Chapter objectives
- •
Identify activities that may cause detrimental stress on a healing/reconstructed anterior cruciate ligament, posterior cruciate ligament, medial collateral ligament, or lateral collateral ligament.
- •
Identify activities that may cause detrimental stress on the patellofemoral joint.
- •
Develop appropriate rehabilitation programs for athletes with a variety of knee injuries.
- •
Determine when to advance an athlete’s rehabilitation program by using specific measurable criteria.
- •
Discuss the reliability, sensitivity, and specificity of arthrometry, lower extremity functional tests, and isokinetics.
- •
Interpret information obtained from an arthrometer, lower extremity functional tests, and measures of strength.
The knee joint is one of the most frequently injured joints in the body, especially in those engaging in athletic activity. In the functional anatomy section of this chapter some of the key information used for developing safe and effective rehabilitation programs is presented. The rehabilitation programs are goal oriented, modified by time instead of being driven by it. Also emphasized are knee rehabilitation of the entire kinetic chain, early controlled motion, return to participation along a functional progression, and restoration of lower extremity muscular strength, power, endurance, and neuromuscular control.
Functional anatomy
To make appropriate clinical decisions for rehabilitation of knee injuries, the clinician must have a thorough understanding of lower extremity anatomy and biomechanics. In the following sections some of the more important biomechanical factors related to rehabilitation of knee injuries are presented.
Ligaments
The knee is inherently unstable because of its location between the two longest bones in the body. Knee stability is maintained through static restraints (e.g., ligaments) and dynamic restraints (e.g., muscles). The role of the ligamentous restraints in controlling forces applied to the knee joint has been studied extensively. Loads produced on the knee by rehabilitation activities have been the subject of a number of investigations. The data gained from these studies provide the clinician with the information necessary to develop safe and effective rehabilitation programs.
Anterior Cruciate Ligament
The anterior cruciate ligament (ACL) is the primary restraint to anterior translation of the tibia on the femur. Grood et al reported that the ACL provides 85% of the ligamentous restraining force to an anterior drawer test at 30° and 90° of flexion. In addition to controlling anterior tibial translation, the ACL has several other functions, including the screw-home mechanism, assisting in the control of varus and valgus stress, control of hyperextension stress, and a guiding function during tibiofemoral flexion-extension. Because of its position in the femoral intercondylar notch, if a valgus stress is placed on a flexed knee, the ACL becomes a restraint to external tibial rotation. The ACL also assists the medial collateral ligament (MCL) in controlling tibial internal rotation.
The stresses placed on the ACL by rehabilitation exercises have been studied in a number of investigations. Henning et al implanted a strain gauge in two patients with grade II ACL sprains. The patients then performed various rehabilitation activities and the strain was recorded. The strain on the ACL was reported as a percentage of the strain of an 80-lb Lachman test ( Table 19-1 ). Although conclusions should be drawn with care from a study with only two subjects, it still provides some useful information about the relative rank of strain on the ACL during particular rehabilitation activities.
Activity | Relative ACL Strain (%) * |
---|---|
Running downhill at 5 mph | 125 |
Isometric quadriceps contraction at 22° of flexion against a 20-lb weight | 62-121 |
Isometric quadriceps contraction at 0° of flexion against a 20-lb weight | 87-107 |
Jogging on the floor | 89 |
Lift the leg in 22° of knee flexion | 12-79 |
Jogging 5 mph on a treadmill | 62-64 |
Isometric quadriceps contraction at 45° of flexion against a 20-lb weight | 50 |
Walking without an assistive device | 36 |
Half-squat, one leg | 21 |
Quad set | 18 |
Walking with crutches, weight bearing at 50 lb | 7 |
Stationary cycle | 7 |
Isometric hamstring contraction | − 7 |
* A single recording indicates that the activity was reported for one subject only, whereas a range indicates recordings reported for both subjects.
Beynnon et al implanted a Hall effect transducer in the knees of 11 subjects with normal ACLs and then determined the strain on the ACL during open chain knee flexion and extension, as well as during isometric contractions. They concluded that the following open chain exercises produce either low or no strain on the ACL: isometric contractions of the hamstrings at 15°, 30°, 60°, and 90°; isometric quadriceps contractions at 60° and 90°; cocontractions of the quadriceps and hamstrings at 30°, 60°, and 90°; active knee flexion and extension between 35° and 90°; and knee flexion and extension with a 45-N (10-lb) weight between 45° and 90°. Exercises that proved to significantly increase strain on the ACL included the following: knee extension exercise with a 45-N weight (particularly at 10° and 20° of knee flexion), isometric quadriceps contractions (at 15° and 30°), and isometric cocontractions of the quadriceps and hamstrings at 15°. During the knee extension exercise the transition from unstrained ACL to strained ACL shifted from 35° of flexion during active unweighted knee extension to 45° during the weighted knee extension condition.
Beynnon et al also compared closed chain with open chain exercises for peak strain on the ACL. They reported the following peak ACL strains: open chain active knee flexion-extension with no load, 2.8%; squatting, 3.6%; open chain knee flexion-extension with a 45-N load, 3.8%; squatting with a sport cord, 4.0%; and 30-Nm isometric quadriceps contractions at 15°, 4.4%. To put these ACL strain values into clinical perspective, these authors reported the peak ACL strain to be 3.7% for a 150-N Lachman test and 1.7% for stationary bicycling. The results of this study regarding the influence of open chain quadriceps activity on ACL strain are predictable from the results of other studies, but the strain results during the closed chain activities are somewhat surprising given that Escamilla et al reported no tensile load on the ACL during loaded squats or leg presses.
Kvist and Gillquist measured anterior tibial translation during knee extension exercises and several types of squats. During active knee extension, as loads increased, so did tibial translation, with the ACL-deficient knee demonstrating greater anterior translation than the uninjured knee. Anterior translation was greater during the eccentric phase than during the concentric phase of the exercise. The greatest anterior translation occurred between 15° and 20° of knee flexion. This matches the range in which Escamilla et al reported the greatest tensile load on the ACL during weighted open chain knee extension. Kvist and Gillquist also reported that anterior translation was less under all squat conditions than during loaded open chain extension exercises in knees with deficient ACLs. Similar results were reported in two different studies by Yack et al.
In most studies investigating anterior shear during rehabilitation activities the results have been reported as strain on the ACL or anterior translation. A few researchers have calculated their results relative to body weight. Table 19-2 contains such data.
Reference | Activity | Knee Position at Peak Anterior Shear | Calculated Force Times Body Weight |
---|---|---|---|
Ericson and Nisell | Cycling at 60 RPM, 120-W workload | 60°-70° | 0.05 |
Kaufman et al | Isokinetic knee extension at 60°/sec | 25° | 0.3 |
Isokinetic knee extension at 180°/sec | 25° | 0.2 | |
Nisell et al | Isokinetic knee extension at 30°/sec | 45° | 1.3 |
Isokinetic knee extension at 180°/sec | 40° | 0.5 |
Posterior Cruciate Ligament
The primary function of the posterior cruciate ligament (PCL) is to limit posterior translation of the tibia on the femur. It also assists in controlling varus, valgus, and hyperextension stress on the knee. The role of the PCL in controlling rotational force appears to be minimal. The importance of the PCL in normal knee arthrokinematics is indicated by the increased compression force observed in the patellofemoral joint and medial compartment in cadaveric specimens when the PCL is sectioned. This correlates with the common complaints of anterior knee pain and medial compartment arthrosis in patients with a PCL-deficient knee. Rehabilitation activities that cause large posterior shear force include isometric hamstring contractions, jogging, lunges, ascending and descending stairs, and squats involving greater than 60° of knee flexion (especially as hip flexion is increased). In addition, during early PCL rehabilitation, the clinician may want to avoid activities that require high force from the gastrocnemius because of evidence that these activities may produce significant strain on the PCL. Isokinetic testing of the hamstrings also produces significant posterior shear force on the tibiofemoral joint. Box 19-1 contains a summary of exercises that may cause excessive stress during the early healing phase after ACL or PCL reconstruction. Table 19-3 contains calculated posterior shear data for several rehabilitation exercises.
ACL
Loaded open chain knee extension from 50° to 5°
PCL
Open chain hamstring exercises in any part of the range of motion
Loaded open chain knee extension from 90° to 50°
Closed chain activities with the knee in greater than 50° of flexion
Reference | Activity | Knee Position at Peak Posterior Shear | Calculated Force Times Body Weight |
---|---|---|---|
Ericson and Nisell | Cycling at 60 RPM, 120-W workload | 105° | 0.05 |
Kaufman et al | Isokinetic knee flexion at 60°/sec | 75° | 1.7 |
Isokinetic knee flexion at 180°/sec | 75° | 1.4 | |
Ohkoshi et al | Squat | 15° | < 0.25 |
30° | < 0.3 | ||
60° | 0.25-0.5 | ||
90° | 1.0-1.25 |
Collateral Ligaments
The MCL is the primary restraint against valgus stress on the knee. It has a role in control of internal rotation torsional force through the knee, but this role decreases as the knee is flexed. The MCL also assists in controlling excessive external rotation. The MCL is taut in extension and external rotation. Consequently, as flexion is initiated, tension within the ligament assists in reversal of the screw-home mechanism. Rehabilitation activities that stress the MCL would include any hip adductor strengthening exercises in which resistance is placed distal to the knee. In addition, care must be taken during closed kinetic chain activities if the athlete lacks hip control because the hip has a tendency in these conditions to adduct and internally rotate, which creates valgus stress on the knee.
The lateral collateral ligament (LCL) is the primary restraint to varus stress on the knee. The tendon of the biceps femoris overlaps the LCL and may act as an active mechanism to bias the tension of the LCL. The LCL, along with the posterolateral capsule, appears to play a large role in control of external tibial rotation. Rehabilitation activities that stress the LCL would include any hip abductor strengthening exercises in which resistance is placed distal to the knee.
Capsular Restraints
The posterior medial and lateral “corners” of the knee joint capsule play an important role in the control of torsional force through the knee. The posteromedial capsule is supported by the semimembranosus muscle and the oblique popliteal ligament, which is an expansion of the tendon of the semimembranosus. The posterior medial corner provides some restraint to valgus stress when the knee is in extension, but it is primarily involved in the control of internal torsional stress.
The posterolateral capsule is reinforced by the arcuate ligament and the popliteus tendon. The posterolateral corner, with the LCL, is primarily involved in control of external rotation stress; however, in an ACL-deficient knee, it also plays a role in controlling internal rotation of the knee. The posterolateral corner plays a minor role in control of varus stress on the knee when the knee is extended. The posterior capsule is less prone to injury when the knee is in the flexed position because the structure is relatively slack.
In vitro isolated sectioning of the ACL or PCL is not generally associated with rotational instability, but when coupled with sectioning of either of the posterior corners, rotational instability becomes apparent. Clinically, this would suggest that an athlete with acute rotational instability probably does not have an isolated cruciate injury.
Menisci
The meniscus serves a number of important functions, including increasing the stability and congruence of the knee joint, load distribution and transmission, shock absorption, joint proprioception, and aiding in joint lubrication and nutrition. Its shape, attachments, and collagen arrangement allow the meniscus to effectively transmit compressive force across the tibiofemoral joint. Removal of the meniscus reduces the contact area between the femur and tibia, which substantially increases the force per unit area between the two articular surfaces. It is likely that the increase in force per unit area leads to the degenerative changes that often occur after removal of the meniscus. The structure and attachments of the menisci also allow early controlled weight bearing after longitudinal meniscal repairs because the sutured edges are approximated by weight-bearing stress.
The peripheral 10% to 30% of the meniscus has a vascular supply from the perimeniscal capillary plexus. The rest of the meniscus, 70% or more, receives nutrition by passive diffusion and mechanical pumping (intermittent compression during joint loading and unloading). Because of the important role that the meniscus plays in the health of the knee joint, meniscal tears in the vascular zone are usually repaired surgically. By extrapolation from animal studies, the tensile strength of the meniscus 12 weeks after repair is approximately 80%.
The menisci move or distort during tibiofemoral motion, distorting posteriorly during flexion and anteriorly during extension. This distortion is caused by a shear from the oblique reaction force between the femur and meniscus. During active flexion, contraction of the semimembranosus and popliteus muscles also assists in pulling the menisci posteriorly. This is why open chain hamstring strengthening is usually avoided in the early rehabilitation phases after meniscal repairs. During internal and external rotation of the knee, the menisci distort in opposite directions. With internal rotation of the femur on the tibia, the medial meniscus distorts posteriorly, whereas the lateral meniscus distorts anteriorly. The opposite occurs during external rotation of the femur on the tibia. In summary, during flexion and extension of the knee, the direction of meniscal distortion follows the direction of movement of the tibial plateau, and during rotation the distortion follows the direction of movement of the femoral condyle. This means that during combined flexion-extension and internal-external rotation movements, the distortions in the anterior and posterior portions of one meniscus are in opposite directions, whereas in the other meniscus the distortion is primarily in one direction. This is why a frequent mechanism of isolated meniscal injury is combined knee flexion and rotation.
Patellofemoral biomechanics
It is important to understand patellofemoral biomechanics when one prescribes knee exercises, regardless of the diagnosis. The connection between the tibiofemoral and patellofemoral joints must not be overlooked, nor should these joints be treated independently. Ignoring important aspects of the biomechanics of the patellofemoral joint during rehabilitation of a tibiofemoral joint problem often creates patellofemoral joint problems and unnecessarily extends the rehabilitation process.
Stability of the patellofemoral joint is based on the interplay among bony geometry, ligamentous-retinacular restraints, and muscles. To function optimally, the patellofemoral joint must be able to control forces in the sagittal and frontal planes. Additionally, the hip and foot must control transverse plane forces through the patellofemoral joint. Three factors play an important role in the sagittal plane mechanics of the patellofemoral joint: the quadriceps force of contraction, the sagittal plane angle of the knee, and the contact area between the patella and femur. The interaction between the force of the quadriceps contraction and the knee angle determines the amount of compressive force that occurs between the patella and femur. The compression force is known as the patellofemoral joint reaction (PFJR) force. Increasing the quadriceps force of contraction or increasing the knee angle increases PFJR force ( Fig. 19-1 ). It is important to note that at knee angles of less than 25° to 30°, even large quadriceps forces do not produce tremendous compression force because the magnitude of the posteriorly directed resultant force vector is sufficiently reduced. During most rehabilitation activities, gravity has a profound influence on the force of the quadriceps contraction. As the torque of gravity increases during an exercise, the force of the quadriceps must increase. For typical open chain quadriceps exercises, as the knee moves toward extension, the torque of gravity increases and therefore the force of the quadriceps must increase. The opposite is true for common closed chain activities: the torque of gravity decreases as the knee moves toward extension and thus the force of the quadriceps decreases as well.

The third factor to consider in sagittal plane forces is the contact area between the patella and femur. The contact area depends on the contact points between the patella and femur, which change based on the tibiofemoral joint flexion angle ( Fig. 19-2 ). In general, the contact area between the articular surfaces of the patella and femur increases as knee flexion progresses toward 90°. Because the patellofemoral contact area varies, it is important to account for it when the influence of the PFJR force is examined. This relationship, PFJR force applied per unit area of contact, is known as patellofemoral contact stress.

During typical open chain quadriceps exercises, as the knee extends, patellofemoral joint contact stress increases significantly because of the increasing PFJR force and decreasing contact area. Experimentally, it appears that during open chain quadriceps exercises, maximal contact stress peaks at approximately 35° to 40°. It then declines as extension continues because of the reduced sagittal plane angle of the knee. During typical closed chain exercises, as the knee extends, the patellofemoral joint contact stress decreases despite the decreasing contact area. This occurs because PFJR force is decreasing rapidly as a result of the decreasing torque of gravity and decreasing sagittal plane angle of the knee. Patellofemoral contact stress is also influenced by patellar position in the frontal and transverse planes. Inappropriate alignment in these planes can produce a nonuniform pressure distribution with higher peak stress in some areas and relative unloading in others.
Figure 19-3 is a graphic representation of data from several studies of patellofemoral contact stress versus knee flexion angle during both open and closed chain activities. The lines for open and closed chain contact stress cross at approximately 50°. These data suggest that for an athlete with an extensor mechanism problem, open chain strengthening activities for the quadriceps are safest from 90° to 50° and from 10° to 0°, whereas closed chain activities are safest from 50° to 0°. These “safe” and “unsafe” ranges should be used only as a guide, with the truly detrimental ranges and loads being determined by the signs and symptoms of the athlete. Initially, athletes with extensor mechanism problems will often experience an increase in pain and symptoms if exercises are performed in the suggested unsafe ranges. However, as the athlete’s condition improves, loads through greater ranges, including the suggested unsafe ranges, will typically be tolerated without symptoms. Decisions on when to advance the exercise program should be based on the signs and symptoms of the athlete. Most athletic endeavors will require the athlete to tolerate loads through a wide range of motion (ROM) under a wide variety of situations. Therefore, in the late stages of the rehabilitation process, it is often necessary for the athlete to perform activities in the unsafe ranges if these activities are to be tolerated on return to sport.

Table 19-4 contains calculated PFJR force data for a variety of rehabilitation activities. Care must be taken when interpreting the data in this table because they do not account for the contact area through which the PFJR force is being applied. Direct comparison should be made only between activities with peak compression force at similar angles of knee flexion and therefore similar contact area. For example, a comparison between knee extension with a 9-kg weighted boot and cycling, which appear to have similar PFJR force values (1.4 and 1.3, respectively), would be misleading. Because the patellofemoral contact area is greater at 83° than at 36°, patellofemoral joint contact stress will be much greater during the knee extension exercise. Box 19-2 provides a summary of activities with high and low patellofemoral contact pressure.
Reference | Activity | Knee Position at Peak Patellofemoral Compressive Force | Calculated Force Times Body Weight |
---|---|---|---|
Dahlkvist et al | Squat, slow ascent | 45° | 4.73 |
Squat, slow descent | — | 7.41 | |
Squat, fast ascent | 55°-60° | 5.99 | |
Squat, fast descent | 60° | 7.62 | |
Ericson and Nisell | Cycling at 60 RPM, 120-W workload | 83° | 1.3 |
Flynn and Soutas-Little | Forward running | 35% of stance phase | 5.6 |
Backward running | 52% of stance phase | 3.0 | |
Huberti and Hayes | Squat | 90° | 6.5 |
Kaufman et al | Isokinetic knee extension at 60°/sec | 70° | 5.1 |
Isokinetic knee extension at 180°/sec | 80° | 4.9 | |
Reilly and Martens | Walking | 8° | 0.5 |
Straight leg raise | 0° | 0.5 | |
Knee extension with a 9-kg weighted boot | 36° | 1.4 | |
Ascending and descending stairs | 40°-60° | 3.3 | |
Deep squat | 135° | 7.6 | |
Scott and Winter | Running | Midstance | 7.0-11.1 |
* Because of the change in patellofemoral contact area at different points in the range of motion, it is appropriate to compare activities that occur only in similar ranges
Activities with high contact pressure
Loaded open chain knee extension from 50° to 20°
Closed chain activities with the knee in greater than 50° of flexion
Activities with low contact pressure
Loaded open chain knee extension from 90° to 50° and from 20° to 0°
Closed chain activities with the knee in less than 50° of flexion
The frontal plane forces that must be balanced by the extensor mechanism also originate from forces developed by the quadriceps. As with the sagittal plane forces, contraction of the vastus lateralis (VL), vastus intermedius, rectus femoris, and vastus medialis longus (VML) produces a superiorly directed force that is resisted by an inferiorly directed force from the patellar tendon. In the frontal plane these two opposing forces do not form a straight line but instead form an angle similar to the physiologic valgus angulation between the femur and tibia ( Fig. 19-4 ). Resolving these two forces provides a resultant force that is directed laterally. This resultant force is referred to as a valgus vector. Thus, when the quadriceps contracts, the patella has a tendency to shift laterally. This tendency toward lateralization is dynamically balanced by the vastus medialis obliquus (VMO) with assistance from the static restraints of the medial portion of the extensor retinaculum. When the patella is seated in the femoral sulcus, the lateral wall of the sulcus will also assist in resisting the laterally directed resultant force vector.

Several factors have been suggested to influence the magnitude of this valgus vector, including hip position, extensibility of the lateral retinacular structures, competence of the medial retinacular structures, femoral and tibial alignment, foot alignment, and ineffective firing or weakness of the VMO. Excessive hip internal rotation during the loading response in walking or running causes a functional increase in physiologic valgus of the femur and tibia. This leads to a greater valgus vector, which can decrease the efficiency of the extensor mechanism. Several factors can contribute to excessive hip internal rotation during gait, including weakness of the gluteus medius, tightness of the tensor fasciae latae, weakness of the hip lateral rotators, and excessive foot pronation. Tightness in the lateral retinaculum is associated with lateral compression syndrome in the patellofemoral joint. Loss of static restraint from the medial retinacular structures can also result in an increased tendency of the patella to track laterally.
Lower extremity bony malalignment can contribute to an increased valgus vector. Such malalignment includes genu valgum, anteversion of the femoral neck, and external tibial torsion. Hughston et al referred to combined femoral neck anteversion and external tibial torsion as the “treacherous extensor mechanism malalignment.” Varus deformity of the foot leading to excessive pronation during gait is another lower extremity alignment problem that contributes to an increased valgus vector. Eng and Pierrynowski reported that use of a soft foot orthosis with medial posting was effective in reducing pain in patients with symptomatic patellofemoral pain syndrome and varus foot deformities.
Some evidence in the literature suggests that closed chain exercise may positively influence tracking of the patellofemoral joint. Ingersoll and Knight compared patellar tracking angles in a group of normal subjects trained in biofeedback who were in a program involving both open and closed chain activities with a group of normal individuals performing a program of entirely open chain progressive resistance exercises. The group performing the combination of open and closed chain exercises with biofeedback had improved tracking measures, whereas the open chain–only group actually had an increase in lateral glide. Doucette and Child investigated the patellar congruency angle with computed tomography during an open chain activity and a closed chain activity in patients with lateral compression syndrome. The patellar congruence angle was improved in the closed chain activity versus the open chain activity at knee angles of 0°, 10°, and 20°. Their results also suggested that patellar tracking during open chain activities improves with greater amounts of flexion. They concluded that open chain exercise appears to be more appropriate at angles greater than 30° of flexion, which corresponds to the safe ranges of patellofemoral joint contact stress for open chain activities.
Muscle function
The quadriceps femoris is the primary dynamic stabilizer of the knee and is responsible for knee extension. Functionally, it plays an important role in decelerating knee flexion and absorbing shock when one lands on the lower extremity. As would be predicted based on the influence of gravity, open chain knee extension exercises with a 12–repetition maximum (RM) load produce greater quadriceps activity than do squats or leg presses with a 12-RM load as the knee extends from approximately 45° of flexion to 0°. Squats and leg presses produce more quadriceps activity from about 60° to 95° of knee flexion than does a 12-RM open chain knee extension load in the same ROM.
The straight leg raise, isometric quadriceps contraction (quad set), and knee extension exercises are typical therapeutic exercises prescribed after knee injury or surgery. Total quadriceps activity is greater during a quad set than during a straight leg raise or unweighted knee extension exercise. Soderberg and Cook reported electromyographic (EMG) data for the rectus femoris and vastus medialis during a straight leg raise and quad sets. They noted an increase in rectus femoris activity with a straight leg raise and an increase in vastus medialis activity when a quad set was performed. These results were supported by a study of EMG activity from the VMO, VML, vastus lateralis, and rectus femoris by Karst and Jewett. These authors also tested whether a straight leg raise performed with the hip in external rotation or while resisting an abduction force would preferentially recruit the VMO. They found no difference in VMO recruitment under these conditions. Karst and Jewett concluded was that if the goal was to address the vasti group, the quad set was a better exercise than the straight leg raise.
Currently, there is no way to test the strength of the VMO in isolation from the rest of the quadriceps musculature, so it is impossible to directly measure isolated weakness in the VMO. Consequently, researchers have used electromyography to quantify the firing patterns and behavior of the heads of the quadriceps. In particular, attention has centered around the firing patterns of the VMO and VL and VMO-to-VL ratios. The results of these studies have not been conclusive. It has been reported that in persons without patellofemoral pain syndrome, the VMO fires significantly faster than the VL does, whereas in persons with patellofemoral pain, the VL fires first. These results, however, were not supported by a more recent study. The VMO-to-VL ratio in both symptomatic and asymptomatic individuals engaged in a variety of open and closed chain activities has been reported to be approximately 1:1 in two studies and 2:1 in another. The discrepancy between these results is in part due to the large individual variability in the VMO-to-VL ratio even within the normal asymptomatic population. In normal individuals, Worrell et al reported a range of VMO-to-VL ratios of 0.35 to 17.21. Additionally, the reliability of the measurement is also problematic, with reported intraclass correlation coefficients being as low as 0.40.
Probably the most comprehensive investigation of quadriceps activity during different rehabilitation exercises was performed by Cerny. In this study, EMG activity was measured from the VMO, VL, and adductor magnus during more than 20 exercises in both normal individuals and patients with patellofemoral pain. The exercises with the greatest quadriceps EMG activity in this study appeared to be quad sets and step-downs. Cerny concluded that none of the exercises studied selectively recruited the VMO in either normal individuals or patients with patellofemoral pain syndrome. The addition of resisted hip adduction to squats or leg presses does not appear to have an impact on recruitment of the VMO or improve the outcome of patients with patellofemoral pain. In all the studies discussed, it should be noted that the VMO never functioned independently of any other head of the quadriceps during any of the exercises evaluated. Thus, the clinical results from these exercises would, in all likelihood, be a generalized quadriceps-strengthening effect.
Much debate has arisen about the different roles of the quadriceps musculature, especially the VMO, in the various ranges of motion. Historically, it was accepted that the VMO was responsible for terminal knee extension. Some of this interpretation was based on the fact that atrophy of the vastus medialis is more visible because of the normal prominence of the muscle and the thinness of its fascial covering in comparison to that over the VL. This visibility misled clinicians into the belief that the quadriceps atrophy was specific rather than general. At the same time, clinicians noted that patients had difficulty performing terminal knee extension and that an extensor lag was often present. In a classic series of studies Lieb and Perry defined the role of the VMO as a dynamic stabilizer against lateral displacement of the patella. Their studies determined that the VMO in isolation could not produce any extension of the knee. Each of the other parts of the quadriceps, in isolation, could produce knee extension. Interestingly, the first study was published in 1968, and confusion still lingers over the role of the VMO. Some of this confusion can be related to the subtlety of the function of the VMO in relation to the other quadriceps muscles. Box 19-3 summarizes the function of the VMO in the form of clinically relevant questions.
Is the VMO active during terminal knee extension? Yes, but so are the other parts of the quadriceps. In fact, the VMO is active through any range of knee extension when the other parts of the quadriceps are active.
Does the VMO become more active during open chain terminal knee extension than during open chain knee extension from 90 ° to 60 ° ? Yes, but so do the other parts of the quadriceps. It is estimated that the terminal ranges of knee extension require twice as much quadriceps force to accomplish the last 15° of extension because of lessening of the quadriceps mechanical advantage and an improvement in the mechanical advantage of gravity.
Is the VMO strengthened with terminal knee extension exercises? Yes, but so are the other parts of the quadriceps. It gets stronger through training, not because it is extending the knee, but because it is contracting against the patellar lateralizing force of the other parts of the quadriceps.
If the VMO is weak in isolation, can that weakness reduce knee extension torque? Yes, because without the function of the VMO to stabilize the patella, the extension torque created by the other parts of the quadriceps is not being applied through an efficient patellofemoral mechanism.
The hamstrings produce knee flexion, tibial rotation, and hip extension. Functionally, the hamstrings act more as hip extensors than as knee flexors because under most closed chain conditions, gravity produces the necessary knee flexion. For the knee joint, providing stability is a more important hamstring role than creating flexion. Hamstring contractions reduce loads on the ACL by creating a posterior shear force on the tibia. Cocontraction with the quadriceps also stiffens the knee joint and thus makes it more stable. The semitendinosus has been found to contract against valgus loads on the knee, whereas the biceps femoris contracts against varus loads. The biceps femoris rotates the tibia externally, and the semimembranosus and semitendinosus rotate the tibia internally. In the presence of anterolateral rotatory instability or anteromedial rotatory instability, facilitation of enhanced neuromuscular control of the biceps femoris (anterolateral rotatory instability) and the semimembranosus and semitendinosus (anteromedial rotatory instability) may assist in the control of abnormal tibial excursions.
An overview of knee rehabilitation principles
Before rehabilitation guidelines and protocols for specific pathologic knee conditions and surgical procedures can be addressed, an overview of general knee rehabilitation principles should be offered. Significant changes have recently occurred in the area of knee rehabilitation, with the greatest change being the speed at which patients are progressed through the rehabilitation process. Although the process has become much more rapid, the rehabilitation specialist should not simply focus on time frames to advance a patient’s rehabilitation program but should always keep in mind certain basic rehabilitation principles when evaluating the patient’s condition and the appropriateness of the rehabilitation program ( Box 19-4 ).
Awareness of the process of inflammation in the joint
Level of muscle control or strength
Amount of range of motion available
Establishment of weight-bearing status
Present functional status and desired outcomes
Note : The rehabilitation specialist should always be mindful of these principles when working within the time constraints placed on the rehabilitation program by any particular protocol.
After trauma to the knee, whether from surgery or injury, the acute inflammatory process must be addressed. Specifically, joint effusion should be evaluated and treated and pain management techniques should be initiated. Appropriate, early treatment focused on controlling acute inflammation can significantly affect the rehabilitation program, both immediately and at the final outcome. This early antiinflammatory treatment can help minimize losses in both strength and ROM. Ice, compression, and elevation, applied in conjunction with safe, pain-free condition-appropriate exercise, can aid in progressing through the acute stage of inflammation as rapidly as possible. Care should be taken by the rehabilitation specialist to not further irritate an acutely inflamed joint. Being too aggressive with exercises, weight-bearing status, or functional activities can keep the knee acutely inflamed and significantly lengthen the time needed for rehabilitation or even lead to permanent damage within the joint.
At the knee, achieving adequate quadriceps recruitment early in the rehabilitation process is extremely important. Quadriceps recruitment can be inhibited by the effects of acute inflammation. Kennedy et al determined that as little as 60 mL of saline injected into the knee decreases quadriceps recruitment by 30% to 50%. In a patient with true acute inflammation, the presence of pain could further diminish the ability to recruit the quadriceps. Biofeedback units may be used to aid the patient in developing a strong voluntary quadriceps contraction. If any voluntary contraction is difficult to perform, electrical muscle stimulation can be used to aid the patient in developing a contraction. Because Spencer et al found that as little as 20 mL of knee joint effusion may selectively inhibit contraction of the vastus medialis, this head of the quadriceps should be targeted when biofeedback or electrical stimulation is used.
Early knee motion after surgery or injury is critical to help prevent joint fibrosis, provide nutrition to the articular cartilage, and initiate controlled stress. This stress will help align the collagen fibers and thereby provide a flexible, strong scar and promote the return of normal joint mechanics. Active ROM at the knee, within case-appropriate range limitations, can be initiated as soon as pain allows. Supine heel slides can be used to aid in regaining knee flexion. However, such slides can be painful at the knee because of contraction of the rectus femoris during flexion at the hip. Active knee flexion can also be done in either the seated or side-lying positions. Active assisted ROM can be very effective in regaining ROM. Techniques such as using a towel to pull the knee into more flexion or having the clinician aid the athlete in taking the knee into more flexion often allow greater gains in ROM to be achieved, as long as the patient’s complaints of pain are not increased significantly. Slow pedaling on a stationary bike is another excellent way to increase knee ROM, as long as care is taken to not irritate the tibiofemoral or patellofemoral joints. If no substitution is allowed, the knee requires approximately 105° to 110° of flexion to complete a revolution on a properly fitted bike.
Mobilization of the patellofemoral joint should be initiated during the early-motion phase to help restore its normal arthrokinematics. Without normal patellofemoral arthrokinematics, it is very difficult for the patient to regain normal, pain-free knee flexion and extension. The patella should be mobilized superiorly, inferiorly, medially, and laterally. A decrease in superior and inferior patellar glide can decrease knee extension and flexion ROM, respectively. Stretching of the lateral patellar retinaculum, as described by McConnell, can possibly help decrease the incidence of patellofemoral pain by aiding in restoration of normal patellofemoral arthrokinematics.
As progress out of the acute stage of knee inflammation is made, more aggressive rehabilitation techniques can be initiated. However, it is imperative that the rehabilitation specialist accurately determine the status of the joint. Signs such as increased temperature, effusion, and levels of pain should be used to classify the stage of inflammation. For example, when the clinician feels soft tissue resistance to passive ROM before the athlete complains of any joint pain, more aggressive passive ROM techniques may be used ( Table 19-5 ). Knee extension is often the most difficult ROM to regain, but normal extension ROM is required before a normal gait pattern can be achieved. Regaining extension, both passive and, when appropriate, active, should be set as a very high priority in any knee rehabilitation program. Various techniques can be used. One method involves placing the athlete prone with the thigh resting on edge of the table, just proximal to the patella. As the athlete relaxes, the knee is pulled into extension. Weight can be added to the ankle to increase the force of the stretch. This method provides a low-load, long-duration stretch that can lead to plastic deformation of the tissues limiting extension ( Fig. 19-5 ). Spring-loaded splints are available commercially, such as the Dynasplint (see Fig. 6-8 ), *
* Available from Dynasplint Systems, Severna Park, MD.
that can be used to deliver a low-load, long-duration stretch to tissues that surround the patient’s knee.Reaction to Movement * | Joint Status | Treatment Action |
---|---|---|
Pain before resistance | Acute | Red light—No attempt should be made to gain ROM. |
Pain with resistance | Subacute | Yellow light—A gentle attempt can be made to regain ROM but should be done cautiously. (Vigorous attempts may cause reversion to the acute state.) |
Resistance before pain | Chronic | Green light—Vigorous intervention may be necessary to restore ROM. |

Numerous techniques can be used in an attempt to regain flexion at the athlete’s knee. For example, supine wall slides can be performed to regain early amounts of flexion. However, when the athlete achieves approximately 110° of flexion, wall slides may no longer be effective unless an additional outside force is applied, such as the athlete’s other leg ( Fig. 19-6 ). The final ranges of flexion can also be achieved by the athlete doing towel pulls while in a seated position. In addition, passive flexion can be achieved by the athlete using body weight while sliding forward from a seated position ( Fig. 19-7 ). Another method to increase flexion is by using a Total Gym †
† Available from Engineering Fitness International, San Diego, CA.
setup as pictured in Figure 19-8 . The stress that is applied to the knee is controlled by the angle of the slide of the board or by the range-limiting protection strap (or by both). Joint mobilization is an important adjunctive treatment that can be used to aid in regaining flexion. Both inferior glides of the patella and posterior glides of the tibiofemoral joint can be used to aid in increasing the athlete’s flexion. As gains in knee flexion are made, it is important to stretch the rectus femoris muscle. In addition to assisting in maintenance of the flexion gained, rectus femoris flexibility also reduces some of the patellofemoral joint compression force that occurs during flexion ROM activities.


Strengthening exercises should be initiated in a controlled fashion to ensure that the athlete properly executes the various strengthening exercises. These exercises should be progressed in a manner that both provides protection to healing structures and prevents abnormal muscle recruitment patterns or habits from developing. For example, additional resistance should not be added to straight leg raises or terminal knee extension exercises until the athlete can go through the full, case-appropriate ROM. If additional resistance is added too soon, the athlete might not be able to exercise throughout the full ROM and weakness in certain areas of the range might develop. For example, if too much resistance is added to an athlete’s terminal knee extension exercises and full extension ROM is not achieved during the exercise, an extensor lag could develop. This extensor lag could then be manifested in a patient’s functional activities as a deviation during gait.
Initially, simple exercises such as four-quadrant straight leg raises, multiple-angle isometrics, and both supine and prone terminal knee extension exercises can be started. These initial exercises are open chain exercises that allow isolation of muscles. These simple, one-plane exercises allow the rehabilitation specialist to concentrate on single muscle groups or physiologic movements that can be considered components of larger functional movements or skills. For example, four-quadrant straight leg raises can be used to strengthen the thigh and hip musculature in preparation for the athlete beginning more advanced functional activities such as single-leg squats.
Verbal, physical, and visual cues should be used to assist the athlete in maintaining correct lower extremity alignment during weight-bearing activities; examples include the following:
- •
Verbal cues remind the athlete to keep the knee directly over the foot during closed chain activities.
- •
Physical cues, such as rubber tubing (or Thera-Band), can be used to apply a valgus-directed load on the knee. The athlete is then instructed to resist the pull of the tubing (while keeping the knee over the foot) as squats, leg press, and other exercises are performed.
- •
Visual cues, such as performing the exercises in front of a full-length mirror, allow the athlete to see when lower extremity alignment is inappropriate.
When weight bearing is allowed, the athlete can begin performing appropriate closed chain exercises. This type of exercise uses muscles that cross joints both proximally and distally to assist with motion at the desired joint. These exercises allow athletes to strengthen muscles throughout their ROM but do not provide isolated strengthening of a particular muscle, which is why both open chain and closed chain exercises should be part of the rehabilitation program. Close attention must be paid to proper performance of these exercises. During closed chain exercises, each joint depends on other joints both proximally and distally to assist in proper body alignment. If closed chain exercises are begun too early or an athlete’s rehabilitation program is limited to just these types of exercise, weak muscles can be substituted for and abnormal habit patterns might develop. For example, if the hip musculature is not strong enough to control adduction and internal rotation of the thigh, the knee will assume a valgus alignment and the foot will be pronated. This alignment increases the Q angle at the knee and predisposes the athlete to the development of patellofemoral pain during the rehabilitation program. This can occur with a lateral step-up or leg press exercise ( Figs. 19-9 and 19-10 ). If this pattern of movement then becomes ingrained into the athlete’s movements during sporting activity, the athlete can become more susceptible to both overuse and traumatic injuries.


As strength and ROM levels in the lower extremity improve, proprioception exercises can be added to the rehabilitation program. Proprioception, as defined by Sherrington, refers to all neural input from joints, muscles, tendons, and associated deep tissues. Proprioception, also referred to as joint position sense, seems to primarily be determined by muscle spindle receptors. These muscle receptors are assisted to a lesser degree by cutaneous and joint receptors, thereby providing the neuromuscular control required for a joint to perform efficiently. Proprioception can initially be addressed in the rehabilitation program even before the patient progresses to full weight bearing. Patients can perform partial weight-bearing activities on the Biomechanical Ankle Platform System (BAPS) *
* Available from Camp International, Jackson, MI.
or Total Gym to begin stimulating the proprioceptive system. As the patient progresses to full weight-bearing activities, the proprioception exercises can be advanced in difficulty in a variety of ways. A sequenced minisquat program ( Box 19-5 ) is often a good method to begin this progression. When the minisquat program is initiated, the athlete has a tendency to excessively flex the trunk and pelvis to achieve the desired depth of motion ( Fig. 19-11 ). This substitution pattern reduces knee ROM and the load on the quadriceps. To train appropriate squat technique, especially as the depth of the squat sequence increases, it is often helpful to have the athlete hold a medicine ball or dumbbell out in front of the body ( Fig. 19-12 ). This provides a slight forward shift in the center of gravity, which in turn allows the athlete to learn how to “sit back” when performing a squat-type activity. To assist in the transition to single-leg full weight-bearing activities, a sled-style leg press or Total Gym is useful. For example, the athlete can hop from spot to spot while on the leg press machine ( Fig. 19-13 ). Progression of this exercise is accomplished by increasing load, increasing performance time, and changing the hop sequence.
Weight shifts with support
↓
Weight shifts without support
↓
Bilateral minisquats with support
↓
Bilateral minisquats without support
↓
Bilateral minisquats against a wall
↓
Bilateral minisquats against a wall with weights in hands
↓
One-legged minisquats with support
↓
One-legged minisquats without support
↓
One-legged minisquats against a wall
↓
One-legged minisquats against a wall with weights in hands
↓
Bilateral minisquats with tubing
↓
One-legged minisquats with tubing
↓
Gradual increase in tubing strength and speed of movement



As the athlete successfully progresses through a minisquat program, further challenges can be achieved with a lunge program ( Box 19-6 and Figs. 19-14 and 19-15 ) and finally a hop/plyometric program ( Box 19-7 and Fig. 19-16 ). Single-leg balance activities can also be performed with a Medi-Ball Rebounder *
† Available from The Hygenic Corporation, Akron, OH.
( Fig. 19-17 ). This type of activity can be progressed by changing from a stable weight-bearing surface to an unstable one, such as a wobble board. If a rebounder is not available, Thera-Band † can be used to increase the difficulty of proprioception exercises. The athlete stands on the involved leg with the Thera-Band attached to the uninvolved leg ( Fig. 19-18 ). Various activities can then be performed with the Thera-Band, such as flutter kicks, hip abduction/adduction, or hip flexion with knee flexion, and again the weight-bearing surface can be changed from stable to unstable as the exercises progress.
Balance and reach forward
Balance and reach backward
Balance and reach laterally
Forward lunge
Backward lunge
Lateral lunge
Diagonal lunge
Crossover lunge
Crossover diagonal lunge
* Lunges can be further progressed by having the athlete carry dumbbells, toss medicine balls, or work against rubber tubing resistance.


Double Leg
Hop in place
Forward hop
Backward hop
Triple hop
Side to side
Crossover
Scissors hop
Dot drills
180° hops
Single Leg
Forward hop
Triple hop
Backward hop
Side to side
Crossover
Dot drills
Lateral bounds
* The athlete should land as quietly as possible with good knee flexion to dissipate force. Difficulty is increased by increasing speed, distance, and time and by adding cones to jump over or adding external loads (or both). Lateral bounds are performed by taking off and landing on different lower extremities.



Other proprioception activities include the use of devices such as the BAPS and the slide board ( Fig. 19-19 ). Chapter 24 contains examples of lower extremity proprioceptive exercises. Care should be taken when one adds proprioception exercises to make sure that the athlete has the strength and coordination needed to safely perform the exercises. All the proprioception activities described force the involved leg to make numerous high-speed adjustments for the athlete to maintain balance.

As ROM, strength, and proprioception improve, cardiovascular and muscular endurance must be addressed in preparation for the athlete to return to full functional levels. Biking can be used to increase endurance when knee ROM is adequate. Pool exercises, including swimming, use of a kick board, or water running, can be used to increase endurance (see Chapter 11 ). Elliptical trainers are a good choice for a weight-bearing endurance activity. Because the elliptical motion is somewhat similar to running, but without the impact loading of running, it can be used as a precursor to a running program. Running can be used as an endurance activity, but care must be taken to not irritate the knee by advancing the program too aggressively. Table 19-6 contains an example of a running progression program. Threlkeld et al determined that backward running avoids the rapid initial loading of the knee that occurs with forward running because of the absence of heel strike. This may benefit an athlete who is unable to perform forward running as a result of pain.
Initial Activities | Advanced Activities |
---|---|
Jogging in place | Jogging in figure of eights (large to small) |
Jumping rope | Jog-sprint-jog (changing speeds) |
Jogging | Sprinting/reversing/cutting on specified spot |
Jogging forward/reversing on command | Sprinting/reversing/cutting on command |
Side-to-side sliding | Sport-specific drills |
Isokinetic equipment may be useful for the development of muscular power and endurance near the end of the rehabilitation process. Because anterior knee pain is often a complication of knee rehabilitation programs, caution must be used with these types of open chain exercises because of both the potential for high patellofemoral joint compression force and the possibility of excessive tibial translatory effects. For these reasons, one may have to limit the ROM that the athlete exercises through on this equipment.
The final stage of any knee rehabilitation program should be return to normal activities through the implementation of an appropriate progression of functional exercises. No athlete should be released back to sports simply on the basis of scores from various strength and ROM measurements. Functional activities should begin with exercises as simple as jogging in a straight line and progress from there. Both the speed and the difficulty of the exercises should be increased until athletes are able to perform activities similar to those that they will be expected to perform when they return to their sport. Specific functional progressions are discussed later in the chapter. Functional testing is covered later in the chapter as well.
In rehabilitation of the knee, every possible option should be offered to help the athlete return to the preinjury level. Each athlete should be constantly reevaluated throughout rehabilitation so that the program can be adjusted appropriately to address specific conditions and requirements. No two injuries are identical because every athlete’s physical, mental, and healing capabilities are different. Consequently, there are no specific “cookbook” approaches, and each rehabilitation program should be designed to maximize the athlete’s potential as quickly and as safely as possible.
Rehabilitation for specific knee injuries
Patellofemoral Dysfunction
Patellofemoral joint dysfunction with pain is one of the most prevalent pathologic knee conditions seen in athletes. This problem can be either the primary diagnosis or a secondary complication found with other knee injuries or after surgical procedures. Even if the patellofemoral joint is not the primary site of injury or dysfunction, care must be taken to not irritate or damage the joint during other lower extremity rehabilitation programs.
Many conditions fall under the broad heading of patellofemoral dysfunction. Anterior knee pain is often the phrase used to describe any condition that leads to pain in the extensor mechanism at the knee. Because the diagnosis is frequently one of a very general nature, rehabilitation can be viewed as general in nature as well. As with other overuse injuries, rehabilitation is often done to correct or improve the biomechanics of the entire lower extremity in an attempt to decrease the patient’s pain and dysfunction.
The need for a comprehensive lower extremity evaluation is paramount in athletes complaining of anterior knee pain. Functioning of the patella depends on a fine balance between ligaments and muscles because of the lack of inherent bony stability at the patellofemoral joint. When this balance is disrupted by weakness, tightness, or other biomechanical problems, improper tracking of the patella can occur. Lateral tracking of the patella is the most common tracking abnormality seen at the patellofemoral joint. Common causes of lateral tracking include VMO dysfunction, tight lateral soft tissues, and various biomechanical problems that increase the tendency of the patella to track laterally. VMO dysfunction can be caused by weakness of the VMO from disuse or effusion-induced neuromuscular shutdown. If the VMO is not pulling with enough force, it cannot offer the medially directed dynamic stability that is required to counteract the other heads of the quadriceps and keep the patella tracking appropriately. Tight lateral soft tissues around the knee can also increase lateral tracking of the patella. If structures such as the patellar retinaculum, iliotibial band, and tensor fasciae latae are tight, they can cause the patella to track more laterally and possibly lead to pain in the area. Biomechanical problems anywhere along the lower extremity that affect alignment of the femur on the tibia can affect tracking of the patella. Many of these malalignment problems can be bony in nature, and it is not possible for the rehabilitation specialist to change them. For example, a wide pelvis that leads to an increased genu valgum angle at the knee cannot be “corrected” by rehabilitation. However, some malalignment problems are able to be treated. For example, excessive pronation of the subtalar joint can lead to an increased Q angle at the knee and possibly increased lateral tracking at the patellofemoral joint. The rehabilitation specialist can treat this excessive pronation as described later in this chapter.
Rehabilitation of patellofemoral dysfunction, after a comprehensive evaluation, should concentrate on recruiting the VMO, normalizing patellar mobility, increasing general flexibility and muscular control of the entire lower extremity, and addressing any other biomechanical problems that can be altered by treatment ( Box 19-8 ). The most important concept to keep in mind when the patellofemoral joint is being rehabilitated is that no exercise should cause pain at the joint. Both the patient and the rehabilitation specialist should always proceed with this guiding factor in mind.
After a comprehensive lower extremity evaluation:
- •
Facilitate recruitment of the VMO
- •
Normalize patellar mobility
- •
Increase lower extremity flexibility
- •
Address any alterable lower extremity biomechanical problems
- •
Be sure that all activities are pain free at the patellofemoral joint
VMO, Vastus medialis obliquus.
Although various exercises have been touted to isolate the VMO, no specific exercises have been proved to isolate the VMO. Therefore, when the rehabilitation specialist is attempting to recruit and strengthen the VMO, no specific exercise should be considered superior to all others. Any knee extension exercise that elicits contraction of the VMO and does not cause pain at the patellofemoral joint is appropriate. Use of a biofeedback unit to monitor VMO contraction is one method that is extremely helpful in ensuring that exercises are having an impact on the VMO. A biofeedback unit can be used in conjunction with a variety of open and closed chain exercises. Electrical stimulation units may also be used to obtain a strong contraction in the VMO.
Lower extremity biomechanical problems frequently lead to or potentiate patellofemoral dysfunction. Key areas to evaluate and address during rehabilitation include hip abductor strength, foot alignment, and the flexibility of the rectus femoris, hamstrings, gastrocsoleus, and tensor fascia latae.
Several techniques have been reported to be helpful in normalizing patellar mobility in patients who have a laterally tracking patella because of tight lateral structures. The three most commonly reported techniques are manual patellar mobilization, patellar taping as described by McConnell, and iliotibial band/tensor fasciae latae stretching. Kramer reported success with manual lateral retinaculum stretching when it was used as part of a comprehensive patellofemoral rehabilitation program. He described two manual maneuvers: (1) medial patellar glide held for 1 minute with the knee extended to stretch the lateral retinaculum and (2) patellar compression with tracking. This second technique is performed with the athlete sitting and the knee flexed to 90°. The patella is compressed against the patellofemoral articular surface and tracked medially by the clinician as the athlete extends the knee ( Fig. 19-20 ). Neither of these techniques should cause any pain in the athlete’s patellofemoral joint. Another method of stretching the lateral structures involves flexing the knee to approximately 30° to 60° of flexion and then applying a posterolateral force against the medial border of the patella. This pushes the medial border of the patella down and lifts the lateral border, thereby stretching the lateral structures.

Patellar taping as described by McConnell remains somewhat controversial in patellofemoral rehabilitation, in large part because the mechanism of how it works remains to be elucidated. The results of studies examining the effects of taping on quadriceps activity, joint kinematics, and patellar positioning have been inconsistent and are generally of low study quality. Derasari et al also suggested that changes in these variables in the posttaped condition are very much influenced by their baseline. However, the majority of studies using pain as an outcome measure have demonstrated a reduction in pain. Therefore, if taping techniques allow the athlete to exercise with reduced or no complaints of anterior knee pain, the techniques should be used. However, the athlete should not rely on taping techniques for prophylaxis; an appropriate rehabilitation program addressing the underlying causes of the pain should be followed.
McConnell based the application of tape on various evaluative measurements. She looked at several components of patellar orientation: (1) the medial/lateral glide component, (2) the medial/lateral tilt component, (3) the rotation component, and (4) the anterior/posterior tilt component. It has been reported that these measurements are not able to be obtained reliably, either between testers or even by the same tester at different times. However, there are other methods of determining which taping techniques should be used. It is rather simple to quickly ascertain whether the taping techniques will benefit a particular patient. One activity that reliably replicates a patient’s complaints of patellofemoral pain must be found before the taping techniques can be used. This activity is referred to as the asterisk sign . Usually, a lateral step-up or minisquat is found to be an asterisk sign for these patients. As individual pieces of tape are placed on the patient’s knee, the asterisk sign is reevaluated to determine whether the tape has decreased the patient’s pain. McConnell described four main types of tape application: (1) correcting lateral glide, (2) correcting lateral tilt, (3) correcting external rotation, and (4) correcting anterior-posterior tilt, in which the inferior pole of the patella is tilted posteriorly ( Fig. 19-21 ). Although whether these pieces of tape correct the position that McConnell described is still being questioned, the tape can still be of clinical use. If pain with the patient’s asterisk sign is decreased or alleviated after an individual piece of tape is applied, that piece of tape is left on. If the tape does not have an impact on pain with the asterisk sign, that piece of tape is removed. This simple procedure is used for all four of the taping techniques that are described. Although attempting to answer why these techniques might work is very difficult, one may quite simply determine whether the tape is a reasonable adjunct to the treatment regimen. The authors have anecdotally found taping techniques to be a reasonable addition that can help many patients progress through a patellofemoral rehabilitation program if they are used appropriately.

Finally, in the area of normalizing patellar mobility, specific attention should be paid to the iliotibial band and tensor fasciae latae. If during the evaluation these structures are found to be tight, stretching should be instituted. It is important that stretching of these structures be performed correctly ( Fig. 19-22 ). Ultrasound applied to these structures in conjunction with stretching may aid in gaining flexibility.

The next area that should be addressed in patellofemoral rehabilitation is increasing general flexibility and muscular control of the entire lower extremity. It is especially important that the athlete have normal levels of flexibility in the rectus femoris, iliopsoas, and hamstring muscles. A tight rectus femoris can cause increased patellofemoral joint pressure. Tight iliopsoas and hamstrings can lead to abnormal gait patterns that increase muscular activity at the knee and patellofemoral joint pressure. Strengthening of the hip abductors to better control the hip in the transverse plane is extremely important ( Fig. 19-23 ). It has been reported that excessive medial rotation of the femur during weight bearing has more impact on patients with patellofemoral pain than does lateral movement of the patella. The athlete should not perform any closed chain exercise in which the hip cannot be controlled ( Fig. 19-24 ; also see Figs. 19-9 and 19-10 ). As flexibility and strength of the lower extremity increase, the athlete must be able to show good muscle control and coordination for the entire lower extremity. This idea of appropriate control at various speeds of activity was discussed earlier in this chapter.


One final topic to be discussed is correctable biomechanical problems that affect lower extremity alignment. The most common problem in this area is excessive pronation at the subtalar joint, which can lead to an increased Q angle at the patellofemoral joint. This excessive pronation is often a compensation for a forefoot varus malalignment. Arch taping techniques, such as the Low-Dye (see Fig. 20-37 ) or Herzog technique, can be used to determine whether controlling pronation will affect the patient’s patellofemoral pain. If taping does lessen the symptoms, orthotics can be used. In a patient with forefoot varus, the orthotic would be constructed with a medial forefoot post to control motion of the forefoot. Appropriate shoe selection and replacement of worn shoes can also aid in control of excessive pronation.
If conservative treatment fails, surgery may be indicated. One of the most common procedures is lateral retinacular release in which the lateral retinaculum is cut to free the patella medially. Additionally, advancement of the VMO can be done in patients who have a VMO with a line of pull more vertical than normal. This is a type of proximal realignment. Table 19-7 shows a lateral release rehabilitation protocol for patients who have not undergone some sort of proximal realignment involving the VMO. The goals in this postoperative rehabilitation program are very similar to those of the conservative rehabilitation program for patellofemoral dysfunction. Emphasis should be placed on regaining patellar mobility and VMO/quadriceps control. Although this surgical procedure was quite popular in the past, it is presently performed less often and only after a course of conservative therapy has failed.
Week 1 | Weeks 2-4 | Weeks 4-6 | Week 6 | |
---|---|---|---|---|
Functional progression | Begin WB and four-quadrant lifts | Begin WB without crutches | Begin strengthening Absence of pain | Return to sport No effusion |
Criteria | As pain allows | SLR with no extensor lag Full extension in gait No limp No increase in pain No increase in edema/effusion | No increase in edema/effusion Full ROM | Functional testing > 85% Quadriceps strength > 85% |
Evaluation | Pain Incision Effusion/edema Active flexion Quadriceps recruitment Passive extension Patellar mobility Hamstring flexibility | Pain Gait Incision/scar Effusion/edema ROM/patellar mobility Quadriceps recruitment | ROM Quadriceps recruitment Patellar mobility Standing balance Self-report functional status | Functional testing Strength testing Self-report functional status |
Treatment | Pain management Effusion/edema control Active flexion exercise Quadriceps recruitment exercises Passive extension Patellar mobilization Hamstring stretching IT band stretching | Pain management Effusion/edema control Scar massage Active ROM exercise Patellar mobilization Quadriceps recruitment General strengthening Flexibility exercise Minisquat progression | Strengthening Endurance exercise Proprioception exercise Hop and lunge progression Jogging progression | Strengthening Endurance exercise Sport-specific drills |
Goals | 75% WB Increase patellar mobility Full passive extension SLR without extensor lag | WB without crutches AROM 0°-110° Normal patellar mobility Good flexibility | Full AROM Strengthening without pain | Return to sport |
Other more involved surgical procedures, such as distal realignment, can be performed if conservative therapy fails. In a distal realignment procedure the tibial tubercle and patellar tendon are transferred to decrease the patient’s Q angle. The main concern with this procedure is advancing knee flexion ROM and weight-bearing status in a fashion that does not excessively stress the tissues involved in surgery. The speed of the rehabilitation program is controlled by the quality of fixation of the realigned bone. Overaggressive flexion ROM work or weight bearing can place too much stress on the fixation site and lead to complications.
Patellar Tendinopathy
Within patellofemoral pain/dysfunction one can consider patellar tendinopathy a special subset of this type of dysfunction. Patellar tendinopathy is a common source of anterior knee pain in athletes engaged in jumping sports, hence the common name “jumper’s knee.” Although the same principles discussed for patellofemoral dysfunction earlier apply to rehabilitation of patellar tendinopathy, it appears that the quadriceps-strengthening program should emphasize eccentric training. Several specialized eccentric training programs have been recommended, with performance of single-leg squats on a decline board being one of the most common. The typical protocol used with single-leg squats on a decline board includes 3 sets of 15 repetitions performed 2 times a day. Load is increased in 5-kg increments when the athlete’s pain during the exercise is less than 3 on a 0 to 10 visual analog pain scale. Load is reduced if the athlete’s pain is greater than 5 on the same scale. The athlete performs the eccentric (lowering) phase of the squat with the involved lower extremity and the concentric (raising) phase with the uninvolved lower extremity. The most commonly recommended decline is 25°, but declines anywhere from 15° to 30° produce similar loads on the patellar tendon, so any decline within this range can be chosen based on the athlete’s comfort and control of the exercise. Because of loads on the patellofemoral joint itself during this activity, the athlete should avoid knee flexion angles greater that 60° when performing this exercise.
Surgical treatment of patellar tendinopathy is an option, but the results of a randomized controlled study directly comparing surgical intervention with eccentric training demonstrated no difference in outcome between the two groups. If the athlete chooses surgical treatment, the emphasis of rehabilitation for the first 6 weeks after surgery is on normalizing ROM and flexibility, controlling inflammation, and gradually introducing pain-free strengthening exercises. After 6 weeks, if the athlete has met the criteria of full ROM, normal flexibility, normal gait, no effusion, and good quadriceps control, the emphasis can shift to an eccentric training program. This can be performed similar to the conservative eccentric treatment program described earlier, with the exception that the athlete should not experience pain during the exercise and any progression should be pain free.
Anterior Cruciate Ligament Injuries
Injuries involving the ACL continue to be relatively common in athletics. Although rehabilitation of this injury still varies, there is greater agreement now than there was historically, especially in the early phase of intervention. We will present an overview of commonly accepted guidelines. The time frames discussed in any protocols should be adapted to fit various situations, with progression through any protocol being defined by criteria-based guidelines instead of time alone.
Regardless of whether an athlete has decided to undergo a surgical procedure or conservative treatment, intervention immediately following an ACL injury is the same. The immediate goal is to control the hemarthrosis and general inflammatory process. The athlete should be given crutches and instructed in a pain-free partial weight-bearing gait, and the traditional antiinflammatory program of ice, compression, and elevation should be started. A brace is not required unless other associated ligamentous injuries are present. Motion exercises should be started immediately, with concentration on passive extension to help prevent rapid scarring in the intercondylar notch. Full extension also allows greater ease of quadriceps recruitment, which is extremely important after ACL injury. Friden et al stated that quadriceps strength decreases after injury because of defects in afferent inflow from the ACL-deficient knee. It is also thought that the lack of voluntary contraction of the quadriceps after ACL injury may be due to reflex inhibition or arthrogenous muscle inhibition. Weight bearing should be increased as pain decreases, joint effusion decreases, quadriceps control increases, and full active knee extension is achieved. The athlete should not be progressed to full weight bearing until these goals have been accomplished.
If the athlete has chosen conservative care, rehabilitation should progress with emphasis placed on quadriceps strengthening, as well as neuromuscular control of the hamstrings. Historically, emphasis was placed on strengthening of the hamstrings because of their role as the primary dynamic restraint in controlling anterior tibial translation. The importance of the quadriceps to outcome is now better understood, and accordingly, emphasis has changed to addressing general muscle control around the knee. A study by Friden et al in which 26 patients with ACL-deficient knees were tested before and after rehabilitation revealed that loss of strength in the hamstrings was minimal and that the best outcomes were obtained when both general muscular strength and coordination were addressed. The importance of the hamstrings in an ACL-deficient knee might lie more in hamstring control and proprioception. Studies indicate that athletes with complete tears of the ACL experience a decrease in proprioception at the knee. The loss of proprioception may be due to the “ACL-mechanoreceptor reflex arc” to the hamstrings. Beard et al found that the latency of reflex hamstring contraction in an ACL-deficient knee was twice that of the contralateral, uninjured knee. It has been suggested that improving recruitment time of the hamstrings may place less stress on the ACL during functional activities. In addition, it has been reported that using active hamstring control to reduce the pivot shift found with an ACL injury might be the key to successfully avoiding reconstructive surgery. Based on all this information, the rehabilitation specialist should concentrate on facilitating control of the hamstrings, as opposed to simple strengthening in the sagittal plane. Engle and Canner reported success in achieving this type of hamstring control in ACL-deficient knees with a program that uses proprioceptive neuromuscular facilitation (PNF) exercises for the hamstrings ( Figs. 19-25 and 19-26 ). More advanced exercises using devices such as the BAPS board, Slide Board, and Medi-Ball Rebounder facilitate hamstring control and proprioception in functional positions and at higher speeds to control anterior tibial translation during more aggressive functional activities. PNF patterns and seated Thera-Band exercises can also be used to help incorporate the tibial rotation component of the function of the hamstrings. Progressive perturbation training has likewise shown promise when added to standard program of strengthening, aerobic endurance training, agility, and sport-specific activities. Fitzgerald et al reported that ACL-deficient subjects in their progressive perturbation plus standard rehabilitation group were almost five times more likely to return to full sports participation than were ACL-deficient subjects in the standard rehabilitation group. Studies have shown that patellofemoral arthrokinematics is altered in ACL-deficient knees. This should be kept in mind during rehabilitation of an ACL-deficient knee, and care should be taken to not irritate or damage the patellofemoral joint.


Although surgery is the most commonly chosen treatment option for competitive athletes, surgery should be delayed until the acute inflammatory process has run its course at the knee. There is great concern that performing surgery on an acutely inflamed joint will lead to more complications during rehabilitation. When the period of acute inflammation has passed, the athlete, using information provided by the sports medicine team, must decide whether a reconstructive procedure is the most appropriate treatment to choose. Whether to reconstruct the ACL or treat it with just a rehabilitation program continues to be a subject of debate. Many factors should be considered when this decision is made, the most important being the ultimate level of function that the patient wishes to achieve. Noyes has recommended that to have the best results, competitive or recreational athletes need surgical intervention whereas light recreational athletes and nonathletes, both of whom can limit their activities, may be able to avoid surgery. Clinically, it appears that a competitive athlete does not generally perform well with an ACL-deficient knee. We are just beginning to understand the combinations of examination measures that may identify the few athletes who might function well without surgery. Noyes et al used subjective and objective measurements to clinically evaluate 84 individuals with ACL-deficient knees. Their conclusion was that an ACL tear leads to functional disability in the majority of patients. They found that one third of the population compensated for the deficiency, knew their limits, and did well; one third of the population compensated for the deficiency but found the instability aggravating; and one third became worse and needed surgery to correct their instability. For the majority of patients who did not undergo surgical reconstructions, knee “giving way” episodes became a common problem. These giving way episodes increase the possibility of a meniscal tear or chondral injury developing. It is well documented that ACL-deficient knees demonstrate abnormal joint kinematics during gait and functional activities, both of which increase the possibility for the development of early degenerative changes.
When surgery is the chosen treatment, the ACL must be reconstructed because of the lack of success with direct repair of the ligament. The one exception to this rule might be repair of a bony avulsion. If an adolescent has an avulsion fracture without significant ligament failure, primary repair can be a viable option. Concerns that must be addressed when surgery is the treatment of choice include timing of the procedure, graft selection, and surgical technique. The timing of surgery in relation to the inflammatory condition of the joint can greatly affect rehabilitation and ultimate outcomes. It has been shown that reconstructions performed on an acutely inflamed joint are more prone to postoperative complications such as loss of ROM and deficits in function. Allowing the patient to go through rehabilitation before surgery helps decrease the number and severity of postoperative complications.
Surgical technique is an area that includes both procedures that are chosen and how well these procedures are ultimately performed. Procedures relate mainly to intraarticular versus extraarticular reconstructions. Extraarticular reconstructions, such as the Ellison or Losee procedures, have been shown to reduce pivot shift but do not reliably limit anterior translation of the tibia or restore normal arthrokinematics through the axis of a normal ACL. Long-term control of knee instability with these extraarticular procedures is poor because of the gradual stretching that occurs in the soft tissues used in the surgical procedure, and therefore extraarticular procedures are used rarely. One remaining use for extraarticular procedures is in skeletally immature patients. Procedures that require drilling through an open physis are often avoided by surgeons because of the possibility of bone growth complications. Although reports are inconsistent, studies have shown that complications have occurred, including leg length discrepancy and physis arrest with the concomitant development of bone deformity. For these reasons it is suggested that skeletally immature patients, especially those who are prepubescent, be treated with physis-sparing surgical procedures if surgery is the treatment of choice.
Intraarticular procedures are by far the most commonly used for reconstruction of the ACL. Both graft fixation and graft placement are extremely important surgical variables that will affect the patient’s outcome. After years of refinement, the choice of fixation device is now less of a concern than the material that the surgeon is attempting to fixate. In other words, it is easier to obtain early stable fixation when the graft has a bony end as opposed to just tendon. Moreover, it is imperative that isometric graft placement be achieved at the time of surgery. If the graft placement sites are not appropriate, deficits in ROM or abnormal ligament tension might be the result.
A variety of different grafts have been examined for use in intraarticular procedures. Important factors to take into consideration when deciding on graft material are graft strength, fixation required, and comorbid conditions that might be present (including factors such as donor site complications and the possibility of disease transmission). Many types of tissue have been used for intraarticular repair of the ACL, including autografts (tissue transferred from one part of a person’s body to another), allografts (human donor tissue), and prosthetics (synthetic material). The most commonly used tissue for both autografts and allografts is a bone-tendon-bone (BTB) taken from the central third of the patellar tendon and the semitendinosus tendon from the hamstrings. Other material can be used as an allograft, including fascia lata and Achilles tendon. Each type of graft has advantages and disadvantages ( Table 19-8 ), which must be taken into consideration when planning the surgery. At one point a BTB autograft was considered the “gold standard” in ACL reconstruction, and although it remains one of the more commonly used grafts, metaanalyses have failed to find significant differences in outcome based on the type of graft used. This suggests that according to currently available evidence, factors other than the type of graft used play larger roles in affecting outcome.
Graft | Advantages | Disadvantages |
---|---|---|
Patellar BTB autograft | High graft strength Good fixation because of bone-to-bone healing Ease of obtaining graft material | Complications with the extensor mechanism, such as patellar fractures, patellar tendinitis, patellar tendon rupture, and anterior knee pain |
Hamstring tendon autograft | Fewer complications with the extensor mechanism Comparable results to BTB autograft | Complications with fixation of graft Possible hamstring weakness More technically complicated procedure |
Allograft | No complications with donor sites Increased availability of material | Higher cost Possible disease transmission Possible recipient rejection |
The times needed and the percentages of graft strength and revascularization achieved have engendered much debate. Noyes et al reported that at the time of harvesting, 14-mm-wide patellar tendon grafts exhibited 168% of the strength of a normal ACL. Cooper et al tested the strength of 10-mm–wide grafts. They chose 10-mm–wide grafts because they believed that most surgeons use this size of graft to minimize the possibility of impingement of the graft in the femoral intercondylar notch. They reported that at the time of harvest the 10-mm–wide graft exhibited 174% of the strength of a normal ACL. Others have reported 10-mm–wide BTB autograft strength to be less (107%) but still above normal values for the ACL. Unfortunately, because of the graft’s loss of vascular supply at the time of harvest, its strength decreases significantly from the time of implantation, and it never regains its initial strength levels. The avascular necrosis that occurs after implantation causes the graft to be its weakest somewhere in the 4- to 8-week postoperative period. On the basis of data from animal models, it is generally accepted that BTB autografts undergo a “ligamentization” process that results in a graft whose vascular and histologic appearance 1 year postoperatively resembles that of a normal ACL. Using the medial third of the patellar tendon in rhesus monkeys, Clancy et al reported transplanted patellar tendon graft strength to be 53% of normal ACL strength at 3 months, 52% of normal at 6 months, 81% of normal at 9 months, and 81% of normal at 12 months. Although these values are below normal strength levels and far below the strength values at the time of harvesting and implantation, Noyes et al suggested that in most strenuous activities, the ACL is seldom exposed to more than 50% of its maximal load. Allografts appear to go through the same process of avascular necrosis followed by revascularization and cellular proliferation. However, it is believed that patellar tendon allografts are generally weaker throughout the rehabilitation process, which accounts for the slight differences that are often seen in rehabilitation protocols.
Although numerous rehabilitation protocols have been developed, the majority of the most commonly used protocols have certain common themes. Most protocols recommend rehabilitation before surgery. The goals for this stage of rehabilitation include regaining full ROM and neuromuscular control, as well as minimizing the inflammatory process. The risk for arthrofibrosis and other postoperative complications is increased when surgery is performed on an acutely inflamed joint. Eitzen et al recommend a 5-week rehabilitation program before surgery that in addition to ROM, includes strengthening, aerobic exercise, plyometric exercises, and perturbation training.
Early Postsurgical Phase
Immediate postsurgical protocols now emphasize early motion, control of inflammation, quadriceps facilitation activities, and patellar mobility. Depending on the surgeon’s preference, controlled weight bearing and initiation of low-load closed chain exercises can also begin in this stage. Even though the exact time frames vary from protocol to protocol, it has been found that achieving the early goals of full passive extension, good quadriceps control, and minimal joint effusion/inflammation leads to better ultimate outcomes for the patient ( Box 19-9 ). In this initial period of rehabilitation, emphasis should be placed on gaining and maintaining full extension because flexion ROM will usually increase as pain and effusion decrease. Full extension is critical for gaining early quadriceps control. In this immediate postoperative phase, quadriceps facilitation activities often include quad sets, multiple-angle knee extension isometrics, neuromuscular electrical stimulation, and weight bearing in extension (if weight bearing is allowed). Because of stress on the ACL graft, protocols often avoid multiple-angle knee isometrics in the 45° to 5° range. Straight leg raises can also be used in this stage as long as the athlete can perform the exercise without a quadriceps lag. If the surgeon is confident about graft fixation, active terminal knee extension exercises can be initiated as soon as the postoperative pain has decreased. These exercises should be done with no more resistance than simply the weight of the leg. Traditionally, any loaded open chain knee extension exercise in the 45° to 0° range has been avoided because of the stress placed on the graft. However, because the graft is strong when it is initially placed in the knee and loses strength only as it necroses and goes through its revascularization process, active terminal knee extension exercises with no added resistance can be used early in rehabilitation to aid in facilitating quadriceps recruitment. If the athlete has difficulty performing supine terminal knee extension exercises, they can be performed in the prone position, where the hip extensors can aid in achieving full knee extension ( Fig. 19-27 ).
Decrease knee joint effusion
Achieve full passive knee extension
Develop good quadriceps control, especially in extension
Develop/maintain patellar mobility

When a patellar tendon autograft is used as the graft material, it is important to achieve normal patellar mobility so that scarring around the harvest site does not occur and lead to infrapatellar contracture syndrome. If the patella loses mobility as a result of scarring, ROM and strength can be significantly decreased. Early in the rehabilitation process, the patellar area might be too tender because of the incision at the graft harvest site to allow productive mobilization. Facilitation of early quadriceps recruitment will help in obtaining patellar movement. Electrical stimulation can be extremely valuable in facilitating a quadriceps contraction great enough to result in superior mobilization of the patella. If electrical stimulation is being used in this early stage for quadriceps strengthening instead of merely facilitation, the knee should be placed in approximately 60° or greater of flexion. Developing quadriceps control at full knee extension is extremely important for several reasons. Quadriceps contractions increase patellar mobility, these quadriceps contractions help maintain full extension ROM, and without good quadriceps control in full extension the athlete will not be able to ambulate with a normal gait pattern. When passive knee extension ROM is obtained during rehabilitation, it can be very difficult to maintain unless the patient has enough quadriceps strength to actively go into full extension.
When the patient can actively recruit the quadriceps, using biofeedback units to increase the level of recruitment can be extremely helpful. Biofeedback requires complete patient involvement because it monitors only what the patient is doing and offers no stimulation of the muscle. For patients who tolerate electrical stimulation, Snyder-Mackler et al found that it helped them regain quadriceps strength at a faster rate. In a study of 110 patients, they found that high-intensity electrical stimulation performed at 65° of flexion in a closed chain position resulted in 70% recovery of quadriceps strength by 6 weeks postoperatively. This compared favorably with the 57% recovery of quadriceps strength in a group that performed only closed chain strengthening exercises. In addition, the group that received electrical stimulation showed better knee control at midstance than did the group that exercised without electrical stimulation.
Weight-bearing status varies between protocols, but most commonly at least partial weight bearing is initiated within days of surgery. It is extremely important to have patients walk with as normal a gait as possible while they are on crutches. Poor habits during gait can be developed in this early stage of rehabilitation, and once a poor gait pattern becomes ingrained, it can be very difficult to break the habit. The most common gait deviation is ambulation with the knee in flexion and never going into full extension. This lack of full extension in gait may be due to lack of strength or ROM (or both), a habit pattern developed before surgery as a result of instability at the knee or a habit pattern developed after surgery. Some protocols require the patient to wear a brace at 0° immediately after surgery so that ambulating with the knee in flexion is not as common a complication. Low-load closed chain exercises can be safely performed in this early stage without creating stresses that are harmful to the graft. The decreased stress that is afforded by the use of closed chain exercises is due to the compression forces at the tibiofemoral joint and the cocontraction of other muscles to help control motion at the hip, knee, and ankle. An excellent initial closed chain exercise is terminal knee extension in the standing position with the use of a Thera-Band ( Fig. 19-28 ).

Progressive Strengthening and Balance Training Stage
The next stage of rehabilitation shifts the focus of the program to progressive strengthening and balance activities. The athlete should advance into this phase only after demonstrating full knee extension, at least 110° of flexion, minimal or no effusion, no quadriceps lag, no pain, and a normal gait pattern without assistive devices. Muscle soreness is not uncommon in this stage, especially as loads and depth of motion are increased, but any activities that cause joint pain should be avoided. Progression of activities through this stage should be curtailed if joint soreness or effusion occurs. If the athlete has also undergone meniscus repair, the rate of progression for lunges, squats, and leg presses (in particular, the depth of motion for these activities) is often slower. If the surgeon used a hamstring autograph for the reconstruction, the rate of progression of open chain hamstring strengthening is frequently slower because of symptoms from the donor site. When the athlete begins this phase, strengthening activities are usually performed with closed chain exercises. It is critical that as loads are increased in closed chain activities, the athlete maintains proper technique. Medial collapse of the lower extremity (see Figs. 19-9 and 19-10 ), “leaking” too far forward (see Figs. 19-11 and 19-24 ), and lack of symmetric loading ( Fig. 19-29 ) are common errors in technique that occur if the athlete cannot control the load. If progressive resistance open chain quadriceps strengthening is included before postoperative week 8 to 10, it is generally still blocked from full extension. The amount of extension that is blocked varies from 30° to 60°, depending on the protocol. Full-range, open chain quadriceps strengthening is generally allowed any time from 10 to 12 weeks after surgery, depending on the specific protocol being followed. It is important to use both closed and open chain quadriceps strengthening activities during this stage because the combination has been demonstrated to produce greater quadriceps strength and earlier return to sports participation than closed chain strengthening activities alone. Because the speed of protocols for rehabilitation after ACL reconstruction has increased so much in recent years, it is imperative that the exercises chosen produce a safe amount of stress on the revascularizing ACL. These exercises were presented earlier in this chapter in the section on functional anatomy of the ACL. An understanding of the exercises that place high loads on the ACL is necessary to determine safe exercises at different time frames in the rehabilitation program.

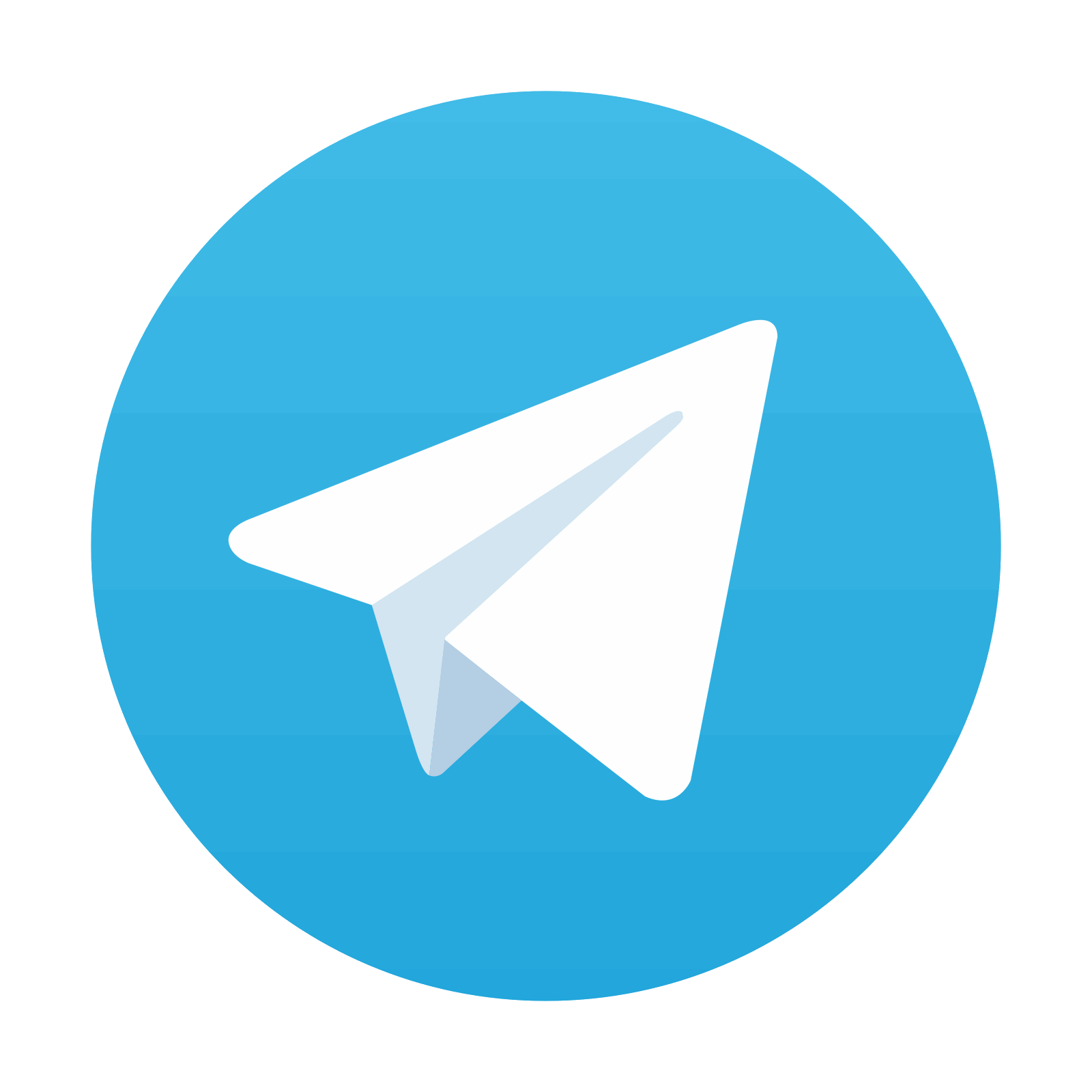
Stay updated, free articles. Join our Telegram channel

Full access? Get Clinical Tree
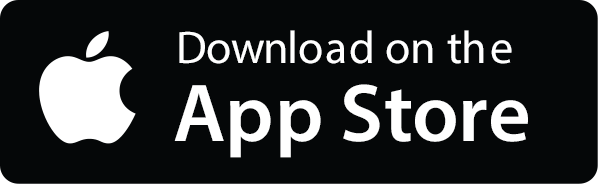
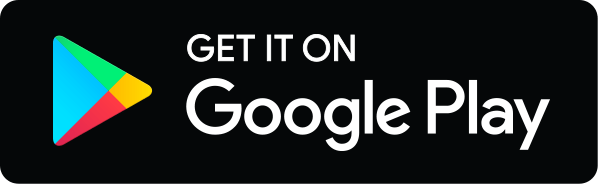
