at different levels into zones is the same as that described above for the frontal view (Fig. 11.15). The center of the paramedial zone is located between the midline and the lateral border of the disk and usually corresponds to the center of the operative field in the interlaminar approach. The medial edge of the pedicle, as seen in an infradiscal section (which contains the pedicle), defines the border of the lateral zone. Medial prolapses and protrusions can be more pronounced on either the right or the left side.
Fig. 11.14 Schematic view of a lumbar vertebral body and disk seen transversely, as in a CT or MRI scan.
Special Biomechanics of the Lumbar Spine
(H.J. Wilke)
Mechanical Stress on the Lumbar Disks
Beyond the general biomechanical properties of motion segments that were discussed earlier in Chapter 4, the lumbar spine has a number of special properties that are relevant to the causation of disk disease and to its prevention.
The human upright stance puts great stress on the lower spinal segments in particular. At these levels, the weight of the entire body above must be carried on a surface with an area of only a few square centimeters. Moreover, when the upper body deviates from the mid-line, the mechanical stress is multiplied many times over.
There are many ways of measuring the mechanical stress on the spine in different postures and when an individual lifts or carries heavy loads. Quantification is rendered difficult by the general impossibility of putting a measuring instrument directly into the spine in a living individual. Usually, therefore, we must resort to theoretical models or indirect measurements for most information of this type. Analytical models are used to determine the overall stress on the spine by calculating all of the individual forces that are placed upon it (Fig. 11.16). Stress on the spine is a complex matter, as the spine does diverse tasks and has a complicated mechanical structure. Muscles must exert force to counteract the forces acting upon the spine and keep the body mechanically stable.
Fig. 11.16 Analytical models: forces determining the mechanical stress on the spine (including the force F resulting from the abdominal pressure P and the resulting reactive forces) (from Wilke 2004).
These forces are the result of a complex interaction of many different muscle groups and individual muscle fibers acting in response to body position, movement, or the externally applied forces mentioned above. The degree of mechanical stress on the spine varies highly among individuals, depending on age, sex, stature, and physical condition. Further forces are said to be exerted on the spine by intra-abdominal pressure and muscle contraction (Grew 1980, Hemborg and Moritz 1980, Nachemson et al. 1986), yet the magnitude of such forces is unknown, nor is there even any consensus regarding their sign, i.e., whether they tend to compress or to distract the spine. It can be assumed that the complex structure of the normal spine is capable of providing adequate stability to the individual motion segments and to the spine as a functional unit, and thus of fulfilling its main tasks optimally, under physiological conditions. Injury, disease, or degeneration of part of the spine can, however, impair its stability.
The following sections outline the main methods of determining the stresses affecting the spine and present their advantages and disadvantages.
Methods of Measuring Stress on the Lumbar Motion Segments
Mathematical Models
Theoretical models provide a simple, analytic approach toward an initial rough estimation of the biomechanical condition of the spine under static or dynamic stress (Schultz and Anderson 1981, McGill 1992). Extensive calculations have been carried out using computer simulation models (Chaffin 1969, 1988; Nussbaum and Chaffin 1996, Parniapour et al. 1997). Finite element models can be used to obtain further information on specific parameters such as deformation, stretching or pressure in individual components of the motion segment (Shirazi 1991, Goel et al. 1993, Wang et al. 2000, Zander et al. 2001) (Fig. 11.17).
The complex overall system, with all of its individual components, can be modeled only in a simplified fashion because of the limitations of computing capacity. The input parameters of computational models must include anatomical, biomechanical, and biological data and materials constants; not all of these parameters can be adequately measured or estimated by other methods, and therefore not all of the inputs to computational models can be considered reliable. The validity of such models, then, is limited by the simplifications and assumptions that they incorporate. The results of computation should always be checked against experimentally measured findings (Fig. 11.18).
Fig. 11.17 An illustration of a finite-element calculation from our own research group. Left: complete model of an L4/L5 spinal segment, posterolateral view. Right: midsagittal section through the anulus. Comparison of the degrees of stretch in flexion and extension (from Wilke 2004).
Fig. 11.18 Mathematical models such as the 3-D static model of Chaffin et al. at the University of Michigan (left) can be checked against disk pressure measurements obtained in vivo in certain body positions (right) (from Wilke 2004).
In-Vitro Studies
In-vitro studies on anatomical specimens offer the opportunity to place measuring devices directly on to or into real structural components of the spine. Components such as the disk, the ligaments, or the vertebral body itself can be studied in isolation or as a functional unit. Thus, for example, the biophysical properties of a component of the spine can be tested in vitro to determine the maximum stress that it can tolerate. Moreover, special experimental apparatus can be used for the in-vitro study of the behavior of spinal component structures under well-defined conditions of stress. For example, the intradiscal pressure, stretching of ligaments, stress on an artificial implant, or movement of individual segments can be analyzed (Wilke et al. 1994, 1995, 1996).
In-Vivo Studies
In-vivo studies are done under much more realistic conditions, but they are feasible only to a limited extent, not just because of the greater technical difficulty of making measurements in the living organism, but above all for ethical reasons. In-vivo measurements in animals are of questionable applicability to humans, because the stresses on the spine in quadrupeds and bipeds are markedly different.
Electromyography (EMG) provides information on individual muscle activity and also, if the experimental setup is held constant from muscle to muscle, a rough picture of the distribution of activity among different muscles. The surface electrodes that are usually used can yield no information about deeper muscles. Thin needle electrodes inserted deeply into the muscle yield direct information about the local situation at the site of the needle tip; thus, these electrodes, though much less commonly used in practice, are also much more informative. Andersson et al. (1974) reported the findings of intradiscal pressure measurements, some of which were made simultaneously with EMG. Unfortunately, it remains impossible to infer absolute muscle strength from the EMG data alone. This task requires more complex optimization algorithms, whose validity is still a matter of hypothesis (Parniapour et al. 1997). In any case, it has been conclusively shown with this method that a kyphotic sitting position requires less muscle activity then a consciously effected lordosis (Dolan et al. 1988, Betz et al. 2001).
Measurements with Instrumented Implants
Biomechanical measurements can be made indirectly in vivo with the aid of measuring devices located in implanted material, such as the implants used in fusion operations on the lower lumbar spine. These implants, when properly equipped, serve as measuring instruments in themselves. Such methods can be used only in a limited number of cases because of the enormous experimental effort required and the high demands that they place on the patient’s readiness to cooperate. Measurements of this type on external fixators provided the first demonstration that these implants are not under any greater stress during sitting than during standing (Wilke et al. 1992). The experimental set-up was taken a step further with the fitting of measuring instruments on internal fixators (Rohlmann et al. 1999, 2000, 2001) (Fig. 11.19). Rohlmann et al. (2004) report the measured stresses in the internal fixators of 10 patients in various bodily positions and during the performance of various activities. The stresses that were measured in the sitting position were approximately 10% lower than during standing and very much lower than during walking. The type of furniture that the patients sat on (stool, office chair, exercise ball, kneeling stool) made little difference with respect to stress. Office chairs with a backrest that could be inclined backward led to less stress on the implants than conventional office chairs. A comparison of the measured implant stresses with the intradiscal pressures measured in one of the experimental subjects revealed close agreement between these two variables for many activities, as long as the measured value of each variable in the standing position was used as a reference.
Fig. 11.19 Stress measurements with an internal fixator fitted with a measuring instrument. Within the measuring component, there are six load sensors consisting of semiconductor extension-measuring strips. A flat coil and a small receiver antenna were fastened to the patient’s back for the measurement. When the fixator was removed as usual after solidification of the fusion, the measuring system was removed with it.
Intradiscal Pressure Measurements
The most reliable in-vivo data are derived from intradiscal pressure measurements of the type first reported by Nachemson and colleagues (Nachemson and Morris 1964, Nachemson 1966, Nachemson and Elfstrom 1970, Nachemson et al. 1986). These investigators inserted a needle with an integrated pressure-sensitive polyethylene membrane into the disk space and connected it to a measuring instrument employing the nanometer principle. The intradiscal pressure was usually measured in the L3/4 disk. The results were normalized to the intradiscal pressure in each subject while standing (which was assigned the value 100%). These measurements showed a marked increase of intradiscal pressure, by about 50%, when the subjects bent forward. If they additionally held weights in their hands, the pressure rose by a further 70–220% of the reference value.
These investigators obtained similar results with respect to the sitting position. Here, too, the intradiscal pressure was raised by forward bending, and even more so by holding additional weights in the hands. Pressure measurements in these positions were approximately 40% higher than during standing. Tensing the abdominal muscles (pressing) causes part of the weight of the upper body to be transferred from the chest cage to the pelvis by way of the abdominal air and fluid “balloon.” The intra-abdominal pressure may rise as high as 140 mmHg (19 kPa) (Bartelink 1957, Eie 1962). Abdominal pressure maneuvers or an orthosis with abdominal support can reduce stress on the lumbar disks by about 30%.
More recent studies on disk stress in the lower lumbar motion segments have replicated Nachemson’s measurements with updated measuring techniques and extended them to further positions and movements of the spine (Wilke et al. 1998, 1999, 2001, 2004). The intradiscal pressure was measured with a flexible pressure gauge with a constant diameter of 1.5 mm that was surgically inserted directly into the nucleus pulposus of the L4/5 disk under sterile conditions (Figs. 11.20, 11.21). Signals were transmitted through a wireless connection to a computer in order to allow the subjects full freedom of movement. Over a period of 24 h, pressures were measured during the following positions and movements, among others: various lying positions, sitting positions on a normal chair, in an armchair, and on an exercise ball (also called a gym ball, Swiss ball, or Pezzi ball), sneezing, laughing, walking, climbing stairs, lifting weights. The pressure changes taking place overnight because of hydration of the disks in the lying position was measured over a 7 h period.
Fig. 11.20 Pressure sensor for intradiscal pressure measurements. The metal sheath, 7mm long and 1.5mm in diameter, was placed in the center of the nucleus pulposus of the L4/5 intervertebral disk.
The first measurements were made while the subjects were still on the operating table. When the subjects lay supine in a relaxed way with mildly flexed legs (ca. 20°), the lowest pressure values of all were measured, ca. 0.08 MPa (0.1 MPa = 1 bar). The pressure could not be reduced any further by using a knee roll or by having the patient lie in the step position. When the legs were extended, the pressure rose to 0.11 MPa; rotation into the lateral position raised the pressure only by a further 0.1 MPa, to 0.12 MPa. The intradiscal pressure in the prone position was intermediate between those measured in the supine and lateral positions, 0.11 MPa. Sitting up from the prone position into the reading position by extending the back and propping the upper body up with the forearms doubled the pressure to 0.25 MPa. The turning maneuver itself (e.g., from supine to lateral) produced pressure spikes up to 0.8 MPa in magnitude. Coughing while supine produced pressures as high as 0.38 MPa, but hearty laughing raised the pressure only to 0.15 MPa.
Fig. 11.22a–f Sitting postures in which the arms are not supported on armrests (from Wilke 2004).
a Relaxed upright sitting: 0.45 MPa.
b Upright sitting, leaning forward: 0.63 MPa.
c Forward flexion with elbows on thighs: 0.43 MPa.
d Flexed, sitting upright: 0.90 MPa.
e Sitting on a Pezzi ball with straight back: 0.50 MPa.
f Sitting on a Pezzi ball with flexed back: 0.65 MPa.
Fig. 11.23 a–h Sitting postures in an armchair (from Wilke 2004).
a Relaxed upright sitting with supported back: 0.33 MPa.
b Stress-reducing sitting position (relaxed sitting): 0.27 MPa.
c Stress-reducing sitting position (relaxed sitting) with supported, extended legs: 0.38 MPa.
d Stress-reducing sitting position with arms folded across the chest: 0.36 MPa.
e Relaxed sitting without backrest (intermediate sitting position): 0.44 MPa.
f Sitting in actively upright position (intermediate sitting position): 0.55 MPa.
g Relaxed standing: 0.48 MPa.
h Supporting the upper body, e.g., while standing up from a chair (bar supports): 0.10 MPa.
In sitting, the pressure varied markedly depending on the degree of support from the back- or armrests (Figs. 11.22, 11.23). Sitting on a chair with a normal, straight back produced a pressure of 0.45–0.5 MPa. Active extension of the lumbar spine raised the pressure to 0.55 MPa. In the sitting position, too, forward bending produced a continual increase in pressure up to a maximum value of 0.83 MPa in maximal flexion, e.g., while tying shoelaces. Pressure in the sitting position could be markedly reduced by leaning back comfortably in an armchair. Relaxed reclining brought the pressure as low as 0.27 MPa.
In walking, the intradiscal pressure was measured at values between 0.53 and 0.65 MPa. The difference between slow and fast walking, or between walking in tennis shoes or barefoot, was small. In jogging, the pressure oscillated between 0.35 and 0.95 MPa. Jogging in tennis shoes lowered the pressure spikes by only a small amount, to 0.85 MPa.
The highest pressure values of all (2.3 MPa) were measured during biomechanically incorrect lifting of a full case of beer weighing 19.8 kg. Incorrect lifting in this case involved a rounded back and straight knees, rather than the correct method of keeping the back straight and bending at the hip and knee, as is taught in back school; correct lifting reduced the peak pressure during lifting to 1.7 MPa. When the case was held against the body at chest height, the pressure was 1.1 MPa; when it was held at the same height but at arm’s length (ca. 60 cm) from the upper body, the pressure was 1.8 MPa (Fig. 11.24).
Conclusions. The findings of these more recent studies agreed with the results of Nachemson et al. (1964) with respect to the pressure values during standing, lying, lifting, and carrying. In particular, the pressure differences between lifting with a straight back and flexed knees vs. a rounded back and extended knees were reproduced with the newer methods. These important results of the older and newer studies measuring intradiscal pressure confirm rules 2 and 3 of back school (see Table 14.1): keep the back straight and kneel to lift. Incorrect lifting of a heavy weight, such as a 20kg case of beer, with rounded back and straight knees raised the intradiscal pressure to 450% of its initial value in the newer studies. This very high value was not matched even during trampolining. If the case was held correctly, however, with flexed knees and a straight back, as taught in back school, the intradiscal pressure rose only to 340% of its initial value. Finally, when the case, after being lifted, was held as close as possible to the body and the subject assumed a mildly lordotic posture, the pressure was only 200% of the value in normal standing.
On the other hand, the marked pressure differences that Nachemson et al. (1964) found in standing vs. sitting, or in lying in different positions, were not confirmed in the more recent studies. It could not be confirmed, for example, that comfortable (relaxed) sitting actually raises the intradiscal pressure by 40%. The current studies show rather that sitting in this position lowers the pressure by 10%; this could be demonstrated both with high-precision stadiometric measurements and with instrumented internal fixators (Rohlmann et al. 2000). The earlier finding that the intradiscal pressure was three times as high in the lateral decubitus position as in the supine position could not be replicated either; the current studies show only a small difference.
The new findings on the relaxed sitting position are of great importance. Relaxed sitting with diagonal positioning of the back and “deposition” of the upper body on the backrest lowers pressure markedly, and the subject also feels this reduction of pressure to be comfortable. As soon as the subject rose from the relaxed sitting position to the upright position, there was a marked increase of pressure in the lumbar disk (Fig. 11.25). Thus, even while seated, the individual can improve diffusion, and thus the nutritive process, within the intervertebral disks by regularly alternating between the upright and relaxed sitting positions (Fig. 11.26).
The differences between the newer measurements and those of Nachemson et al. (1964) are probably due to the different measuring techniques. Nachemson et al. (1964) integrated their measuring devices into a stiff cannula that could be bent, e.g., by muscle tension or displacement, which might have given rise to false signals and thus to incorrect measured values. The pressure gauges that are currently used cannot be bent out of shape, because they are flexible along their entire length. The only nonflexible element is the 7 mm metal tip, which, after implantation, is located completely within the nucleus pulposus, where it is exposed to hydrostatic and therefore equally distributed pressure. The new findings are confirmed by their excellent agreement with measurements made on external fixator implants (Wilke et al. 1992) and stadiometric measurements (van Deursen 2001).
Fig. 11.24 a–h Intradiscal pressure measurements during lifting and carrying (from Wilke 2004).
a Lifting a case of beer according to the rules of back training: straight back, bent hip and knee joints, case directly in front of body: 1.7 MPa.
b “Wrong” lifting of a case of beer: rounded back, extended knee joints, case far out in front of the truncal axis: 2.3 MPa.
c Gymnastic exercise with rounded back and extended knee: 1.6 MPa.
d Holding and carrying a case of beer next to the trunk with straight back: 1.0 MPa.
e Holding and carrying a case of beer about 60 cm in front of the trunk: 1.8 MPa.
f Carrying a case of beer with one arm: 1.0 MPa.
g Lifting two cases of beer, one with each arm: 2.1 MPa.
h Carrying two cases of beer: 0.9 MPa.
Fig. 11.25 Comparison of disk pressure measurements by Nachemson et al. (1964) with those of Wilke et al. (1992– 2001). The absolute pressure values shown below the bars are from the newer measurements. The relaxed sitting position with reclined backrest, in which part of the weight of the upper body is supported by the backrest, yields a lumbar intradiscal pressure of 0.3 MPa and is thus associated with a significant reduction of stress on the lumbar disks. The low pressure allows fluid uptake into the disk even during sitting (see Chapter 4) (from Wilke 2004).
Fig. 11.26a, b Intradiscal pressure in the L4/5 disk in various bodily positions (Wilke 20004) (a) and pressure-dependent fluid shifts at the edge of the disk (Krämer 1997) (b).
Special Pathological Anatomy and Pathophysiology
Protrusions and Prolapses
Terminology and Classification
The terms used in clinical practice should be well defined in order to convey precise information about the pathological anatomical changes that are present in so-called disk prolapse or disk herniation. Is the finding a bulging disk whose anulus fibrosus is still intact, or is it a prolapse with a free fragment of disk tissue lying outside the disk space? A wide variety of transitional states can occur, ranging from an intradiscal displacement of tissue to free sequestration of disk tissue in the spinal canal.
The current consensus in German-speaking countries is to use the terms Protrusion (protrusion) and Prolaps (prolapse), following the recommendation of the Working Group (now called the Section) on Degenerative Spinal Diseases of the German Society for Orthopedics and Orthopedic Surgery (Krämer 1983, Pharmaceuticals Commission of the German Medical Association 2006). These terms are used in the manner described in the relevant literature: DePalma and Rothman (1970), Rothman and Simeone (1982), Crock (1983), McCulloch (1989, 1998), Farfan (1996), Postacchini (1998), Nachemson and Jonsson (2000), Fardon (2001), Bendix (2004), Borenstein et al. (2004), Pearson et al. (2008).
Protrusion
A disk protrusion is a bulging of a disk whose anulus fibrosus is more or less well preserved. A disk prolapse is a disk herniation with perforation of the anulus fibrosus. Even in the case of a prolapse perforating the anulus fibrosus, the disk material outside the anulus may still be enclosed in a thin membrane. In the case of a disk protrusion, in which the disk tissue is displaced within the disk, the fibrous ring around it is largely intact (grade I or II dislocation) (see Fig. 11.31). Intradiscally injected contrast medium remains within the disk and does not flow into the epidural space. A protrusion (bulging) in this sense corresponds to the usual Anglo-American terms, “contained” or “bulging” disk (Fig. 11.27). Farfan (1996) calls it “intradiscal loose islands of disk material.”
Fig. 11.27 a–c Protrusions (grade II dislocations) of lumbar disks. The anulus fibrosus is intact. There is a possibility for the displaced tissue to return to the center of the disk.
a Medial protrusion: lumbago.
b, c Paramedial protrusion: protrusion sciatica.
A covered prolapse (grade III dislocation, see Fig. 11.31) is one in which the displaced disk tissue has perforated the anulus fibrosus but is still covered by a thin membrane, the so-called ventral epidural membrane (Ludwig 2004). Fragments that find their way under this membrane or under part of the posterior longitudinal ligament, which becomes thinner laterally, are called submembranous or subligamentous sequestra (fragments) and correspond to the covered perforations found at surgery. The term “herniation” can also be used correctly in this situation, because the displaced tissue is still covered by a tissue layer. When contrast medium is injected into the disk (discography), it fills the space occupied by the prolapsed tissue, which sometimes extends to supra- or infradiscal levels.
The disk tissue that lies outside the disk space, but remains covered, still remains in communication with the intervertebral space by way of its path of exit and is therefore in an exceptional situation from the biochemical point of view as well. The displaced disk tissue remains a part of the osmotic system of the intervertebral disk, i.e., it is still nourished by diffusion by way of the pressure-dependent fluid shifts within the disk. This tissue, therefore, does not shrink as rapidly as a fragment lying freely within the spinal canal, which is exposed to the enzymatic activity of the lymphatic fluid, among other influences. Furthermore, the still-covered disk tissue outside the intervertebral space continues to participate in the normal fluctuations of consistency and volume affecting the disk.
As long as there is still a strong layer of anulus fibrosus over the disk protrusion, the protruding tissue may still be able to find its way back into the center of the disk (unless it is a so-called incarcerated fragment). This possibility is exploited by a number of therapeutic techniques, e.g., traction, step positioning and movement therapy.
A distinction must be drawn between disk protrusion through outward displacement of the centrally located, mobile disk tissue and simple outward bulging of the anulus fibrosus in a totally degenerated disk (Fig. 11.28).
Anulus fibrosus bulges of this type are often found at multiple segmental levels simultaneously and become more pronounced with axial loading and backward bending. They play a role in the narrowing of the spinal canal in lumbar spinal stenosis, particularly when they are located at the level of the upper edge of the ascending facet.
Prolapse
A disk prolapse involves complete perforation of the anulus fibrosus and the ventral epidural membrane. The dislocated disk tissue lies more or less free within the spinal epidural space; the perforation is no longer covered. Intradiscally injected contrast medium flows into the epidural space and distributes itself within it. The intraspinal fragment may still be contiguous with the disk (grade IV dislocation) and thus be located “half inside and half outside.” A free fragment (grade V dislocation) lies free in the epidural space and has no connection to the disk.
Once it has perforated the posterior border of the disk, the dislocated disk tissue can travel in any direction. In general, the prolapsed material migrates laterally and caudally along the nerve root, compressing it from the ventral side. The root is thereby raised and pressed against the posterior wall of the spinal canal (lamina, ligamentum flavum). The prolapsed tissue can also migrate cranially or further caudally to compress a nerve root at an adjacent level.
Fig. 11.28a, b Rolling-out of the anulus fibrosus due to narrowing of the intervertebral space because of disk degeneration or surgical removal of the disk. The protruding tissue is the anulus fibrosus.
Fig. 11.29a, b Medial and paramedial herniation (grade IV dislocation) of a lumbar disk. The anulus fibrosus is perforated. The displaced tissue cannot return to the center of the disk.
a Medial prolapse: cauda equina syndrome.
b Paramedial and lateral prolapse: prolapse sciatica.
Fig. 11.30a–c Different directions in which prolapsed disk tissue can migrate (grade V dislocation) at a single level.
a Laterally, with compression of the nerve root in the intervertebral foramen.
b Medially, with bilateral sciatica.
c Dorsally, with dorsolateral compression of the cauda equina.
Every large operative series contains not just standard situations like these, but exceptional cases as well. If the prolapsed material migrates medially, the contralateral nerve root can be compressed: sciatica on alternating sides is the result (Fig. 11.29). The material can also migrate around the dura mater to compress the nerve root from the dorsal side (Fig. 11.30). Very rarely, hard sequestrated fragments can perforate the ventral dura mater of the lumbar sac and present as an intradural or intrathecal fragment. Such cases have been reported by Roda et al. (1982), Lee (1983), Griss (1984), Kasch (1986), Yildizhan and Okten (1991), McCulloch (1998), and Postacchini (1999).
Grades of Dislocation
The classification of disk protrusions and prolapses by grade of dislocation (I–V) is shown in Fig. 11.31. The grade of dislocation, together with the clinical findings, determines the most advisable form of treatment; intradiscal therapy may still be possible, or an open operation may be necessary (Figs. 11.32–11.37).
Fig. 11.32 Grade II dislocation. Subanular fragment; dislocated disk tissue has become displaced to the outermost layer of the anulus fibrosus and pushes this layer outward (“bulging disk,” “contained disk”). The dislocated disk tissue can be reached at surgery only by incising the anulus fibrosus.
Spontaneous Changes of Disk Prolapse
Once the disk tissue has left its usual environment in the intervertebral space and entered the epidural space, it is subject to new metabolic conditions. It was originally nourished by diffusion through the normal, pressure-related fluid shifts within the disk, but it now lies more or less suddenly in a space surrounded by connective tissue where it is exposed to lymphatic fluid.
When the disk tissue is no longer subject to intradiscal pressure, it takes up fluid, according to the relation discussed in Chapter 4, thereby swelling up and increasing markedly in volume. This occurs because of the laws of osmosis and the intrinsic turgor of disk tissue. We have been able to show, in experiments on dissected specimens of disk tissue (Krämer 1973), that the volume of a disk prolapse induced by identical mechanical conditions is greater in hypo- or isotonic solution than in hypertonic solution. Nevertheless, neither this experiment nor others carried out on operatively removed disk tissue (Wittenberg et al. 1990) revealed any significant shrinkage of prolapsed tissue when exposed to hypertonic solution.
Fig. 11.33 Grade III dislocation. Covered prolapse at the discal level. The prolapsed tissue has completely perforated the anulus fibrosus and is only covered by a thin membrane (the ventral epidural membrane). This membrane can be bluntly perforated, e.g., with a 2 mm dissector. In general, the edge of the lamina will have to be resected for the prolapse to be reached.
Fig. 11.34 Grade III dislocation. Covered prolapse at the supradiscal level. The dislocated disk tissue has emerged from the intervertebral space but is still covered by the ventral epidural membrane. The dislocated disk tissue can be reached only by opening the membrane with a blunt 2 mm dissector. In general, the lower edge of the lamina will have to be resected for the prolapse to be reached. A hemilaminectomy may be necessary.
Fig. 11.35 Grade III dislocation. Covered prolapse at the infradiscal level. The prolapsed tissue is found under the ventral epidural membrane. After blunt opening of this membrane, e.g., with a dissector, the dislocated disk tissue is reached. The submembranous infradiscal prolapse can generally be reached by fenestration at L4/5 and L3/4. At the L5/S1 level, part of the sacral lamina may need to be resected.
Fig. 11.36 Grade IV dislocation. The dislocated disk tissue is found partly inside and partly outside the disk. This situation generally obtains only at the discal level, when the ventral epidural membrane is also perforated at this level. Extensive removal of the dorsal portion of the disk is necessary to prevent recurrent disk herniation.
Fig. 11.37 Lumbar disk herniation, grade V dislocation. The prolapse is found as a free fragment in the spinal canal or the intervertebral foramen. When the prolapse is removed, the neighboring disk (donor disk) does not necessarily have to be emptied of disk tissue.
Increased pain and the progression of neurological deficits in the initial phase of discogenic sciatica are due, among other things, to spontaneous uptake of fluid by the prolapsed disk fragment (hydration), leading it to increase in volume.
Fluid uptake by prolapsed tissue can be demonstrated by MRI: on a T2-weighted image, the prolapsed tissue has higher signal intensity than the disk from which it emerged (Fig. 11.38).
Prolapsed disk tissue in the epidural space remains swollen for several weeks. As mentioned in the discussion of the osmotic system in Chapter 4, the capacity for hydration and dehydration of disk tissue depends on its proteoglycan content. Nucleus pulposus tissue can take up more fluid than anulus fibrosus or cartilaginous end-plate tissue.
After a period of hydration, the prolapsed disk tissue begins to become dehydrated and lose volume.
Its water content and volume decrease and the pressure on the nerves diminishes. Depolymerization and enzymatic degradation of the hydrophilic molecules in the disk fragment causes it to lose turgor. These processes can be verified at surgery: freshly prolapsed tissue (removed only a short time after the onset of symptoms) has more pronounced turgor than old extradiscal fragments, which are said in surgeons’ jargon to be “pulpy.”
Aside from osmotic swelling and shrinkage, extradiscal fragments are also enzymatically degraded.
Disk tissue in the spinal canal induces a foreign-body reaction.
The disk and the nerve root are indeed part of the same motion segment, as far as their function is concerned, yet they are composed of very different types of tissue that arise from different primordial layers during embryonic development. The mechanical impingement and biochemical influence of disk tissue on epidural neural structures induce an inflammatory reaction that accelerates the degradation of the disk tissue. Small fragments are directly enzymatically resorbed by phagocytosis. It is therefore necessary, when a free fragment is to be surgically removed, to verify by a pre-operative MRI scan that it is in fact still there. In some cases, persistent neurological deficits are not due to the persistence of compressive disk tissue but are rather the residua of past nerve compression.
Larger disk fragments are degraded by vascularization and connective-tissue organization from the surrounding epidural fat. Nucleus pulposus tissue is more easily degraded by macrophages and T cells than anulus fibrosus tissue. According to our own studies (Owczarek and Schmidt 1994) and others reported in the literature (McCulloch 1998, Postacchini 1999), once a disk prolapse has been spontaneously resorbed, follow-up CT and MRI scans reveal no significant degree of scarring or adhesion formation in the spinal canal.
The time it takes for prolapsed disk tissue to be spontaneously resorbed depends on the mass and composition of the fragment as well as its position in the spinal canal.
Large fragments, i.e., those that occupy more than one-third of the cross-sectional area of the spinal canal, are less likely to be resorbed in an acceptably short period of time than smaller fragments. Parts of the anulus fibrosus and the cartilaginous end plates are resorbed more slowly than parts of the nucleus pulposus. Hard disk fragments therefore more commonly require surgery and also cause more intense pain (Krämer, Herdmann, and Krämer 2005, Willburger 2005). Supra- and infradiscal fragments are resorbed more rapidly because they are surrounded by the well-vascularized ventral epidural membrane and the posterior wall of the vertebral body. The farther away the fragment is from the donor disk, the more likely it is to be spontaneously resorbed. If it is located in the concavity of the posterior surface of the vertebral body, the disk prolapse also exerts less pressure on the dura mater and nerve roots. The fragment has less of an opportunity to avoid impingement on these structures if the spinal canal is narrow and if the fragment is located within the foramen. Our group has published reports on the spontaneous course of lumbar disk prolapses that have been successfully managed conservatively, as observed in serial CT and MRI scans (Owczarek and Schmidt 1994, Krämer and Wilke 1988, Krämer, Hermann, and Krämer 2005), and so have many others (Koeller et al. 1984, Saal and Saal 1989, Hirabayashi et al. 1990, Saal and Herzog 1990, Yutaka et al. 1993, Donelson et al. 1997, Martikainen 1998, Trasimeni 1998, Fergusson 1999, Aelart 2004, Nakagawa 2004) (see also Chapter 12).
In some cases, total resorption of the prolapsed fragment is accompanied by complete remission of the clinical signs and symptoms; in other cases, the clinical findings regress partly or completely despite unchanged findings on CT or MRI. The prolapsed tissue usually becomes calcified. In such cases, the patient’s condition tends to improve after a shorter or longer follow-up interval.
Fig. 11.38 Lumbar disk herniation, grade III dislocation. The extradiscally located tissue is brighter (contains more water) than the disk from which it was extruded.
Fig. 11.39a, b Symptom-producing factors when a lumbar disk is slack, and their accentuation by lordosis.
Disk Slackening and Instability
Not all symptoms arising from the lumbar motion segment are due to displacement of disk tissue. Apart from protrusions and prolapses, there are a number of other pathological structural changes that can be collectively designated by the term “disk slackening.” Schmorl and Junghanns (1968) described a condition that they called “instabilitas intervertebralis.”
The term “disk slackening” refers to all phenomena that are due to progressive loss of water from the matrix of the disk and to the loss of fiber elasticity.
Disk slackening and instability do not necessarily imply the presence of clinical signs and symptoms. These conditions become clinically relevant only when pain and disability arise as a result of instability (see Chapter 6). If disk slackening and instability are present in combination with the associated clinical manifestations, the term “clinical instability” can be used (White and Panjabi 1978, Frymoyer et al. 1979, Kirkaldy-Willis 1984, Farfan 1996, Krismer et al. 1997). Instability leads to insufficiency of the lumbar erector spinae muscles, excess stress on the intervertebral joints, and sometimes manifestations of nerve root irritation. Disk slackening also plays a role in the pathogenesis of lumbar spinal stenosis.
In its initial stages, disk slackening can be compensated for by the abdominal and erector spinae muscles when the spine is under normal amounts of stress. If the functional reserve capacity of these muscles is exceeded, however, signs of insufficiency appear. The most commonly affected muscles are the deep and superficial erector spinae muscles of the lumbar motion segments, though the proximal muscles of the lower limbs can also be affected. Not just the muscles, but also the intervertebral joints are subjected to increased stress by disk slackening. The intervertebral joints of the lumbar spine already have a greater range of normal positions than those elsewhere in the spine because of the marked pressure and volume fluctuations of the lumbar disks (Fig. 11.39).
If the lumbar disks are also slack and subject to greater than normal fluctuations in volume, abnormal and excessive stress on the lumbar intervertebral joints can result, producing arthrogenic symptoms after long periods of mechanical loading followed by unloading. If the joints are subject to abnormal or excessive stress for a long time because of disk slackening, spondylarthrosis can develop (arthrosis deformans).
Disturbances of the muscles, ligaments, and joints arising in the setting of lumbar disk slackening are the cause of arthroligamentous low back pain.
Displacement of the vertebral bodies with respect to one another can also come about because of disk slackening. Dorsoventral dislocation was called pseudospondylolisthesis in the nomenclature of Schmorl and Junghanns (1968)—“pseudo,“ because the articular facets are fully intact in this condition, as opposed to genuine isthmic spondylolisthesis. The most commonly used term in current clinical parlance is degenerative spondylolisthesis. Rotatory spondylolisthesis is usually due to disk slackening in combination with scoliosis, though it can also occur in the absence of scoliosis.
Sciatica can also be produced by slackening of intervertebral disks without any displacement of disk tissue (Benini 1976, Krämer, Herdmann, and Krämer 2005). In a slackened motion segment, the disk is of less than normal height and the lumbar vertebrae are displaced to some extent in relation to one another. The intervertebral foramina are narrowed, producing nerve root compression, mainly when the disk loses height dorsally and the vertebral body above is simultaneously posteriorly displaced with respect to the one below. The intervertebral foramen between them is then narrowed ventrally by the posterior border of the upper vertebra and by the upper portion of the articular facet of the lower vertebra. The lateral and oblique views of a myelogram or myelographic MRI scan will then reveal indentations in the column of contrast medium (spinal canal stenosis).
If a disk is slackened, low back pain and radicular symptoms tend to arise in particular positions and with particular movements, e.g., reclination of the trunk.
Hyperlordosis of the lumbar spine is an important symptom-provoking factor in slackening of the lumbar disks.
Returning the lumbar lordosis to its normal shape through relaxing positions and postures thus plays a major role in the treatment and prophylaxis of disk disease (Fig. 11.40).
Clinical course. Disk slackening and its clinical manifestations are present during a transient, though sometimes quite prolonged, phase in the course of disk degeneration. As the disks progressively dry out and become fibrotic with advancing age, the abnormal movements and displacements of the motion segment also diminish, so that the symptoms gradually disappear— unless the bony changes at the intervertebral joints have lead to narrowing of the spinal canal, i.e., spinal canal stenosis.
Fig. 11.40a, b Slackened lumbar motion segment in kyphotic posture (a). When the patient rapidly leans back, the inelastic anulus fibrosus is rolled out posteriorly (b).
Bony Deformation
The types of bony deformation arising in spondylosis, osteochondrosis, and spondylarthrosis of the lumbar spine are not as clinically significant as those in the cervical spine, but they can nonetheless produce bony compression of nerve roots with symptomatic nerve root irritation. Ventral and lateral spondylotic osteophytes can often be very prominent in the lumbar spine yet are of no clinical relevance. They provide documentary evidence of disk slackening that has occurred in the past.
Of greater clinical significance are small osteophytes on the posterior border of the vertebral body, so-called retrospondylosis, particularly when they are dorsolaterally located and compress a nerve root.
Dorsal osteophytes can arise by appositional bone growth at the edge of the vertebral body. It is also possible, however, for a small prolapse to harden by calcification and then become rigidly attached to the dorsal edge of the vertebral body.
In such cases, one speaks of a “hard prolapse.” Though bony projections of this type develop slowly, giving the nerve root sufficient opportunity to adapt, they may be so sharply pointed that they repeatedly induce segmentally radiating pain. Inflammatory adhesions and bridging bands gradually form and finally encase the nerve root, maintaining it in a state of chronic irritation.
This situation is often not recognized because the objective clinical signs and changes on CT and MRI are not very pronounced. It can be remedied only by surgical removal of the osteophytes and freeing of the nerve roots from the surrounding tissue (rhizolysis). In spondylarthrosis, osteophytic reactions also arise at the edges of the intervertebral joints. If they jut into the spinal canal, they cause degenerative spinal canal stenosis. Because the lumbar intervertebral joints are part of the dorsal wall of the intervertebral foramina, spondylarthrosis can also cause foraminal stenosis. As mentioned above, bony deformation alone is not clinically relevant; symptoms are produced only when the segment is also slack.
In end-stage disk degeneration, fibrosis and osteophytes are still present, but there is no longer any segmental instability and the pain therefore improves. The lumbar spine has a markedly restricted range of motion in all directions. New protrusions and prolapses arise much less commonly in end-stage disk degeneration, but they sometimes occur even in individuals aged 70 or older.
Summary: Anatomy, Biomechanics, and Pathology of the Lumbar Motion Segments
In the lower lumbar spine, the intervertebral foramina and the spinal nerves traveling through them are situated at the level of the intervertebral disks, so that degenerative changes of the disks lie in the immediate vicinity of the spinal nerves. The traversing and exiting L5 and S1 nerve roots can be compressed by disk protrusions and prolapses in the anterolateral epidural space; from the dorsal side, they can be impinged upon by displacement or arthrotic enlargement of the L4/5 and L5/S1 articular facets. Local and radicular lumbar syndromes usually arise from these critical points in the lower two lumbar motion segments. Variations in the number of lumbar vertebrae and the possible presence of transitional vertebrae should be taken into account when planning treatments by local (epidural or foramino-articular) injection or open microsurgical procedures on the lumbar spine.
High intradiscal pressure in the lower lumbar intervertebral disks is the cause of intradiscal tissue displacement, leading to protrusions and prolapses. In children and adolescents, this produces extension stiffness of the hip and thigh; in adults, it produces lumbago. There is a continuous pathological spectrum ranging from disk protrusion to disk prolapse. Dorsal and dorsolateral displacements of disk tissue are clinically significant. These produce both low back pain and signs of nerve root irritation. The pattern of clinical symptoms is determined by the precise site of a lateral disk protrusion, i.e., whether it points more toward the midline, laterally, or fully outward (into the neural foramen). Protrusions and prolapses are classified according to the extent of the tissue dislocation and the amount of tissue that still separates the dislocated disk tissue from the neural structures in the epidural space. The therapeutic approach is determined by the major clinical manifestations rather than by the pathoanatomical finding alone.
Conclusion
The vast majority of cases of low back pain and sciatica arise from a circumscribed region of the lower lumbar spine characterized by a special combination of topographical, anatomical, and biomechanical properties.
Clinical Features of Lumbar Syndromes
Introduction
Just as in the cervical spine, the clinical symptoms and signs of damage to the lumbar disks are characterized by a wide variety of pathological manifestations. The range of symptomatic conditions ranges from mild low back pain to a deep spinal transection syndrome, with all possible transitional states in between. The spectrum of organs that can be directly or indirectly affected is not as wide as in cervical syndrome, but nevertheless the course, extent, and intensity of the symptoms in the back and the sciatic distribution are highly variable and their systematic classification is difficult. The same pathoanatomical substrate—e.g., a lateral disk protrusion—can produce symptoms that differ from patient to patient and that change over time in the individual patient as well. This variability is due to biochemical and biomechanical changes in the disk matrix and to individual differences in the responsiveness of the central and peripheral nervous systems to external stimuli.
At the onset of a lumbar syndrome, the symptoms produced by disk tissue displacement still correspond to well-defined clinical patterns (lumbago, sciatica). Later, as the secondary degenerative changes in the motion segment increase, it becomes increasingly difficult to ascribe the disease manifestations—e.g., chronic low back pain—to particular pathoanatomical states.
Lumbar syndromes are broadly classified according to the most prominent clinical manifestations of disease, with the use of generally recognized terms such as lumbago and sciatica. Some further specification is necessary, however, so that the clinician can draw the necessary therapeutic consequences; e.g, protrusion sciatica should be distinguished from prolapse sciatica. In the following sections, we present the general types of symptoms and signs that appear in all disk-related conditions of the lumbar spine. The specific findings of particular segmental syndromes will be discussed later on in the relevant sections.
Symptoms
Pain is the most important symptom of lumbar syndrome. What the patient says about the onset, development, and responsiveness of the pain provides important clues to the diagnosis. Objective signs are often mild or absent despite severe pain; thus, physicians often have difficulty assessing the situation correctly, especially (but not only) in the medicolegal setting. Moreover, the physician often sees the patient for the first time only after the pain has begun to diminish in intensity, or during an asymptomatic interval.
![]() | |
![]() | |
![]() | |
![]() |
The patient can usually describe the localization and character of the pain so precisely that the diagnosis of “lumbar syndrome” can be given on the basis of the history alone. When objectifiable neurological deficits are lacking, the only evidence for lumbar root syndrome is the typical band of pain in the lower limb, whose precise location is also an important clue to the affected segment. The patient complains of numbness or sensory irritative manifestations such as a pins-and-needles sensation or the feeling that the limb has “gone to sleep.” The severity of the pain is, in general, proportional to the pressure exerted on the nerve root, until the area supplied by the root becomes entirely anesthetic and analgetic when the nerve is maximally compressed.
Disk-related symptoms in the lumbar region generally develop over a short time; in most cases, their onset is sudden (Table 11.1). Many patients attribute the onset of the pain to a particular event, perhaps because of a natural desire for a plausible explanation. The events most commonly mentioned in medicolegal assessments are faulty lifting and rotatory movements of the trunk.
More than half of the patients who were interrogated by questionnaire in specialized practices and in our outpatient clinic (Fig. 11.41) reported no particular event before the onset of the pain to which the pain could be attributed. Their sciatica or lumbago appeared to strike them out of a clear blue sky. Accidents and other mechanical forces of the types commonly discussed in medicolegal cases played a numerically insignificant role in this group of nonmedicolegal patients.
Fig. 11.42 Gradual resolution of sciatica to isolated low back pain: McKenzie’s centralization phenomenon.
The character and radiation of the pain in lumbar syndromes are subject to continuous change: pain lying deep in the low back moves to one buttock or to the sacroiliac region on one side. Sciatica sometimes arises, too, initially only in the thigh, then later radiating all the way down to the foot. The low back pain may entirely disappear, leaving only sciatica in the lower limb (sciatica without low back pain). The reverse type of varying course is seen when the pain moves proximally, reflecting a centralization phenomenon (McKenzie 1987): sciatica changes to isolated low back pain (Fig. 11.42).
The positional dependence of disk-related symptoms is more pronounced in the lumbar region than anywhere else in the spine because of the marked fluctuations of intradiscal pressure and the changing relative positions of the neural structures and the posterior border of the disk that accompany postural shifts. Both low back pain and sciatica are functions of the position of the lumbar motion segments and the mechanical demands placed on them. This is also reflected in the circadian variation of symptoms. Aside from intradiscal pressure, another important question is whether the patient is able to assume a stress-reducing position. If this is not possible, the pain intensifies even when the patient lies down with a lordotic posture of the lumbar spine, e.g., in the prone or flat supine position. Forward bending or sitting in a chair raises the intradiscal pressure considerably, though many patients are asymptomatic when they sit because this posture widens the intervertebral foramina. Only when the patient sits up, increasing the lumbar lordosis, does the slackened posterior portion of the disk get caught in the “nutcracker” of the posterior vertebral body edges and protrude toward the dura mater and nerve roots. This situation arises at night, too, when the patient shifts from the kyphotic lateral decubitus to the flat supine or prone position. Most patients are most comfortable when lying with flexed hip and knee joints, either on their back or on the side (the “fetal” position).
Other patients, however, say that they feel relief only when they are prone or bending backward. The asymptomatic, stress-reducing position thus differs from patient to patient depending on the site of the protrusion or prolapse.
The exacerbation of pain by coughing, sneezing, or pressing is a common feature of all disk-related symptoms in the lumbar region. The importance of the valve-less epidural venous plexus in the spinal canal and its relation to intraabdominal and intrathoracic pressure has already been discussed in Chapter 4. Nachemson (1976), in his measurements of intradiscal pressure, found that abdominal pressing raises the pressure in the lumbar disks to a considerable extent. Patients are aware of, and fear, these elevations of pressure and assume all possible pressure-reducing positions when abdominal pressing is unavoidable. The dependence of the pain on position and on pressing is subject to change at different times of day and also over the course of the illness. Any reported bladder or bowel symptoms or sexual dysfunction can be an important clue to a possible partial cauda equine syndrome; any weakness of the dorsiflexors of the feet and toes, or of the gluteal and calf muscles, may indicate a motor disturbance of a type that often accompanies sciatica.
Some patients with S1 syndrome report cramps in the calf muscles. Motor dysfunction limited to the monosegmentally supplied muscles must be clinically distinguished from pain-related, reflexive “favoring” of an entire limb. Patients will often state that the limb seems to be “weak” or “paralyzed” even though the examination reveals no motor deficit; they are merely reflexively avoiding movement in order not to stir up additional pain.
At the initial examination, the physician must allow sufficient time to take a full history from the patient, because the symptoms that the patient relates often provide crucial clues to the diagnosis.
A physician who is a “history fanatic” has the best chance of diagnosing a disk syndrome correctly.
Findings of Clinical Examination
After taking the history, the physician should carry out a thorough physical examination, consisting of inspection, palpation, functional testing, and a directed neurological examination. Special tests for lumbar syndrome should be a routine part of the overall examination, to facilitate differential diagnosis. The examination should be structured in such a way that the patient needs to move as little as possible, because each change of position will be difficult and painful.
Thus, multiple tests (e.g., tests of range of movement and neurological function) should be carried out in each position of the body.
The first indication that a lumbar syndrome may be present is already evident on inspection of the patient when they enter the room. Their gait is inharmonious, cautious, and (if the patient suffers from sciatica) limping. The patient prefers to stand during clinical history-taking, or sits down hesitantly on the edge of a proffered chair. All movements, including sitting down, standing up, dressing, and undressing, are executed cautiously and appear stiff. The patient has great difficulty taking off shoes and socks, because this maneuver requires bending the trunk forward, which is painful. Postural abnormalities may already be noted while the patient is still dressed; they are most noticeable when the unclothed patient is viewed from behind.
Closer inspection reveals a marked bulging of the tense lumbar erector spinae muscles (lumbar spasm). Flexion of the lumbar spine to eliminate the lordosis makes this even more evident.
The presence of lumbar spasm and partial fixation of the lumbar spine are among the most important diagnostic criteria for lumbar syndrome.
The standing patient is first asked to bend forward and to the sides and to rotate the trunk actively, and then these same movements are tested passively. A limited range of motion to one side is found. Lateral bending is not as severely restricted as forward and backward bending. The abnormal posture and limited range of motion of the trunk may sometimes be only mild or even absent, e.g., in the asymptomatic interval of a lumbar syndrome or with a far lateral S1 disk protrusion. Painful limitation of motion of the lumbar spine is often not evident until the patient is asked to bend laterally while keeping the trunk bent slightly forward. If the physician suspects that a limited range of forward bending may have a nonorganic cause (e.g., in medicolegal situations or possible psychogenic overlay), a kneeling test can be done at this point (see Chapter 13): if the patient still cannot bend forward (at the hip joint) when kneeling on a chair, a position in which the sciatic nerves and ischiocrural muscles are relaxed rather than stretched, then the limited range of motion is probably of nonorganic origin.
Next, the patient is asked to stand, and to take several steps, when standing on tiptoe and then on the heels; this will reveal any possible weakness of the foot dorsiflexors (L5) or calf muscles (S1).
The remainder of the examination is carried out with patient lying down. Lumbar spasm often disappears when the patient is horizontal, because the trunk muscles no longer have a stabilizing, antigravity task to do. The supine position with mildly flexed hips and knees is usually the most comfortable position for the patient (the maximally stress-reducing position). Patients find it unpleasant to lie flat in the prone position.
With the patient prone, the physician should palpate the spinous processes and ascertain whether there is any tenderness of the affected motion segment to gentle shaking or percussion. Because the lumbar intervertebral joints are sagittally oriented, lateral shaking of the spinous process will always produce movement of the vertebral bodies above and below as well; thus, this part of the examination cannot be considered segmentally specific.
Paraspinous tenderness is more informative: in thin and not particularly well-muscled patients, one may be able to exert pressure on the ligamentum flavum and the nerve root lying directly under it by deep palpation with one finger. The soft tissues between the laminae may be depressed by this maneuver to such an extent that the nerve root is squeezed between them and a disk protrusion lying anterior to it in the spinal canal (Fig. 11.43), resulting in the provocation of the patient’s typical local and sciatic pain. This pain on deep palpation is still more intense if it is evoked when the patient’s back is in a lordotic posture, with flexed hip joints, in the kneeling, squatting, or lateral decubitus position, because these positions increase the height of the interlaminar window.
Fig. 11.43 Deep paravertebral palpation with pressure on the nerve root that is compressed by a disk herniation.
Fig. 11.44 Overextension of the hip causing femoral nerve stretch pain (the “reverse Lasègue sign”) in a patient with an upper lumbar radicular syndrome.
The result of this test is significant only when it is positive; it may be negative, for example, in the presence of a far lateral protrusion or of one located under a broad lamina, neither of which can be reached by deep interlaminar palpation.
The region of the sacroiliac joint on the affected side is also tender to palpation and percussion. This finding does not indicate an abnormal position or blockage of the sacroiliac joint but is rather a type of radiating pain corresponding to the dorsal branches of the affected spinal nerve. Intradiscal injections can provoke this type of pain in typical fashion.
Keeping the patient prone, the physician proceeds to test the ability of the hip joints to be hyperextended, checking for the provocation of pain on femoral nerve stretching, the so-called “reverse Lasègue sign” that indicates a disk protrusion at a higher level (Fig. 11.44).
Just as the sciatic nerve is stretched when the patient lies supine and the lower limb is elevated, the femoral nerve is stretched when the patient lies prone and the hip joint is hyperextended.
Certain parts of the neurological examination can now be carried out while the patient remains prone, starting with sensory testing in the dorsally located dermatomes. The physician asks the patient to tense the gluteal musculature and palpates for any difference in muscle tone that may indicate the presence of an S1 syndrome. The prone position is also suitable for testing of active knee flexion, which can be impaired in higher lumbar root syndromes.
The supine position. After determining that the range of motion of all joints (including rotation at the hips) is free, the physician tests for the presence of the Lasègue sign; this can be done either by raising the straight lower limb or by extending the knee when the limb is already flexed at the hip.
No matter which of these two methods is used, Lasègue’s test consists of hip flexion at a right angle combined with knee extension. If this provokes pain in the back radiating down into the sciatic distribution, the Lasègue sign is said to be positive; the clinician must differentiate among locally induced back pain, classic radicular pain, and the dull discomfort that accompanies stretching of the hamstrings. Lasègue (1864) was also the first to observe that patients with sciatica keep the foot in a plantar flexed position and complain of increased pain when it is dorsiflexed (Fig. 11.45). In common medical parlance, the term “positive Lasègue sign” can also refer to the provocation of pain by raising of the straight lower limb; this is the “straight-leg raising test” often mentioned in the Anglo-American literature. The provocation of pain by this maneuver was first described as a typical finding in sciatica by Forst, a pupil of Lasègue (cited in Finneson 1980).
Straight-leg raising displaces the L4, L5, and S1 spinal nerves by up to 5mm and stretches them by 2–4% (Smith et al. 1993).
Pain is induced by the same mechanism in the straightleg raising test and in the test originally described by Lasègue (passive extension of the knee when the hip is in 90° of flexion): components of the sciatic nerve are stretched.
The straight lower limb can normally be raised to 70–90° by passive flexion at the hip, with some variability between individuals and depending on age. Limb raising is limited by a feeling of tension in the ischiocrural muscles, but no unusual feeling normally arises from the sciatic nerve during this maneuver. Sciatica is provoked only when the sciatic nerve is irritated or if it is less than normally mobile, or already under stretch, in the region of the nerve roots.
The sensitivity and specificity of the Lasègue sign have been investigated by Edgar (1995), Andersson and Deyo (1996), Hogen et al. (1996), Igarashi et al. (2004), and Summers et al. (2005). In their systematic review of the literature, Walter et al. (2000) concluded that the specificity of the Lasègue sign is low. Thus, pain on straight-leg raising should only be considered to be of diagnostic value in the context of other accompanying manifestations of lumbar syndrome.
Fig. 11.45 a–c The Lasègue test.
a Pain on raising the flexed leg.
b Pain on raising the extended leg (straight-leg raising test).
c Additional dorsiflexion of the foot as a test of sciatica.
Depending on the site of the displaced disk tissue, radicular pain may also be induced or intensified by raising of the contralateral lower limb, i.e., the one that is not painful (contralateral Lasègue sign). This is sometimes the case when the prolapse is medially situated and compresses the nerve from the caudal side (Fig. 11.46).
Fig. 11.46 a, b Paramedian disk prolapse in the axilla of the nerve root (a). Tension on the opposite root when the leg is raised increases the pressure exerted by the prolapse on the affected root (positive crossed Lasègue sign) (b).
Straight-leg raising will also be painful in pathological conditions affecting the hip and sacroiliac joints. To rule out these potential sources of pain, one can do the sciatic nerve test (Fig. 11.45c): The straight lower limb is raised to the point at which pain begins to be felt and then lowered till the pain just disappears. If mild passive dorsiflexion of the foot at this point induces pain again, then it can be regarded as certain that a sciatic nerve syndrome is present. Dorsiflexion stretches the tibial nerve by about 1–2 cm. This maneuver does not induce pain originating in the hip or sacroiliac joints, because it causes no additional movement in these joints.
In the popliteal fossa pressure test, the straight leg is raised to the point at which pain begins to be felt, and then the knee is flexed and placed on the physician’s shoulder. Pressure from the physician’s thumb in the patient’s popliteal fossa provokes sudden sciatic pain if the nerve is under tension (Fig. 11.47).
The pain-provoking effect of sciatic nerve stretch in patients with lumbar syndrome is also exploited in the straight-leg sitting test (see Chapter 13). The patient is unable to sit up on a flat surface, e.g., in bed or on an examining table, with the knees extended. This objective sign of the intensity of pain is of particular value in medicolegal assessment: because straight-leg raising is a commonly known medical test, patients will often indicate the presence of severe pain when the leg is raised only to 20° or 30°, but will then be able to converse normally with the physician when sitting up in bed with the knees extended. The same applies to the sciatic nerve stretching test in the sitting position, the so-called “reclination test”: the patient sits up in a chair without leaning back and the examiner raises the patient’s calf. If the patient then leans back because of pain, the test is positive (Fig. 11.48).
Fig. 11.48a, b Positive reclination test: when the leg is extended at the knee and raised, the patient leans backward.
The remaining testing of sensorimotor and reflex function is carried out with the patient supine. The reflexes should be examined very carefully, with distracting maneuvers if necessary, because even slight asymmetry of the reflexes is of diagnostic value. The knee-jerk reflex is often influenced by antalgic tensing of the entire lower limb. The patient must first be brought into as pain-free a position as possible.
Increased reflexes are of no diagnostic value in lumbar syndromes.
The knee-jerk reflex is diminished in compression of the third or fourth lumbar nerve root.
If the Achilles reflex cannot be elicited with the patient supine, it should be tested with the patient in the kneeling position and performing a distracting maneuver, such as interlocking the hands and attempting to pull them apart (the Jendrassik hand grip).
Motor testing in lumbar syndromes focuses on the monosegmentally innervated muscles of the lower lumbar segments, because positive findings here are of the greatest diagnostic significance.
Some useful diagnostic evidence can already be obtained at the start of the examination when the examiner observes the patient during toe-standing, heelstanding, toe-walking, and heel-walking. The motor dysfunction may be so mild that the patient does not notice it. Fibrillation may be seen in affected muscle groups. The magnitude of the motor deficit is generally proportional to the pressure on the nerve root. Large muscles are only mildly weakened in monosegmental lumbar irritative syndromes, even when a nerve root is massively compressed. The quadriceps, for example, is supplied by the L3, L4, and L5 nerve roots, but the extensor hallucis longus is supplied only by L5. An L3/4 disk prolapse compressing the L4 root produces quadriceps weakness that is evident only during active knee extension, but an L4/5 prolapse compressing the L5 root produces marked loss of strength of the foot dorsiflexors (i.e., a foot drop). Thus, the knee flexors and extensors should always be tested, but the most important tests are of the raw strength of the plantar flexors and dorsiflexors of the feet and, specifically, of the big toes (Fig. 11.49).
Although motor testing often yields unexpected findings for both the physician and the patient, the findings of sensory testing are often already partly implicit in the clinical history itself (complaints of numbness, etc.). Because disk-related nerve root syndromes generally impair sensation only superficially, sensory testing can be restricted to directed testing with a pin-wheel, needle, cotton-wool pad, or similar object. Just as in motor testing, dermatomal overlap (particularly in the proximal portion of the lower limb) often precludes reliable assignment of a sensory deficit to a single segment.
Bradford and Spurling (1950) reported a surgical series of S1 rhizotomy (complete transection of the S1 root within its dural sleeve) on a number of patients. They found that this produced a different distribution of hypesthetic areas in the thigh and leg in each patient. The various dermatome charts that are available also differ from one another; the classic ones are those of Foerster (1933), Tilney and Riley (1938) and Keegan (1943).
The only reliable statement that can be made is that an area of the dorsum of the foot that includes the big toe belongs to the L5 dermatome, but the heel, the lateral edge of the foot, and the little toe belong to the S1 dermatome.
Cutaneous sensation must also be tested in the perineal area in order not to miss the finding of saddle anesthesia in cauda equina syndrome.
Hyperesthesia is also a feature of lumbar nerve root irritation syndromes. It is due to an incomplete spinal nerve lesion, a common result of compression by a lumbar disk protrusion. If the examination reveals evidence of altered temperature sense or proprioception or other deficits that are not generally seen in lumbar syndrome, a neurologist should be consulted for a thorough neurological examination.
Precise history-taking and physical examination of a patient with lumbar syndromes usually reveals not only the diagnosis but also the specific level that is affected. Plain radiographs, blood and CSF analyses, myelography, EMG, CT, MRI, and other tests are used to confirm the diagnosis and exclude other diseases.
Neurophysiologic Testing—EMG
The general remarks on neurophysiologic testing on the cervical spine (Chapter 9) also apply to the lumbar spine. Because lumbar nerve root compression syndromes are common and often produce paresis of variable severity, neurophysiologic testing in the lumbar area can be very useful, particularly EMG. The physician and the patient want to know to what extent the nerve root is already damaged, how long this damage has been present, and whether it can be expected to improve. These questions arise particularly in the follow-up evaluation of patients with mild to moderately severe weakness, who now increasingly tend to be treated conservatively. In contrast to a routine primary diagnostic assessment and clinical follow-up, EMG still involves a relatively invasive procedure with multiple needle-sticks. Muscle action potentials are recorded with two or more concentric needle electrodes inserted about 1 cm apart, in a transverse orientation with respect to the muscle fibers. Recordings must be made at various depths and at multiple insertion sites. EMG should therefore only be used in directed fashion and to answer specific questions (Dvořák and Haldeman 2004).
In the lumbar region EMG is most often used for the diagnostic assessment of dorsiflexor and quadriceps weakness, because the findings may imply the need for surgery. Patients suffering from nothing more than a pins-and-needles paresthesia and/or weakness of unimportant muscles, such as the extensor hallucis longus, should be spared the unpleasantness of an EMG and instead followed up clinically with serial assessment of muscle strength.
Plain Radiographs
Just as in the cervical spine, plain AP and lateral lumbar spine views mainly serve the purpose of ruling out other diseases. Disk protrusions, prolapses, and slackening all take place in the radiologically transparent intervertebral space and therefore can only be visualized with special techniques involving the injection of contrast medium.
Degenerative disk changes can be recognized by the narrowing of the intervertebral space and the bony reaction of the neighboring vertebral bodies. Plain radiographs of older patients reveal narrowing of the disk spaces, which may be very marked, as well as osteosclerotic changes of the upper and lower end plates, with hook-like osteophytes at the vertebral body edges that fuse with one another to form bony bridges. These newly formed bony structures initially jut horizontally outward from the vertebral body margin for a greater or lesser distance and then take a vertical course; if a similar osteophyte grows from the vertebral body on the opposite side of the disk, the intervertebral space at this level can be bridged by bone. These bony struts are usually located ventrally and laterally. Only in exceptional cases does a dorsal osteophyte derived from an old disk prolapse protrude into the spinal canal or the intervertebral foramen.
Spondylotic and osteochondrotic changes are documentary evidence of earlier disk slackening rather than of a currently active disk syndrome.
Torgeson and Dotter (1976) obtained plain lumbar spine radiographs of more than 300 patients with lumbar syndrome and compared them with radiographs of a control group of asymptomatic individuals. The frequency of spondylosis was the same in the two groups. Wansor and Fleischhauer (1986), in our institution, came to the same conclusion. Other comparable findings in the literature include those of Bogduk (2000), Nachemson and Jonsson (2000), Jarvik and Deyo (2002), Borenstein et al. (2004), and Van et al. (2006). An evaluation of our patient population by Strickstrack (1983) revealed an increased prevalence of degenerative changes in the upper lumbar segments in individuals over the age of 40 without any associated clinical manifestations.
Despite this, the interpretation of plain radiographs remains important, as it is necessary to recognize not just infection and tumors of the lumbar spine but also pre-discotic structural abnormalities such as axial deviation, asymmetrical transitional vertebrae, juvenile developmental anomalies, abnormalities of the laminae, and narrowing of the spinal canal, all of which predispose to the development of intervertebral disk disease.
In the AP view, one first counts the lumbar vertebrae and notes any transitional vertebrae, which are of clinical importance only in the case of an asymmetrically anchored lumbosacral vertebra causing asymmetrical stress on the disks lying above it (see p. 28). One should also note whether the upper and lower end plates are parallel, as they should be, and inspect the shape and location of the oval shadows representing the pedicles seen on end. In torsion scoliosis, for example, these are projected toward the concave side, and they may be blurry or absent if the pedicles are involved by a neoplastic or infectious process.
Disk collapse and lumbar hyperlordosis cause adjacent spinous processes of the middle and upper lumbar spine to touch one another, leading to reactive sclerosis and, sometimes, clinical symptoms (Baastrup disease) (Fig. 11.50).
The width and borders of the interlaminar windows are of importance for epidural and perineural injections and can be seen in their projections on an AP view, so that the physician can determine the optimal puncture site in advance. Spina bifida occulta, if present, should be mentioned in the radiography report for the sake of completeness but is of no biomechanical significance for the motion segment or for the generation of disk-related symptoms. The lateral view of the lumbar spine is more informative than the AP view (Fig. 11.51).
Loss of the normal lordosis (i.e. abnormal straightening) of the lumbar spine is called the Güntz phenomenon.
Just as in the cervical spine, straightening of the lumbar spine cannot always be interpreted as evidence of an acute disk syndrome, because the normal lordosis can also be abolished voluntarily. When radiographs are taken with the patient lying on one side and flexing the hip and knee joints, as is often done, the lumbar lordosis is often considerably reduced in any case. Findings of greater significance include marked narrowing of the intervertebral spaces in young adults; lateral, rotational, or dorsoventral displacement of the vertebral bodies with respect to one another without interruption of the articular processes (pseudospondylolisthesis in the terminology of Schmorl and Junghanns 1968); and exophytes on the posterior edge of the vertebral column.
If such changes are found in the plain lateral radiographic view, one should proceed to obtain oblique, spot, and tomographic views. Particularly when there is a question of spondylolysis and spondylolisthesis, oblique views should not be omitted. These also show intervertebral joint arthrosis, with characteristic telescoping of the joints due to degenerative disk collapse (Fig. 11.52).
The notochordal remnants and juvenile developmental anomalies to which so much attention is often paid are actually clinically unimportant and imply nothing more than possible weakness of the structures bordering the intervertebral disks, which might create a predisposition to disk disease. Not every protrusion seen in the posterior silhouette of the vertebral column on a plain lateral radiograph is a dorsal osteophyte; a large lateral osteophyte may also be projected over the spinal canal (Fig. 11.53). This situation can only be clarified with a CT or MRI scan.
Fig. 11.52 Arthrosis of the spine with telescoping of the joint surfaces (arrows) due to disk degeneration.
Lumbar Myelography
Myelography is the visualization of the spinal sub-arachnoid space with contrast medium.
The lumbar subarachnoid space is a compartment filled with cerebrospinal fluid (CSF) that is contained between the arachnoid membrane lying just under the spinal dura mater and the pia mater surrounding the spinal cord and nerve roots. The space between the spinal dura mater and the arachnoid membrane is only virtual under normal circumstances. The dural sac containing the subarachnoid space extends caudally to the S2 vertebral level. In the lumbar region, the CSF of the sub-arachnoid space surrounds the nerve rootlets of the cauda equina; CSF continues to surround the nerve roots in nerve root sleeves that extend into the intervertebral foramina.
![]() | |
![]() | |
![]() | |
![]() | |
![]() | |
![]() | |
![]() |
Myelography has become a much less important diagnostic technique for the assessment of disk disease since the introduction of CT and MRI. It is now possible to display the CSF-containing spaces with MR myelography in much the same way that they were displayed with conventional myelography. Furthermore, MRI displays the soft tissues neighboring the CSF spaces, including disk protrusions and prolapses, which myelography does not. Thus, myelography is currently used only for a narrow range of indications, e.g., when a patient suffers from acute cauda equine syndrome and all lumbar segments must be examined radiographically but an MRI scanner is not available.
The combination of myelography with post-myelographic CT has also largely fallen out of use because of the improved display of intraspinal structures with MRI. Practically the only advantage that myelography has over other procedures is that CSF is always obtained as a part of the procedure and can be analyzed in the laboratory for the differential diagnosis of a variety of neurological diseases.
Fig. 11.53 Large lateral osteophytes mimicking a dorsal osteophyte in the lateral radiographic view.
In conclusion, myelography has largely gone out of use, being supeseded by CT and MRI, in accordance with the MIRACLE principle stated in Chapter 1. In comparison with these newer techniques, it is more invasive, has a greater risk of complications, and generally provides less information, at least in the diagnostic evaluation of disk disease.
CT
CT generates cross-sectional radiological images in the horizontal plane with the aid of computer technology. An roentgen ray tube rotates around the patient’s body and obtains pictures from many different angles, which are then manipulated by computer to create a cross-sectional image.
Indications
Although CT is a noninvasive diagnostic technique it is quite expensive and exposes patients to ionizing radiation. A CT scan should therefore be obtained only when there is suspicion of a space-occupying lesion in the lumbar spinal canal and should be restricted to the region of suspicion, i.e., as few levels as possible. Thus, the radiologist should be informed at what level the pathological process is expected to be found. Patients with disk-related radicular sciatica usually have a lesion in the L4–S1 segments. Imaging of higher segments is indicated in patients with upper lumbar root syndromes, polyradicular manifestations, suspected tumor or spondylitis, or cauda equina syndrome. MRI displays lumbar disk prolapses and protrusions much better than CT; if MRI scanning is available, the remaining indications for CT include all bony changes of the lumbar spine, including fracture, infection (spondylitis), and bony tumors, which CT displays better than MRI.
In combination with discography (“disco-CT”), CT can still be used to advantage in the diagnostic evaluation of disk protrusions and subligamentous prolapses. A disco-CT can be helpful in differential diagnosis, particularly for the choice of the optimal intradiscal therapeutic technique (Table 11.2). Its main disadvantage is that the invasive procedure of disk puncture has to be carried out twice: once for diagnostic purposes and then later, after evaluation of the CT images, for therapeutic purposes in a second sitting. A CT scan of the lumbar spine can also be useful as part of a comprehensive assessment in medicolegal cases, if warranted by the clinical findings.
The findings of degenerative spinal change that are revealed by CT have the same significance as those revealed by plain radiographs and myelography: these radiological findings are of no pathological significance and do not imply a need for surgical intervention except when they are closely correlated with the patient’s clinical manifestations.
Treatment of any kind, including surgical treatment, should never be based on radiological findings alone, and certainly not on the findings of myelography, CT, or MRI when these lack clinical correlation.
Technical Procedure
Positioning. A CT scan of the lumbar spine is obtained with the patient supine. Roentgen rays emitted by rotating tubes, oriented in all radial directions perpendicular to the long axis of the body, are sensed by detectors placed opposite the tubes. The resulting data are processed by computer to generate a cross-sectional image in the transverse (horizontal) plane. The lumbar spine should be as straight as possible, i.e., the lumbar lordosis should be reduced as much as possible, so that the planes of section will be parallel to the vertebral bodies and disks. This is achieved by positioning the patient with the knees flexed and the pelvis tilted backward (Fig. 11.54). This method of compensating for the lumbar lordosis is not adequate at the L5/S1 level, for which one can additionally tilt the imaging plane by 20° by tilting the gantry of the CT scanner.
Once the patient is properly positioned, the position must be maintained for the duration of scanning, which takes a few minutes, so that the individual cross-sectional images will correspond correctly to the planes of section indicated on the scout view or topogram of the lumbar spine that is obtained at the beginning. The planes indicated by lines in the scout view are numbered with the numbers of the corresponding transverse (horizontal) images in the study.
The sequence of cross-sectional images and their interpretation. The distance between cross-sectional images can be chosen at will; the images should be more closely spaced at levels near the intervertebral disks than elsewhere. The mid-level of the vertebral body at the height of the pedicles is generally not imaged, to reduce cost and radiation exposure, even though free fragments can be found at this level, just as at other levels.
The cross-sectional images generated by computer are always displayed in the same way (Fig. 11.55).
The CT images are always displayed as if one were looking at the patient from below, i.e., in the caudocranial direction.
Fig. 11.54 The patient is supine with gently elevated legs to neutralize the lumbar lordosis for the lower lumbar CT scan.
Fig. 11.55a, b The lower lumbar CT scan is displayed as if one were looking at the patient from the feet upward, as seen in (a). Thus, the right side of the body appears on the left side of the radiological image (b).
Thus, the patient’s right side is always seen on the left side of the image. Because the lumbosacral junction contains structures that are visually distinct and easy to recognize (the sacrum and ilium), the sequence of images is generally best viewed from the bottom up, i.e., from caudal to cranial.
It is advisable to refer to the L5/S1 disk seen in a CT scan as the “last” intervertebral disk and to the L4/5 disk as the “second-last” one to avoid any possible confusion, particularly when intradiscal therapy or disk surgery is to be performed.
Otherwise, the presence of transitional vertebrae may lead to inconsistent numbering. When the image intensifier is used in the operating room, it is safe and easy to image the sacrum first and then count the intervertebral spaces from the bottom up.
The Normal CT Scan
The interpretation of CT scans is similar to that of conventional radiographs. In a CT image, as on a plain radiograph, thick (cortical) bone appears white and fat or air appears dark. All shades of gray are possible in between. The vertebral body spongiosa has a salt-and-pepper appearance. Disk tissue, the dural sac, and the nerve roots usually all appear equally gray to the naked eye and can thus be told apart only by their configuration. They are separated from one another by the intervening epidural fat.
Before searching in detail for pathoanatomical findings in the spinal canal on a cross-sectional CT image, one must first ascertain the segment, and the level within the segment, that the image represents. The lateral scout view (topogram), on which the individual cross-sections are indicated and numbered, can be of assistance here, informing the examiner whether the plane of section passes through a vertebral body, a disk, or (in the case of L5/S1) both.
Even without looking at the scout view, however, the examiner should still be able to determine the level of a CT section of the lumbar spine within the particular segment at which it was obtained. Sections in which the intervertebral foramina are not seen can easily be distinguished from those in which they appear as lateral openings in the vertebral arch. The images displaying the intervertebral foramina are clinically important because they show the immediate vicinity of the intervertebral disk.
On all sequences of CT images of the lumbar spine, it is important to follow the nerve root from its site of origin from the dural sac all the way to its exit from the spinal canal through the neural (intervertebral) foramen.
Displacement of the nerve root, or invisibility of the nerve root because of impingement from adjacent pathological structures, is of clinical significance. A disk protrusion that does not impinge on a nerve root cannot be the cause of sciatica.
The CT scan demonstrates the entire course of the nerve roots and clearly shows that protrusions and prolapses of the lower two lumbar disks can often impinge on the L5 and S1 nerve roots at the same time. Thus, for example, a large paramedian L4/5 disk prolapse can impinge on the L5 root from the medial side and simultaneously impinge on the S1 root from the lateral side just as it emerges from the dural sac, or while it still lies intrathecally. A similar situation arises with a lateral L5/S1 disk herniation, which can push the L5 root outward and the S1 root inward.
Anatomical variants, including conjoined roots and variable origin of the nerve roots from the dural sac, can produce unusual clinical and radiological situations. A preoperative imaging study—CT, myelogram, or MRI—is therefore essential in every case, even if the segmental symptoms and signs appear to be unequivocal.
Protrusions and prolapses of lumbar disks. Modern CT scanners have high enough resolution to enable displaced disk tissue in the spinal canal to be recognized and differentiated from the neighboring soft tissues. The contours of the disk are at their most pronounced where there is a large amount of epidural fat in the vicinity, e.g., behind the L5/S1 disk.
Disk protrusions and prolapses can be recognized in the lumen of the spinal canal either because of their typical localization and configuration or through the manner in which they impinge on and distort the neighboring dural sac, epidural fat, and nerve roots. Disk tissue is usually more radiodense than the lumbar CSF, though these two tissues sometimes appear isodense to the human eye. Isodense soft tissues in the spinal canal are more commonly seen in CT images produced by older scanners with poorer resolution, or else when the spinal canal is narrowed, so that the relatively radiodense rootlets of the cauda equina are not surrounded by much CSF, epidural fat is largely absent, and the dural sac is adjacent to the bony wall of the vertebral canal on all sides.
Signs of tissue displacement. Displaced disk tissue always pushes some of the ventral epidural fat aside, producing an asymmetrical configuration of the fat. It must be borne in mind, however, that epidural fat may be absent, e.g., because of spinal canal stenosis (see below), postoperative adhesions and scoliosis, or simply advanced age. The most important radiological finding is the displacement or loss of contour of a nerve root together with indentation of the dural sac. The differential diagnosis includes asymmetry of the nerve root origins from the dural sac and scoliosis causing the CT plane to be angulated rather than truly transverse in relation to the spine.
The site and extent of the dislocated disk tissue must be precisely specified with information in all three dimensions. Stating the level of the disk fragment specifies the horizontal plane in which it lies; its horizontal distance from the midline determines its location in the frontal plane; and the extent to which it protrudes into the spinal canal is its size in the sagittal plane. Inspection of the images at multiple levels reveals whether a prolapse is more displaced in the more cranial or more caudal direction. The precise depiction of the displaced disk tissue on the CT scan tells the surgeon how far to explore within the spinal canal to remove all of the expelled disk fragments.
An indirect sign of nerve root compression is thickening of the nerve root shadow at the affected level and at more caudal levels. Inspection of the course of the nerve roots in a series of transverse sections often reveals asymmetrical nerve root diameter over multiple levels.
When dislocated disk tissue protrudes into the spinal canal, the indication for surgery or intradiscal treatment depends to a large extent on whether the finding is a protrusion with a preserved anulus fibrosus or a prolapse perforating the outer layer of the fibrous border of the disk. Discography provides an unequivocal answer to this question, but CT can also be very helpful. Disk protrusions have a symmetrical base and a greater ratio of base width to height than prolapses; prolapses, on the other hand, jut further into the epidural space from a relatively narrow base and tend to have an irregular surface with a pointed tip, producing a triangular outline (Fig. 11.56).
Fig. 11.56 CT criteria for distinguishing disk protrusions (with intact anulus fibrosus) from disk prolapses (with perforated anulus fibrosus).
The periphery of a protrusion may contain sickle-shaped areas of calcification representing the initial stage of ossification of the posterior longitudinal ligament. In a young patient, this finding represents the bony margin of the vertebral body, which has been undermined and lifted up by disk tissue.
A prolapse can be displaced cranially or caudally, either wholly or in part, and can be seen on transverse sections at vertebral body levels, but this is not the case for a simple disk protrusion.
The CT appearance of subligamentous fragments is intermediate between that of protrusions and prolapses. Subligamentous fragments can be treated by intradiscal methods.
Even very broad prolapses seen on CT do not necessarily require invasive treatment. Their management should always be based on the clinical findings (Figs. 11.57–11.62).
Fig. 11.57a–c Clinical findings: 42-year-old man with S1 sciatica for the last 12 weeks, Lasègue sign positive on the left at 30°, crossed Lasègue sign positive on the right at 60°, pain and band-like hypesthesia in a left S1 distribution, diminished left Achilles reflex, no motor deficit. CT: broad-based protrusion of disk tissue (grade II dislocation) at the L5/S1level, with left paramedial indentation of the dural sac. Treatment: conservative.
Fig. 11.58 a–f Clinical findings: 51-year-old woman with severe, persistent left-sided sciatica for the last 6 weeks, Lasègue sign positive at 40°, Achilles reflex absent on the left, pain and band-like hypesthesia in the left S1 distribution, grade 3 paresis of left plantar flexors. CT: a free fragment lying paramedially in the epidural space on the left side is seen at the L5/S1 level (dislocation grade V); it extends caudally to the upper edge of the S1 vertebral body. The two S1 roots can be seen again ventral to the dural sac only at the mid-S1 level; even here, the left S1 root is still somewhat dorsally displaced. Treatment: surgery. A caudally displaced free fragment, hard and bean-sized, was found in the axilla of the S1 nerve root (see Fig. 11.58 e).
Fig. 11.59 a–c Clinical findings: 37-year-old man with massive contralateral sciatic postural abnormality for the last 5 weeks. Band-like pain in an L5 distribution, mild weakness of the left extensor hallucis longus. CT: broad-based protrusion of the L4/5 disk at the discal level. The protrusion is broader than it is deep and is mainly located paramedially on the left side. Disk protrusion, grade II dislocation. Treatment: conservative.
Fig. 11.60 a–f Clinical findings: 39-year-old woman with left S1 sciatica, diminished Achilles reflex on the left, positive Lasègue sign at 60°, band of pain and hypesthesia in a left S1 distribution, band-like pain partly in an L5 distribution as well. CT: broad-based L5/S1 disk protrusion extending into the intervertebral foramen (grade II dislocation), displacing the S1 root dorsally and also impinging on the exiting left L5 nerve root laterally in the foramen (→). In the more caudal images, the left S1 root is less severely displaced. This root is thickened in comparison to its counterpart on the right. Treatment: conservative.
Fig. 11.61a–c Clinical findings: 39-year-old man with L5 sciatica for the last 4 months and an acute exacerbation for the last 6 weeks. Grade III dorsiflexor paresis on the left, Lasègue sign positive at 20°, ipsilateral postural abnormality, absent left Achilles reflex, band of pain in an L5 and S1 distribution, hypesthesia of the entire dorsum of the foot, no cauda equina symptoms or signs. CT: large fragment at the L4/5 discal level obliterating the left L5 root and compressing the dural sac to half of its normal cross-sectional area. Treatment: immediate surgery. A prolapse the size of a fingertip, partly free and partly still under the posterior longitudinal ligament, was found deep in the axilla of the L5 root (grade IV dislocation).
Postoperative CT
Even though CT scanning technology is now quite advanced, postoperative scans remain hard to interpret. One reason is the limited experience that has been gathered in this area to date; another is the fact that a postoperative scan generally contains multiple isodense soft tissue shadows lying next to one another within the spinal canal that are poorly demarcated from each other. In the first few days after disk surgery, CT scanning may be necessary if the original symptoms persist or new ones have arisen. The cause may be a residual or newly expelled disk fragment, hematoma, abscess, or pseudomeningocele due to leakage of CSF from the dural sac.
Fig. 11.62a–e Clinical features: 46-year-old man with very severe left S1 sciatica for the past 5 weeks. Absent Achilles reflex, Lasègue sign positive at 30°, band of pain and hypesthesia in a left S1 distribution, ipsilateral postural abnormality. CT: extradiscal fragment (grade V dislocation) from the L5/S1 disk extending caudally, seen at its greatest extent in the section just below the L5/S1 disk. The left S1 nerve root is obliterated by the fragment. Additional finding: lateral spinal canal stenosis. Treatment: surgery. Immediately upon opening of the ligamentum flavum at the left L5/S1 level, sequestrated disk material came forward, having worked its way posteriorly past the dura mater and nerve root to just under the ligamentum flavum. Postoperative CT: status post prolapse removal and widening of the lateral recess (→). The patient has been asymptomatic ever since the operation. Collections of serous fluid in the wound, the dural sac, and the nerve root together create an isodense shadow on the left side of the spinal canal; this is a normal postoperative finding.
Collections of blood or CSF can usually be recognized by their rounded shape and distinguished from one another by density measurements in Hounsfield units: for example, the CSF-filled cavity of a pseudomeningocele has the same radiodensity as water.
Scar tissue in the epidural space is recognizable as a grayish mass with irregular borders adjacent to the disk, posterior edge or the vertebral body, and/or lamina. The configuration of the dura mater and nerve root is asymmetrical. Often, the nerve root can no longer be seen on the operated side, because it is enveloped in scar tissue. The most important sign of scar tissue is the absence of epidural fat.
After hemilaminectomy, the laminar contour is interrupted on one side. In this case, the scar tissue runs uninterruptedly from the dural sac to the muscles of the back.
When a fat pad has been placed in the wound before surgical closure, the postoperative scar tissue is interrupted by a more or less broad, rounded area that appears dark (i.e., with the density of fat). The CT findings after fat-pad insertion have been described by Schroeder (1982), Burton (1983), and Krämer and Köster (2001), among others.
The difficulty of interpreting postoperative CT scans lies in the fact that nerve roots, disks, and scar tissue all have similar radiodensity. Thus, a recurrent disk prolapse may hide within the apparently uniform gray shadow of extensive postoperative scarring. The extent of impingement can then only be recognized indirectly by the distortion of the dural sac.
A further means of distinguishing different types of soft tissue from one another in CT scans is the systemic (i.e., intravenous) administration of contrast medium. The theoretical basis for this is the fact that well-perfused tissue will take up contrast medium to a greater extent than tissue that is only poorly perfused or not at all, such as a fresh disk prolapse. Contrast-enhanced CT scans are mainly useful for the detection of recurrent disk prolapse within peridural scar tissue. Fresh, well-perfused scar tissue naturally takes up more contrast medium than old, fibrotic sheets of scar in which old disk-derived tissue has become incorporated.
In view of these difficulties, one should not be too quick to use postoperative CT to diagnose recurrent disk prolapses, in order not to cause further undue worry in patients who have just undergone disk surgery but still have symptoms. Most of these symptoms are due to scarring and adhesions rather than new prolapses. Every new operation only makes this situation worse.
Summary: CT
In CT scanning, roentgen rays are used to produce cross-sectional images in the transverse plane. A roentgen ray tube rotating around the patient obtains data in each plane of section that are then synthesized into images by computer. Even though CT does not distinguish different types of soft tissue as well as MRI, it is superior to MRI for the examination of bony structures. For example, it enables a clear differentiation of disk tissue from calcification or ossification (the latter may be the residua of old disk prolapses). In addition to its limited ability to tell different types of soft tissue apart, further drawbacks of CT include the imaging of only part of the lumbar spine and the associated radiation exposure, which is especially high in repeated so-called CT-guided injections of the lumbar spine.
Diagnostic Study of the CSF
Additional studies of other types are done mainly for differential diagnostic purposes. These include laboratory tests such as the complete blood count, CRP, and electrophoresis, which show no pathological changes in lumbar syndrome. Whenever myelography is carried out, CSF is obtained as a by-product and should always be sent to the laboratory for analysis.
In patients with lumbar disk prolapse, the CSF is of normal appearance (clear) and flows rapidly through a thin spinal needle.
If blockage of CSF flow is suspected a Queckenstedt test can be done, but this is not part of the routine examination in lumbar syndrome. The CSF laboratory findings in lumbar syndrome are usually normal. When the lumbar nerve roots are subject to continuous, intense irritation from a large prolapse, or in post-discotomy syndrome, one may sometimes find a mildly increased protein concentration, though the cell count remains normal.
A markedly elevated protein concentration combined with a normal cell count and normal CSF opening pressure is called albuminocytologic dissociation. This is a classic finding in Guillain–Barré syndrome but can also be seen in spinal irritative conditions of many other types and is thus nonspecific. In such cases, both the total protein content and the colloid curves display high values.
The analysis of CSF contributes very little to the positive demonstration of a disk prolapse and is therefore used mainly for the exclusion of other potential causes of the clinical syndrome, e.g., infectious processes (herpes zoster, syphilis) or tumors.
Pathological elevation of the protein concentration and cell count, or obstructed flow as revealed by the Queckenstedt test, is an indication for further diagnostic testing.
Discography
After initial enthusiasm for this diagnostic technique (Erlacher 1949, Lindblom 1951, 1969, Witt 1951), lumbar discography fell into almost total disuse for a long period, because it was recognized that pathological configurations of contrast medium in the interior of the disk lacked clinical significance. Not every degenerated disk produces symptoms. Moreover, myelography with the newly developed contrast media proved to be much more informative. Only in the last few years have publications on lumbar discography begun to reappear, though each new publication seems to emphasize different aspects of the interpretation of the findings (Hugdins 1977, Crock 1983, Fraser et al. 1983, Park 1985, Sullivan 1985, Schleberger et al. 1986, Sachs et al. 1987, Mooney 1988, Weinstein et al. 1988, Braithwaite 1998, Ito 1998, McCulloch 1998, Carragee 1999, 2000, 2002, 2006, Lam 2000, Nachemson and Jonsson 2000, Herkowicz 2004, Böhm et al. 2005). A meta-analysis by Ahn (2006) shows that the role of discography remains controversial in the work-up of patients with low back pain and degenerative disk disease. A lumbar discogram is considered to be positive, with reference to the indication for surgery, only when the contrast medium that is injected into the interior of the disk flows out dorsally into the epidural space (reflux) or when a disk fragment is found in the dorsal portion of the anulus fibrosus, in some cases protruding out of it. All other discographic findings are designated as negative, even when severe degenerative changes are found.
The interpretation of discograms focuses on the question whether a disk prolapse or protrusion is present.
The diagnostic accuracy of lumbar discography, when it is employed in this way, is 83% (Hudgins 1977) (Fig. 11.63). Discography is also a useful supplement to CT scanning in many cases, because it provides special information about the location and size of any dorsally displaced disk fragments. Discography yields information about the disease process beyond the findings that can be appreciated visually on radiographs: if the disk is closed (not perforated), the injection pressure and injectable volume of fluid indicate the size of the intradiscal cavity system and the extent of disk degeneration (Fig. 11.64). Injection of the affected disk generally reproduces or intensifies the patient’s typical radiating pain, similarly to the cervical spine distension test (memory pain).
Fig. 11.63 Schematic classification of discographic findings and indications for intradiscal treatment and surgery.
Fig. 11.64 Discography at L4/5 reveals a totally degenerated disk with a dorsally displaced fragment, type 7. No epidural outflow of contrast medium. If this finding correlates with the clinical findings, intradiscal therapy is indicated.
The interpretation of contrast medium patterns. In general, a lateral radiographic view suffices to show whether the contrast medium injected into the disk remains in the center of the disk or flows out of it through a narrow connecting pathway into the epidural space. To localize a fragment more precisely, additional oblique views are needed (Fig. 11.65). The contrast medium injected into the interior of the disk spreads out in different patterns depending on the size and type of the intradiscal cavity system. The contrast medium depot may appear rounded or branch out irregularly toward the periphery. These intradiscal contrast medium patterns are of little clinical importance, however, because from a certain age onward nearly all lumbar disks display degenerative tears and branching of greater or lesser severity. The only findings that should truly be considered pathological with reference to a possible indication for surgery are contrast medium reflux into the epidural space and the presence of a tissue fragment.
A tissue fragment is defined as a piece of tissue around which contrast medium flows but which is not itself filled with contrast medium and lies at the dorsal edge of the anulus fibrosus, possibly protruding outward from it.
Such fragments are often covered by a thin layer composed of the outer lamellae of the anulus fibrosus, the posterior longitudinal ligament, and/or the ventral epidural membrane. The hernia-like sac around the fragment may take up so much contrast medium that the fragment itself can no longer be seen.
Corresponding to the diverse pathoanatomical operative findings in patients with lumbar disk prolapses, discography can reveal almost any conceivable pattern of contrast medium, a fact that makes interpretation rather difficult (Fig. 11.66). If the lateral and oblique radiographs after discography do not provide sufficient information about the site of a disk fragment, a CT scan of the affected segment can be obtained about 2 h later (so-called CT discography). This technique is especially suitable for the demonstration of intraforaminal protrusions (Jackson and Glah 1987, Kornberg 1987, Schulitz et al. 1988).
Fig. 11.65a, b Discography at L5/S1 reveals a subligamentous (→) fragment, type 8. If this finding correlates with the clinical findings, intradiscal therapy is indicated. Even when the tip of the needle is placed at the edge of the disk, the intervertebral space can be filled with contrast medium, and thus also with the therapeutic agent.
Fig. 11.66 Discography at L4/5 with type 9 findings. A large amount of contrast medium immediately flows into the epidural space.
MRI
Synonyms: MRT (magnetic resonance tomography), NMR (nuclear magnetic resonance).
Physical principles underlying MRI. When a strong external magnetic field is applied to the body, atoms within the body that have a magnetic moment orient themselves in the direction of the field. When the external field is then switched off, the “relaxation” of the atoms oriented along it is accompanied by the emission of electromagnetic radiation that can be detected and measured outside the body. The patient must lie still for a relatively long time inside a fairly narrow tube containing a strong magnetic field while the images are produced with the aid of computer technology. Images can be generated in any imaging plane. Unlike CT, MRI does not involve the use of ionizing radiation.
Technical procedure. Just as in CT, the patient lies supine with slightly flexed legs and must remain in the same position throughout the study. The scout scan and sectional images in the transverse plane are analogous to their CT counterparts. The patient’s right side is always on the left side of the image (cf. Fig. 11.54, p. 173).
Advantages. MRI is the diagnostic imaging method of choice for lumbar disk disease because it enables imaging of the entire lumbar spine, in multiple planes of section, with better differentiation of various types of soft tissue from one another than CT. A particular advantage is the absolute harmlessness of the technique, which has now been fully documented.
MRI is the only technique used to diagnose lumbar disk prolapses that does not employ roentgen rays.
The advantage of MRI for disk surgery is that it can be used to generate images of all of the lumbar motion segments in a single study. It is thus much harder to overlook dislocated disk fragments or simultaneous disk prolapses at other levels in an MRI scan as compared to CT, which is routinely used to image only two or three lumbar segments at a time.
MRI | CT |
---|---|
No ionizing radiation | Ionizing radiation |
Time-consuming study | Quick study |
All segments | Routinely only 2–3 segments |
All planes | Only the axial plane |
High contrast resolution for disk tissue | Low contrast resolution for disk tissue |
Very expensive | Expensive |
![]() |
![]() |
![]() |
The ability to show images in any plane of section at will (sagittal, coronal, horizontal, or selective demonstration of foraminal planes) permits the precise localization of dislocated fragments and osseous nerve root compression.
The resolution of the technique is such that prolapsed disk tissue and the dural sac are well demarcated from one another. The indentation of the dural sac by a disk prolapse resembles what is seen in a lateral myelographic image. Furthermore, the prolapsed tissue is seen in fine detail. Well-hydrated nucleus pulposus tissue has a high signal intensity, equivalent to that of CSF, in T2-weighted images. Portions of the anulus fibrosus, the cartilaginous end plates, and the posterior longitudinal ligament have a low signal intensity in all signal weightings because of their low water content (Table 11.3).
The water content of the disk prolapse or protrusion is an important determinant of the optimal form of treatment, and of the prognosis.
A well-hydrated free fragment in the epidural space can be treated conservatively at first, as long as no major neurologic deficit is present, because it can be expected to shrink rapidly in volume now that the nucleus pulposus tissue within it is no longer in contact with the intradiscal osmotic system. On the other hand, hard, cartilaginous fragments, which usually produce severe pain, are unlikely to shrink over time and one should not wait too long before removing these surgically.
In addition, MRI enables quantitative assessment of degenerative changes of the disks, particularly their water content. We have found that the human intervertebral disk contains more water in the morning than in the evening. This finding reveals the operation of an osmotic system within the disks (see Chapter 4).
We know from pathoanatomical studies and clinical experience in discography that disk degeneration is not synonymous with disk-related symptoms. Findings on MRI within 12 weeks of the inception of serious low back pain are highly unlikely to represent any new structural change (Carragee 2006). Thus, it is wrong to attach too much importance to the radiological finding of degenerative dehydration of disk tissue with consequent bulging of the anulus fibrosus. On the contrary, the hypointense, poorly hydrated degenerated disks seen on an MRI scan (“black disks”) bode fewer problems for the future than the bright, well-hydrated disks that still have a normal turgor pressure (see Fig. 11.68).
CT or MRI?
The indications for lumbar spine MRI in disk disease are determined mainly by considerations of availability and cost. The entire diagnostic spectrum can be covered by CT, occasionally supplemented with myelography (Table 11. 4).
The special advantages of MRI have to do with the localization and differentiation of dislocated fragments in theepiduralspace.A surgeon should not operate on a disk without a preoperative MRI scan, particularly if microsurgery is to be done through a small incision. MRI is also much better than CT and myelography at distinguishing postoperative scar tissue from residual or recurrent prolapses. Likewise, it is better for the differential diagnosis of other neurological diseases in the lumbar region. All other questions, particularly those concerning bony structures, are better answered by CT (Table 11.5).
Findings in Disk Degeneration
The strengths of MRI are particularly easy to appreciate when MRI and CT scans of the same patient are compared. The surgeon obtains clear information about how far and in what direction the spinal canal must be explored so that all sequestrated fragments can be removed. The designation of locations in the lumbar and epidural space with a conventional scheme of levels and zones enables radiologists and surgeons to communicate effectively and unambiguously about the MRI findings. In addition to precise localization, MRI also yields information about the consistency and water content of disk tissue. When interpreting MRI scans, however, the clinician must be aware that the perifocal edema surrounding dislocated pieces of disk tissue makes the degree of impingement on neighboring structures appear more extensive, and more impressive, than it really is. Medial protrusions and prolapses seen in sagittal MRI views often seem to occupy the entire spinal canal.
MRI exaggerates the true findings in disk prolapse.
The clinical findings above all determine the choice of therapy, no matter how impressive the MRI findings may be.
The pathoanatomical changes in the intervertebral space are displayed better on MRI than on plain radiographs or CT scans. Loss of water within disk tissue, leading to fibrosis and sclerosis, is revealed as loss of signal. Recent improvements in MRI technology make it possible to visualize individual structures within the intervertebral space, e.g., fragments that have lost their connection to the surrounding tissue, the outer lamellae of the anulus fibrosus, and ligamentous structures. Protrusions can be distinguished from prolapses. Discography is very rarely needed now to answer these questions.
Fig. 11.67 Modic classification, including differential diagnosis vs. spondylodiscitis (from Linhardt and Grifka in Wirth and Zichner 2004).
The Modic Classification
Beyond the changes in the disk, there are also corresponding changes in the neighboring vertebral bodies, expressed not only as spondylosis and osteochondrosis, which can be seen even in plain radiographs or CT scans, but also as a variable appearance of the spongiosa. The MRI abnormalities of the bone marrow space of the neighboring vertebrae were classified by Modic (1985) into three types, representing stages that cannot always be sharply distinguished from one another (Fig. 11.67):
Type I: ingrowth of vascularized, contrast-enhancing tissue into the neighboring bone marrow, with increased signal intensity on T2-weighted images and decreased signal intensity on T1-weighted images.
Type III: increasing sclerosis and scarring of the bone marrow space with loss of signal in images of all signal weightings.
Type I degenerative changes of the vertebral bodies may resemble spondylodiscitis on MRI. Therefore, if the clinical manifestations and laboratory findings warrant it, conventional tomography, CT, and in some cases diagnostic tissue biopsy for infectious material should be carried out.
The clinical relevance of the degenerative changes classified by Modic is slight. Bone marrow changes are seen on MRI as a normal correlate of age-related, generalized intervertebral disk degeneration. A few publications have appeared in which the authors attempt to correlate the changes described by Modic with certain clinical symptoms (Pfirrmann et al. 2001, Kuisma et al. 2004, 2007, Albert and Manniche 2005, Jones et al. 2005, Kjaer et al. 2005, Modic et al. 2005, Linhardt et al. 2007, O’Connell et al. 2007).
Postoperative MRI
Relatively well-hydrated disk tissue can be more easily distinguished from postoperative scar tissue on a plain radiograph than on a CT scan. In T2-weighted MR images, disk tissue appears bright and thus different from hypointense, poorly vascularized scar tissue. As soon as some of the disk material is degenerated, however, it becomes isointense and is no longer so easy to distinguish from scar tissue. In such cases, intravenous contrast medium must be used, just as for a CT scan. Clinical experience has shown that diagnostic accuracy can be improved by comparing T1-weighted images obtained before and after the injection of contrast medium (Peters et al. 1990, Krämer and Köster 2001). Even this method, however, does not eliminate all differential diagnostic problems, because granulation tissue that is histologically identical to epidural granulation tissue may grow from the end plates into the disks as part of the degenerative process.
Further discussion of postoperative CT vs. MRI can be found on pages 286 ff. and 290 ff.
MRI Findings without Symptoms
Disk protrusions, signs of degeneration with black disks, signal changes in the vertebral bodies, and postoperative scarring on the MRI scan need not necessarily be accompanied by corresponding symptoms. Often, such MRI findings are found in segments neighboring a disk prolapse as radiologic correlates of asymptomatic disk degeneration, as the following examples will show. When a patient presents with a nerve root compression syndrome and the MRI scan shows abnormal findings in multiple segments, it is usually only one segment that is responsible for the clinical manifestations. Other diagnostic techniques in addition to MRI must be used to localize the origin of the pain, e.g., a discogram revealing “memory pain,” a nerve root block, or an EMG.
Reports in the literature. There are many published reports of extensive degenerative changes seen on MRI in individuals who had no symptoms at all, or who at least had none at the time the MRI was obtained. Boden et al. (1990), for example, found a disk protrusion or prolapse in 20% of individuals under age 60 and 36% of those over age 60. In their longitudinal study of asymptomatic individuals, Borenstein et al. (1998) found that degenerative changes seen on MRI have no predictive value for future low back pain. Further studies documenting pathological radiological findings in asymptomatic individuals include those of Bozzao et al. (1992), Buirski and Silberstein (1993), Carragee et al. (2000), Krämer and Köster (2001), Krappel and Harland (2001), and Assheuer (2002).
Case Illustrations (from Krämer and Köster 2001)
1. L2/3 Protrusion and Prolapse, MRI and CT (Fig. 11.68)
Clinical presentation. This 44-year-old man reported feeling a sudden snap in his back while getting up from a low chair 4 weeks ago, and thereafter severe back pain radiating into the lateral aspect of both thighs. The pain persisted despite 3 weeks of conservative treatment, and he was admitted to hospital. Examination revealed a sciatic postural deformity with the torso inclined slightly to the right. There were no motor deficits, and the deep tendon reflexes were normal. The Lasègue sign was positive on the right at 30°.
MRI and CT. These study sequences are shown in Fig. 11.68.
Findings. The L3/4 and L5/S1 intervertebral discs are of markedly diminished signal intensity in the T2 image. There is a broad-based, asymmetrical posterior displacement of disk tissue of relatively high signal intensity at level L2/3 (c, →), which compresses on the dural sac (a–c). There is also a circumscribed posterior displacement of disk tissue in the median zone at the L5/S1 level (a, b, d), without compression the dural sac. The CT findings at L5/S1 are consistent with the MRI findings, but the CT additionally shows clear evidence of calcification or ossification (e, →).
Fig. 11.68a–f Study sequences (from Krämer and Köster 2001).
a T2 TSE, sagittal, median.
b T1 SE, sagittal, median.
c T2 TSE, axial, L2/L3.
d T2 TSE, axial, L5/S1.
e, f CT, axial, L5/S1 (e) and superior end plate of S1 (f).
Note. Bony structures in the L5/S1 segment that are seen on the CT scan cannot be visualized by MRI with the sequences shown in this case.
Treatment. Hospitalization was recommended for conservative treatment consisting of relaxation supine with the hips and knees flexed, a flexion orthosis, and local injections.
Clinical course. The pain improved steadily under conservative treatment. After 3 months there was only mild residual sacral pain, without radiation into the legs.
Comments. The subligamentous paramedial protrusion in segment L2/3 produced the clinical symptoms in this case. Compression involved only the intrathecal portions of the spinal nerves, and for this reason the pain did not radiate further down the legs. The broad-based protrusion seen in the axial images is consistent with an intact anulus fibrosus. The protrusion at L5/S1 is not clinically significant: both CT and MRI show that it makes no contact with the neural elements.
2. L5/S1 Protrusion and Prolapse, Modic II Signs (Fig. 11.69)
Clinical presentation. This 40-year-old man complained of low back pain and left-sided sciatica of 3 months’ duration. The band of pain corresponded to the left S1 segment. The pain was of moderate intensity; he was able to sleep at night, and he felt pain during the day only with certain movements. Examination revealed a diminished left Achilles tendon reflex and a positive Lasègue sign on the left at 60°.
MRI. The study sequences are shown in Fig. 11.69.
Findings. Disk degeneration is present in the last two segments, which are of diminished signal intensity and diminished height (a, b). Marked changes (Modic type II) are seen in the superior and inferior end plates at L4/5. The L4/5 disk and especially the L5/S1 disk (a–c) are posteriorly displaced, narrowing the left caudal intervertebral foramen (e, →). The subligamentous portions of the disk are of relatively high signal intensity in the T2 image (e, →). The left S1 nerve root is thickened (a, b, f, →).
Diagnosis. Broad-based disk protrusion at L4/5 (dislocation grade II) and prolapse at L5/S1 (dislocation grade V).
Treatment. Because the pain was relatively mild, conservative treatment was provided.
Clinical course. The treatment included epidural perineural injections on the left side in segment L5/S1, cube positioning (with hips and knees flexed), and physiotherapy. The symptoms improved, and the patient returned to his office job.
Comments. Neither the clinical findings nor the MRI findings are particularly severe. Some of the denser tissue in the left lateral recess of S1 corresponds to the left S1 nerve root, which is thickened because of edema. Modic type II changes of the upper and lower end plates of L4/5, of no clinical importance.
3. L4/5, Prolapse with Supradiscal Extension, Modic II Signs (Fig. 11.70)
Clinical presentation. This 61-year-old man had suffered from back pain, with occasional radiation into the legs, for several years. In the week leading up to presentation, the pain had become increasingly severe and radiated into the right leg in an L4 and L5 dermatomal pattern, with some radiation into the anterior aspect of the thigh as well. Physical examination revealed limping on the right side due to pain and a positive Lasègue sign on the right at 60°. The right Achilles tendon reflex and patellar reflex were slightly diminished.
MRI. The study sequences are shown in Fig. 11.70.
Findings. The last two intervertebral discs are of diminished height and signal intensity on the T1- and T2-weighted images, and there is a zone of increased signal intensity in the end plates (a, b). There is disk tissue lying posterior to the L4 vertebra (a, b, arrowhead) and retaining only tenuous contact with the intervertebral disk (a, →). This tissue fills the entire right L4 lateral recess (c) and masks the right L4 nerve root (→ = left L4 nerve root). The MIP reconstruction (d) shows the topographic relationship of the prolapse (P), the L4 nerve root (→), and the L5 nerve root (→).
Diagnosis. Right paramedial L4/5 disk prolapse with supradiscal fragment (dislocation grade V). Osteochondrosis at L4/5 and L5/S1 with Modic type II bone marrow changes.
Treatment. Surgery was recommended because of the severe pain and the massive fragment, despite the absence of a motor deficit.
Operative findings. Half of the inferior margin of the L4 lamina was removed to reveal a massive free fragment compressing the intrathecal portion of the L5 nerve root medially and the exiting L4 nerve root laterally. The L4/5 intervertebral space was so narrow that it did not admit insertion of a disk rongeur.
Fig. 11.69 a–f Study sequences (from Krämer and Köster 2001).
a, b T2 TSE, sagittal, left paramedian/paramedial (a) and left paramedial/lateral (b).
c, d T1 SE, sagittal, left paramedian/paramedial (c) and left paramedial/lateral (d).
e, f T2 TSE, axial, L5/S1 (e) and superior end plate (f).
Fig. 11.70 a–d Study sequences (from Krämer and Köster 2001).
a T2 TSE, sagittal, right paramedial.
b T1 SE, sagittal, right paramedial.
c T2 TSE, axial, middle third of the L4 vertebral body.
d FISP 3D, oblique sagittal MIP reconstruction.
Clinical course. There was almost no leg pain after surgery. At the 3 month follow-up examination there was still no leg pain, but the back pain remained at its preoperative background level.
Comments. The massive fragment had separated from the parent disk at L4/5 and migrated craniolaterally, where it compressed both the intrathecal portion of the L5 nerve root and the exiting L4 nerve root. This produced a band of pain in the L4 and L5 dermatomes. Surgical intervention was deemed necessary because of the severe pain and the large size of the fragment.
4. L3/4, Prolapse with Supradiscal Extension and Modic I Signs (Fig. 11.71)
Clinical presentation. This 52-year-old man complained of severe back pain of 3 weeks’ duration. The pain had initially radiated into the anterior aspect of his right thigh, but then radiated laterally in a band corresponding to the proximal portion of the right L5 dermatome. A marked ipsilateral sciatic postural deformity was present (i.e., the torso was inclined to the right). The Lasègue sign was positive at 30° on both sides.
EMG. There was evidence of nerve root irritation at the L3 and L4 levels, without any sign of florid denervation or a functionally relevant deficit.
MRI. The study sequences are shown in Fig. 11.71.
Findings. The lowest three intervertebral discs are of diminished signal intensity. A circumscribed subligamentous posterior displacement of disk tissue is seen at the L3/4 level, and the annuloligamentous complex (→) is well demarcated. The extruded disk tissue extends cranially as far as the middle of the posterior margin of the L3 vertebral body and is continuous with the parent disk (a, b). The dural sac is markedly compressed (a–c). A circumscribed edematous area in the posterobasal portion of the L3 vertebral body (a, c, →) is consistent with Modic type I bone marrow changes.
Fig. 11.71a–d Study sequences (from Krämer and Köster 2001).
a T2 TSE, sagittal, median.
b T1 SE, sagittal, median.
c, d T2 TSE, axial, inferior end plate of vertebra L3 (c) and L3/L4 (d).
Diagnosis. Subligamentous medial and paramedial L3/4 intervertebral disk prolapse with supradiscal extension.
Treatment. Because there was no relevant functional impairment, conservative treatment was provided, consisting of relaxation supine with the hips and knees flexed, epidural perineural injections, physiotherapy, and back school.
Clinical course. Gradual resolution of symptoms with disappearance of leg pain, so that only sacral pain remained. The patient was discharged 2 weeks after surgery and fitted with a Discoflex torso orthosis. At follow-up examination 3 months later, the patient was nearly asymptomatic. Lumbar spine mobility was limited only at the end of the range of motion, and physiotherapy was not needed.
Comments. The L3/4 extrusion at disk level initially caused severe pain referable to the L3 and L4 nerve roots as it migrated into the concavity of the posterior wall of the vertebral body. The pain later became less severe. The extruded fragment was not sequestrated in the epidural space, but rather lay beneath the epidural membrane of the L3 vertebra, as can be seen from its relatively broad-based bulging into the epidural space, with a smooth margin (Fig. 11.71c). There are no nerve roots in the median and paramedian zones of the supra-discal level of the L3/4 segment; a disk prolapse at this level affects only the intrathecal nerve roots.
Fig. 11.72a–d Study sequences (from Krämer and Köster 2001).
a T2 TSE, sagittal, left lateral.
b T1 SE, sagittal, left lateral.
c T1 SE, axial, inferior end plate of vertebra L4.
d T1 SE, axial, inferior end plate of vertebra L4, after IV injection of gadolinium.
5. L4/5, After Previous Surgery, Modic Type II Upper and Lower End-Plate Changes (Fig. 11.72)
Clinical presentation. This 58-year-old woman had undergone disk surgery 2 years previously. Pain in the left leg resolved after surgery, but returned 3 months later and persisted. The pain radiated into the L5 dermatome and occasionally also the S1 dermatome. The Lasègue sign was positive on the left at 30° and on the right at 60°. There was no neurologic deficit.
MRI. The study sequences are shown in Fig. 11.72.
Findings. There is soft tissue formation in the left L4/5 intervertebral foramen, with marked contrast enhancement (c, d, →). The spinal ganglion is only vaguely distinguishable in the contrast-enhanced images (d, →). There is marked dural enhancement, mainly posteriorly (d, →). The sagittal images show a lack of fat signal at the infrapedicular (a, b, →) and infradiscal levels (a, b, →).The L4/5 intervertebral disk is severely degenerated, with accompanying Modic type II degenerative changes in the upper and lower end plates.
Diagnosis. Scarring and granulomatous changes due to previous surgery in the left L4/5 intervertebral foramen and on the posterior aspect of the dura mater. No evidence of recurrent disk prolapse.
Treatment. This patient suffered from postdiscectomy syndrome. Conservative treatment was initially provided, with epidural perineural injections, physiotherapy, and a flexion orthosis.
Clinical course. Conservative treatment yielded no significant improvement. As leg pain was the primary symptom, neurolysis was recommended.
Comments. This patient had a typical postdiscectomy syndrome. There was no evidence of recurrent disk prolapse. Extensive postoperative scarring was present, both in the spinal canal and in the paraspinous musculature. As the radicular symptoms were more prominent than the back pain, neurolysis and fat-pad insertion was preferred to spinal fusion as the surgical treatment.
Fig. 11.73a–c Study sequences (from Krämer and Köster 2001).
a T2 TSE, sagittal, median.
b T1 SE, sagittal, median.
c T2 TSE, axial, L1/L2.
6. L1/2, Protrusion, Multisegmental Degeneration with Modic Type II Changes (Fig. 11.73)
Clinical presentation. This 60-year-old man had suffered from back pain for 20 years. There had been recurrent episodes of severe low back pain, occasionally radiating along either the anterior or the posterior aspect of the thighs.
MRI. The study sequences are shown in Fig. 11.73.
Findings. All of the lumbar intervertebral discs are of markedly diminished signal intensity, with varying reductions in height and disk protrusions of varying severity. There is a marked reduction of disk height at L1/2, where mild retrolisthesis and posterior osteophytes (b, →) are also seen. There are circumscribed areas of Modic type II degeneration in the upper and lower end plates. There is a broad-based posterior displacement of the disk, most severe in the lateral zones, causing marked bilateral stenosis of the intervertebral foramina, while the posterior surface of the disk remains concave (c, →) and the dural sac and the conus medullaris within it are, therefore, not compressed.
Diagnosis. Chronic, broad-based, bilateral disk protrusion at L1/2 with accompanying multisegmental degenerative disk disease.
Treatment. Conservative treatment was recommended, consisting of flattening of the lumbar lordosis (relaxation supine with the hips and knees flexed and a flexion orthosis), epidural injections, and facet infiltrations.
Clinical course. Only moderate improvement under conservative treatment.
Comments. There is disk degeneration in almost all of the lumbar segments; L1/2 is the most severely affected, with protrusion of the anulus fibrosus, but without disk prolapse. Surgical decompression is not advisable, because the symptoms are not localizable to any particular level. Further conservative therapy is recommended. Gradual spontaneous improvement can be expected over the next few years.
Summary: MRI
MRI is a noninvasive imaging technique, not employing roentgen rays, that can be used in the diagnostic assessment of degenerative changes in the spine. Multiplanar visualization and differentiation of different types of soft tissue are the major advantages that have led MRI to be widely adopted in place of certain types of invasive imaging study that were previously used, i.e., discography and myelography. MRI truly provides “all-in-one imaging.”
The precise localization of the pathoanatomical changes causing pain makes precisely directed treatment possible, including directed injection techniques (the province of the pain specialist) and microsurgical removal of the responsible lesion through a small incision. The MRI findings are of clinical importance only when correlated with the patient’s symptoms and signs.
Conclusion
Assessed in terms of the MIRACLE principle (see Chapter 1), MRI is a noninvasive and risk-free but costly diagnostic study that provides much important information.
Clinical Syndromes
Classification of Lumbar Syndromes
Lumbar syndromes can be classified into three categories (Table 11.6): simple low back pain, back and leg pain, and alarming spinal syndromes (Nachemson and Jonsson 2000, Waddell 2004, German Back Pain Guidelines 2006). This threefold classification corresponds to the scheme of the Spine Working Group (now called the Spine Section) of the German Society of Orthopedics and Orthopedic Surgery, which divides lumbar syndromes into local lumbar syndrome, lumbar root syndrome, and differential diagnoses with alarming manifestations (Krämer 2004).
In accordance with this threefold classification, the following sections will deal with
local lumbar syndrome
lumbar root syndrome
the differential diagnosis of low back pain, with discussion of the serious conditions, both intrinsic and extrinsic to the spine, that can cause it.
No precise epidemiologic data are available on the relative frequency of simple and complicated low back pain, back and leg pain, and alarming spinal symptoms. Waddell (1993) estimated that 93% of patients suffer from low back pain alone, 5% from back and leg pain, and 2% from alarming symptoms; similar estimates were given by Bogduk (2000), Casser (2001), and Nachemson (2004). Nonspecific low back pain should not be viewed as a homogenous condition. Outcomes can be improved when subgrouping is used to guide treatment decision-making (Brennan 2006)
Local Lumbar Syndrome, Low Back Pain
Definition: Local lumbar syndrome consists of all clinical manifestations that are directly or indirectly caused by degenerative and functional disturbances of the lumbar motion segments and that are restricted to the lumbar region.
Local lumbar syndrome is largely analogous to local cervical syndrome in terms of its etiology, pathogenesis, and clinical features. All transitional states are possible in local lumbar syndrome, ranging from acute lumbago that suddenly arises and then rapidly abates to chronic, recurrent low back pain. It would be terminologically imprecise to refer to all of these conditions as “lumbago,” as lumbago is only one of the possible manifestations of local lumbar syndrome.
Local lumbar syndrome is characterized by positionally dependent low back pain, spasm of the lumbar erector spinae muscles, and restricted range of motion of the lumbar spine. There is no segmental radiation of pain into the lower limbs (Table 11.7). The symptoms arise from degenerative changes of the lower lumbar motion segments leading to mechanical irritation of the posterior longitudinal ligament, the intervertebral joint capsules, and the spinal periosteum. The sensory fibers of the meningeal and dorsal branches of the spinal nerve are mainly affected. Reflex spasm of the erector spinae muscles of the back is unpleasant and painful. These muscles are innervated by the dorsal branches of the lumbosacral spinal nerves. It is not possible to identify the particular segment that is involved when pain arises through irritation of a dorsal branch, unlike when it arises through irritation of a ventral branch (sciatica).
![]() |
![]() |
![]() |
![]() |
Local lumbar syndromes are by far the commonest type of disk-related symptom in the entire spine. They are even more frequent than local cervical syndromes. Patients with only mild local lumbar syndrome (so-called simple low back pain) rarely consult a physician because their symptoms resolve spontaneously in a short time and respond well to treatments that are commonly known to the general public, such as the application of heat of any type and suitable positioning. Only when the pain is very severe, lasts longer than usual, or recurs frequently and at ever shorter intervals does the patient start to worry and seek medical help.
Classification. Local lumbar syndromes are subdivided into acute and chronic types based on the pattern of major symptoms. It is of therapeutic importance whether the patient is suffering from discogenic lumbago or from chronic low back pain of arthroligamentous origin. The most important clues to the diagnosis are to be found in the clinical history, as in all disk-related conditions.
Lumbago, Discogenic Back Pain
Lumbago is an acute type of local lumbar syndrome. All of the major sympoms of local lumbar syndrome are accentuated and appear suddenly.
The history often includes an unexpected mechanical stress on the spine, such as bending and lifting; heat and dampness are often also mentioned. Immediate-onset low back pain usually leads to instant immobility of the low back, which freezes in a characteristic abnormal posture. In order to maintain this locked position, which is the only position in which the pain is reduced, there is reflexive, strong contraction of the lumbar erector spinae muscles. Any attempt to move actively or passively out of the locked abnormal position causes severe pain. The patient fears and avoids any movement and also reports that coughing, sneezing, or pressing worsens the pain.
The main cause of lumbago is intradiscal tissue displacement with irritation of the posterior longitudinal ligament (cf. Fig. 11.27a). The main zone of pain is located in the lower lumbar region and over the sacrum, either in the midline or somewhat lateral to it. The pain may also spread diffusely, on an autonomic basis, in the ventral or cranial direction. There may be pseudoradicular radiation of pain to the thigh muscles (cf. Fig. 8.5). In addition to lumbago of sudden onset associated with a particular movement, there is also a rarer form that gradually increases in severity and that takes a few hours to reach its peak.
The major finding on clinical examination is the abnormal, rigidly held posture of the lumbar spine (Fig. 11.74). Flexion of the trunk is impossible; any flexion that the patient may be able to carry out occurs only at the hip joints, while the lumbar spine remains completely rigid. The patient can rise from the examining table only with a characteristic lateral rolling movement, using the buttocks as a fulcrum.
The lower lumbar spinal processes are tender to deep pressure and percussion. Straight-leg raising in the supine position worsens low back pain. There are no positive neurological findings.
The abnormal posture depends on the site of the lesion; it may consist of a rigid extension (kyphosis) of the lumbar spine combined with inclination to the right or left side. This abnormal posture is also the only positive radiological finding. When stress is taken off the low back, i.e., when the patient lies with the hips and knees lightly flexed, the pain is usually absent and the abnormal posture disappears.
It is mainly younger patients who suffer from lumbago, because their intervertebral disks are more likely to undergo intradiscal tissue displacement.
The attacks of pain are usually of brief duration at first and tend to resolve spontaneously. Their further course is unpredictable. Attacks of lumbago in youth and middle age may remain the only manifestation of disk degeneration. Often, however, they represent the beginning of a chronic, recurrent lumbar disk syndrome that expresses itself in its further course with frequent attacks of low back pain and sciatica.
Fig. 11.74 Typical postural abnormality in lumbago. Mild inclination of the trunk (antalgic kyphosis). Marked spasm of the paravertrebral muscles limiting movement of the lumbar spine.
Low Back Pain of Arthroligamentous Origin
Characteristics and etiology. Long-lasting and recurrent low back pain constitutes the chronic form of local lumbar syndrome. The major symptoms of local lumbar syndrome are often of only mild intensity, arise gradually, and subside slowly. Low back pain of arthroligamentous origin can be regularly provoked by certain positions, e.g., prolonged sitting, standing, or sometimes even lying, and ameliorated by changes of position. Lumbago, in contrast, is of sudden onset and is not induced or ameliorated by positional changes. Chronic, recurrent low back pain is usually thought to be due to changes of the elasticity and volume of the lumbar disks with secondary effects on the joints, ligaments and muscles. Because these manifestations arise only when disk degeneration has reached an advanced stage, chronic low back pain generally arises only after age 35–40. Depending on the position that induces it, the pain can be designated as low back pain in flexion or in extension, or else as lumbar kyphosis pain or low back pain in hyperextension (facet syndrome).
Facet Syndrome
Facet syndrome is low back pain arising mainly from the lumbar intervertebral joint facets. It may radiate into the lower limbs, but not in a radicular pattern.
Symptoms arising from the intervertebral joints were comprehensively described as early as 1936 by Lange in his monograph entitled Die Wirbelgelenke (“the intervertebral joints”) (Lange 1936). The condition was usually referred to as “spondylarthrosis” in the German-speaking countries till the adoption of the term “facet syndrome,” which was coined by Mooney and Robertson (1976) and then entered into international use (Schwarzer et al. 1994, 1995, Bogduk 2000, Herkowicz 2004, Jerosch and Steinleitner 2005). Neuroanatomical studies on the subject were carried out by Kiessling (1993) and by Murata et al. (2000).
Most facet syndromes are stress-related, with symptoms worsening over the course of the day. The pain disappears when the patient lies flat with mildly flexed hips and knees (Helbig and Lee 1988). In many individuals, postural weakness while standing leads to hyperlordosis of the spine that becomes painful in a very short time. In this position, the facet joints are subject to excessive stress, and the spinal canal and the intervertebral foramina are relatively narrow. The low back pain may even be associated with radicular symptoms (see Fig. 11.39). Low back pain in hyperlordosis arises after prolonged walking and standing, particularly when the individual wears high-heeled shoes, indirectly producing forward tilting of the pelvis and hyperlordosis of the spine. The pain of hyperlordosis can also be induced or exacerbated when the lumbar lordosis is increased by downhill walking or by activities requiring the individual to lean backward, e.g., hanging up laundry, looking at pictures, or any type of work above the head.
Other types of stress-related pain are due to gradual loss of height of the intervertebral disks and fatigue of the truncal musculature over the course of the day. The disks become less elastic as they shrink. The remaining components of the lumbar motion segments, mainly the intervertebral joints and ligaments, are subject to excessive or inappropriate stress and give rise to pain mediated by the meningeal branch of the spinal nerve.
The pain of facet syndrome originates in the lumbar intervertebral joints and is felt in the low back with radiation into the buttocks, groin, lower abdomen, thighs, and occasionally the scrotum. Patients describe it as diffuse and widespread and demonstrate its localization by laying the hand flat over the affected area, unlike patients with radicular syndromes, who can draw the borders of the affected dermatome with one finger. Examination reveals tenderness to shaking and percussion over the affected segments. Not all of the intervertebral joints are affected to the same extent, and the diagnostic assessment must therefore include segmental examination, with testing for pain on rotation, flexion, and extension of the lumbar spine (Fig. 11.75).
The Sacroiliac Joint and Sacroiliac Joint Syndrome
The sacroiliac joint can be considered functionally and neuroanatomically as the lowest pair of facets. Kiessling (1993) studied the neuroanatomy of the sacroiliac joint and found a pattern of innervation resembling that of the lumbar intervertebral joints. Spontaneous pain and tenderness to pressure can be found on the iliac crest, at the greater trochanter, and in the groin over the origin of the iliopsoas muscle.
A special test of the sacroiliac joint consists of palpation of the posterior iliac spine during movement to check for the so-called forward bending phenomenon. Normally, the two posterior iliac spines are at the same height both before the patient bends forward and afterward. A difference in height at the end of forward flexion is considered pathological (Fig. 11.76).
The diagnosis of facet pain or sacroiliac joint pain is strongly suggested by the generation of pain in the low back and leg with the so-called “figure 4” maneuver, in which the lumbar spine is passively brought into lordosis when the examiner maximally abducts and externally rotates the patient’s hip (Fig. 11.77). The diagnosis is further confirmed by the reduction or elimination of the pain upon injection of local anesthetic into the capsule of the affected joint (facet infiltration, see p. 243).
Facet syndrome and sacroiliac joint syndrome with stress-related low back pain have a fluctuating course. The frequency and intensity of the pain depend on bodily activity and posture: patients can largely prevent the emergence of symptoms by regularly alternating between sitting, standing, and lying positions and avoiding abrupt movements. On the other hand, biochemical and biomechanical involutional processes in disk tissue that are still poorly understood can cause the symptoms to arise and subside suddenly and unpredictably, because any change in the disk necessarily brings about an altered position of the intervertebral joints.
Fig. 11.75 a, b The search for a segmental irritation point in the lumbar spine (kyphosis sensitivity) (a). Testing for functional segmental irritation in the lumbar spine with testing of lordosis sensitivity (b) (Bischoff 1994, from Rubenthaler et al. 2005).
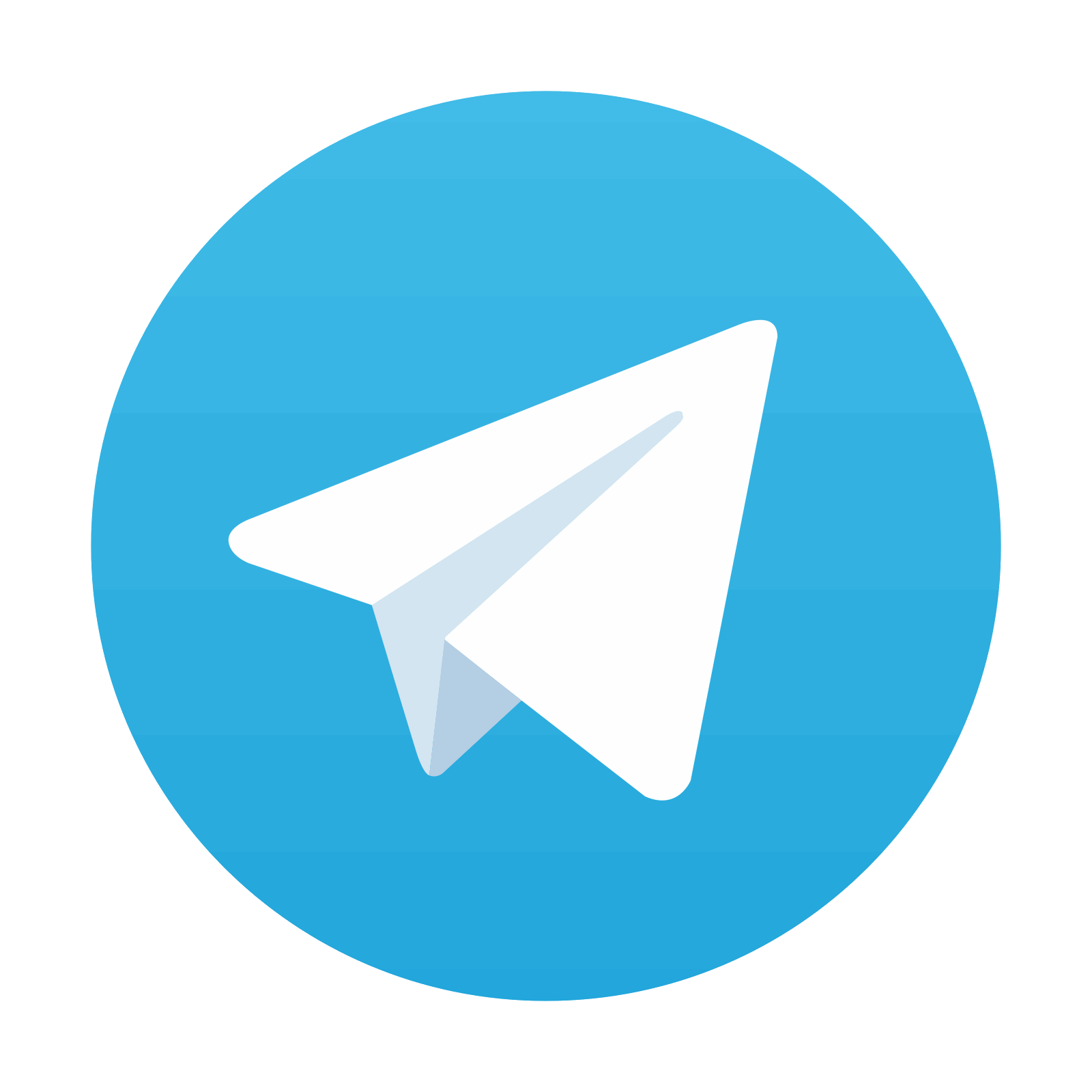
Stay updated, free articles. Join our Telegram channel

Full access? Get Clinical Tree
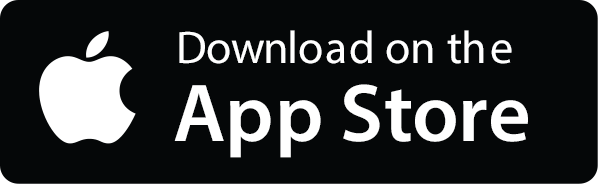
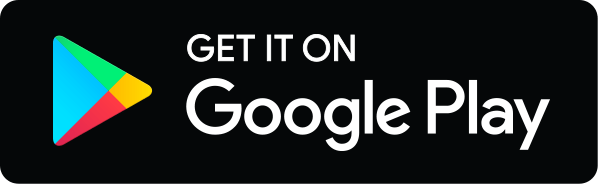