Chapter 2 Joint Anatomy and Basic Biomechanics
This chapter provides an academic picture of the applied anatomy and clinical biomechanics of the musculoskeletal system. The human body may be viewed as a machine formed of many different parts that allow motion. These motions occur at the many joints formed by the specific parts that compose the body’s musculoskeletal system. Although there is some controversy and speculation among those who study these complex activities, the information presented here is considered essential for understanding clinical correlations and applications. Clinical biomechanics and applied anatomy encompass the body of knowledge that employs mechanical facts, concepts, principles, terms, methodologies, and mathematics to interpret and analyze normal and abnormal human anatomy and physiology. Discussions of these concepts require specific nomenclature, which enables people working in a wide variety of disciplines to communicate (see glossary). Biomechanics is often overwhelming because of its mathematical and engineering emphasis. This chapter presents a nonmathematical approach to defining clinically useful biomechanical concepts necessary to describe and interpret changes in joint function. Thorough explanations of biomechanical concepts are discussed in other works.1 2 3
Fundamental concepts, principles, and terms
Levers
In a first-class lever, the axis (fulcrum) is located between the force and resistance; in a second-class lever, the resistance is between the axis and the force; and in a third-class lever, the force is between the axis and the resistance (Figure 2-1). Every movable bone in the body acts alone or in combination, forcing a network of lever systems characteristic of first- and third-class levers. There are virtually no second-class levers in the body, although opening the mouth against resistance is an example.
Body planes
It is also necessary to delineate the specific body planes of reference, because they are used to describe structural position and directions of functional movement. The standard position of reference, or anatomic position, has the body facing forward, the hands at the sides of the body, with the palms facing forward, and the feet pointing straight ahead. The body planes are derived from dimensions in space and are oriented at right angles to one another. The sagittal plane is vertical and extends from front to back, or from anterior to posterior. Its name is derived from the direction of the human sagittal suture in the cranium. The median sagittal plane, also called the midsagittal plane, divides the body into right and left halves (Figure 2-2, A, Table 2-1). The coronal plane is vertical and extends from side to side. Its name is derived from the orientation of the human coronal suture of the cranium. It may also be referred to as the frontal plane, and it divides the body into anterior and posterior components (Figure 2-2B). The transverse plane is a horizontal plane and divides a structure into upper and lower components (Figure 2-2C).
TABLE 2-1 Body Planes of Movement
Plane of Movement | Axis | Joint Movement |
---|---|---|
Sagittal | x | Flexion and extension; Lateral to Medial, and Medial to Lateral Glide |
Coronal | z | Abduction and adduction (lateral flexion); Anterior to Posterior, and Posterior to Anterior Glide |
Transverse | y | Medial and lateral rotation (axial rotation) Inferior to Superior, and Superior to Inferior Glide (compression, distraction) |
Axes of movement
An axis is a line around which motion occurs. Axes are related to planes of reference, and the cardinal axes are oriented at right angles to one another. This is expressed as a three-dimensional coordinate system with X, Y, and Z used to mark the axes (Figure 2-3). The significance of this coordinate system is in defining or locating the extent of the types of movement possible at each joint—rotation, translation, and curvilinear motion. All movements that occur about an axis are considered rotational, whereas linear movements along an axis and through a plane are called translational. Curvilinear motion occurs when a translational movement accompanies rotational movements. The load that produces a rotational movement is called torque; a force that produces a translational movement is called an axial or shear force.
Joint motion
Motion can be defined as a continuous change in position of an object and can be described as rotational, translational or curvilinear. Rotational motion takes place around an axis. Translational movements are linear movements or, simply, movement in a straight line. The terms slide and glide have been used to refer to translational movements between joint surfaces. Curvilinear motion combines both rotational and translational movements and is the most common motion produced by the joints of the body (Figure 2-4).
The x-axis extends from one side of the body to the other. The motions of flexion and extension occur about this axis and through the sagittal plane. Flexion is motion in the anterior direction for joints of the head, neck, trunk, upper extremity, and hips. Flexion of the knee, ankle, foot, and toes is movement in the posterior direction. Extension is motion in the direct opposite manner from flexion (Figure 2-5, A). Lateral to medial glide and medial to lateral glide (laterolisthesis) translate through the coronal plane and along the x-axis.
The z-axis extends horizontally from anterior to posterior. Movements of abduction and adduction of the extremities, as well as lateral flexion of the spine, occur around this axis and through the coronal plane. Lateral flexion is a rotational movement and is used to denote lateral movements of the head, neck, and trunk in the coronal plane (see Figure 2-5, B). In the human, lateral flexion is usually combined with some element of rotation. Abduction and adduction are also motions in a coronal plane. Abduction is movement away from the body, and adduction is movement toward the body; the reference here is to the midsagittal plane of the body. This would be true for all parts of the extremities, excluding the thumb, fingers, and toes. For these structures, reference points are found within that particular extremity. Anterior to posterior glide (anterolisthesis) and posterior to anterior glide (retrolisthesis) are translational movements through the sagittal plane and along the z-axis.
The longitudinal axis (y-axis) is vertical, extending in a head-to-toe direction. Movements of medial (internal) and lateral (external) rotation in the extremities, as well as axial rotation in the spine, occur around it and through the transverse plane. Axial rotation is used to describe this type of movement for all areas of the body except the scapula and clavicle. Rotation occurs about an anatomic axis, except in the case of the femur, which rotates around a mechanical axis.4 In the human extremity, the anterior surface of the extremity is used as a reference area. Rotation of the anterior surface toward the midsagittal plane of the body is medial (internal) rotation, and rotation away from the midsagittal plane is lateral (external) rotation (see Figure 2-5, C). Supination and pronation are rotation movements of the forearm. Distraction and compression (altered interosseous spacing or superior or inferior glide) translate through the transverse plane along the y-axis.
Joints are classified first by their functional capabilities and then are subdivided by their structural characteristics. Synarthroses allow very little, if any, movement; an amphiarthrodial (symphysis) joint allows motion by virtue of its structural components; diarthroses, or true synovial joints, allow significant movement. The structural characteristics of these joints are detailed in Table 2-2.
TABLE 2-2 Joint Classification
Joint Type | Structure | Example |
---|---|---|
Synarthrotic | ||
Fibrous | Suture—nearly no movement Syndesmosis—some movement | Cranial sutures Distal tibia-fibula |
Cartilaginous | Synchondrosis—temporary Symphysis—fibrocartilage | Epiphyseal plates Pubes Intervertebral discs |
Diarthrotic | ||
Uniaxial | Ginglymus (hinge) Trochoid (pivot) Condylar | Elbow Atlantoaxial joint Metacarpophalangeal joint |
Biaxial | Ellipsoid Sellar (saddle) | Radiocarpal joint Carpometacarpal joint of the thumb |
Multiaxial | Triaxial Spheroid (ball and socket) | Shoulder Hip |
Plane (nonaxial) | Intercarpal joints Posterior facet joints in the spine |
Bony elements
The bony elements provide the supporting structure that gives the joint its capabilities and individual characteristics by forming lever arms to which intrinsic and extrinsic forces are applied. Bone is actually a form of connective tissue that has an inorganic constituent (lime salts). A hard outer shell of cortical bone provides structural support and surrounds the cancellous bone, which contains marrow and blood vessels that provide nutrition. Trabecular patterns develop in the cancellous bone, corresponding to mechanical stress applied to and required by the bone (Figure 2-6). Bone also has the important role of hemopoiesis (formation of blood cells). Furthermore, bone stores calcium and phosphorus, which it exchanges with blood and tissue fluids. Finally, bone has the unique characteristic of repairing itself with its own tissue as opposed to fibrous scar tissue, which all other body tissues use. Bone is a very dynamic tissue, constantly remodeling in response to forces from physical activity and in response to hormonal influences that regulate systemic calcium balance. Bone, by far, has the best capacity for remodeling, repair, and regeneration of all the tissues making up joint structures. The bony elements of the spine are the vertebral body and neural arch. The cortical shell (compact bone) and cancellous core (spongy bone) play a significant role in weight-bearing and the absorption of compressive loads. The compressive strength of the vertebrae increases from C1 to L5.
Articular cartilage
Articular cartilage, a specialized form of hyaline cartilage, covers the articulating surfaces in synovial joints and helps to transmit loads and reduce friction. It is bonded tightly to the subchondral bone through the zone of calcification, which is the end of bone visible on x-ray film. The joint space visible on x-ray film is composed of the synovial cavity and noncalcified articular cartilage. In its normal composition, articular cartilage has four histologic areas or zones (Figure 2-7). These zones have been further studied and refined so that a wealth of newer information regarding cartilage has developed.
The outermost layer of cartilage is known as the gliding zone, which itself contains a superficial layer (outer) and a tangential layer (inner). The outer segment is made up solely of collagen randomly oriented into flat bundles. The tangential layer consists of densely packed layers of collagen, which are oriented parallel to the surface of the joint.5 This orientation is along the lines of the joint motion, which implies that the outer layers of collagen are stronger when forces are applied parallel to the joint motion rather than perpendicular to it.6 This particular orientation of fibers provides a great deal of strength to the joint in normal motion. The gliding zone also has a role in protecting the deeper elastic cartilage.
The transitional zone lies beneath the gliding zone. It represents an area where the orientation of the fibers begins to change from the parallel orientation of the gliding zone to the more perpendicular orientation of the radial zone. Therefore fiber orientation is more or less oblique and, in varying angles, formed from glucuronic acid and N-acetylgalactosamine with a sulfate on either the fourth or sixth position. The keratin compound is formed with galactose and N-acetylgalactosamine. All of this occurs in linked, repeating units (Figure 2-8).
Articular cartilage is considered mostly avascular and lacks a perichondrium, eliminating a source of fibroblastic cells for repair. Articular cartilage must rely on other sources for nutrition, removal of waste products, and the process of repair. Therefore intermittent compression (loading) and distraction (unloading) are necessary for adequate exchange of nutrients and waste products. The highly vascularized synovium is believed to be a critical source of nutrition for the articular cartilage it covers. The avascular nature of articular cartilage limits the potential for cartilage repair by limiting the availability of the repair products on which healing depends. Chondrocytes, the basic cells of cartilage that maintain and synthesize the matrix, are contained within a mesh of collagen and proteoglycan that does not allow them to migrate to the injury site from adjacent healthy cartilage.7 Moreover, the articular cartilage matrix may contain substances that inhibit vascular and macrophage invasion and clot formation that are also necessary for healing.8 After an injury to the articular cartilage, the joint can return to an asymptomatic state after the transient synovitis subsides. Degeneration of the articular cartilage depends on the size and depth of the lesion, the integrity of the surrounding articular surface, the age and weight of the patient, associated meniscal and ligamentous lesions, and a variety of other biomechanical factors.7 Continuous passive motion has increased the ability of full-thickness defects in articular cartilage to heal, producing tissue that closely resembles hyaline cartilage.9
Ligamentous elements
The primary ligamentous structure of a synovial joint is the joint capsule. Throughout the vertebral column the joint capsules are thin and loose. The capsules are attached to the opposed superior and inferior articular facets of adjacent vertebrae. Joint capsules in the spine have three layers.10 The outer layer is composed of dense fibroelastic connective tissue made up of parallel bundles of collagen fibers. The middle layer is composed of loose connective tissue and areolar tissue containing vascular structures. The inner layer consists of the synovial membrane. The fibers are generally oriented in a direction perpendicular to the plane of the facet joints. This joint capsule covers the posterior and lateral aspects of the zygapophyseal joint. The capsular ligaments provide flexion stability in the cervical spine.1 The ligamentum flavum covers the joint capsules anteriorly and medially and connects the borders of adjacent laminae from the second cervical vertebra to the first sacral vertebra. These ligaments, referred to as yellow ligaments, are composed of a large amount of elastic fibers. This allows for a significant amount of tension to the ligament without permanent deformation. Clinically this is an important characteristic for the spine if it suddenly goes from full flexion to full extension. The high elasticity of the ligamentum flavum minimizes the chances of any impingement of the spinal cord. The anterior longitudinal ligament (ALL) is a fibrous tissue structure that is attached to the anterior surfaces of the vertebral bodies, including part of the sacrum. The ALL attaches firmly to the edges of the vertical bodies but is not firmly attached to the annular fibers of the disc. It is narrowed at the level of the disc. The posterior longitudinal ligament (PLL) runs over the posterior surfaces of all of the vertical bodies down to the coccyx. It has an interwoven connection with the intervertebral disc and is wider at the disc level but narrower at the vertebral body level. Both the ALL and PLL deform with separation and approximation between the two adjacent vertebrae and with disc bulging. The ALL has been found to be twice as strong as the PLL.1 The intertransverse ligaments attach between the transverse processes. They are fairly substantial in the thoracic spine, but quite small in the lumbar spine. The interspinous and supraspinatus ligaments attach between the spinous processes (Figure 2-9).
Synovial fluid
Synovial fluid is an ultrafiltrate of blood with additives produced by the synovium to provide nourishment for the avascular articular cartilage and contribute to the lubrication and protection of the articular cartilage surfaces.11 The identity of the significant active ingredient within synovial fluid that provides the near frictionless performance of diarthrodial joints, has been the quest of researchers for many years. Initially, hyaluronic acid was thought to be the lubricant, but it has not demonstrated the load-bearing properties required within the physiologic joint. Currently lubricin is being investigated as the possible substance within the synovial fluid with the necessary attributes. Lubricin is the glycoprotein fraction of synovial fluid that is secreted by surface chondrocytes and synovial cells. It has been shown to have the same lubricating ability because of the surface-active phospholipids present in lubricin.12,13
Although the exact role of synovial fluid is still unknown, it is thought to serve as a joint lubricant or at least to interact with the articular cartilage to decrease friction between joint surfaces. This is of clinical relevance because immobilized joints have been shown to undergo degeneration of the articular cartilage.14 Synovial fluid is similar in composition to plasma, with the addition of mucin (hyaluronic acid), which gives it a high molecular weight and its characteristic viscosity. Three models of joint lubrication exist. The controversy lies in the fact that no one model of joint lubrication applies to all joints under all circumstances.
The elastohydrodynamic model is a modification of the hydrodynamic model that considers the viscoelastic properties of articular cartilage whereby deformation of joint surfaces occurs with loading, creating increased contact between surfaces. This would effectively reduce the compression stress to the lubrication fluid. Although this model allows for loading forces, it does not explain lubrication at the initiation of movement or the period of relative zero velocity during reciprocating movements.15
In the boundary lubrication model, the lubricant is adsorbed on the joint surface, which would reduce the roughness of the surface by filling the irregularities and effectively coating the joint surface. This model allows for initial movement and zero velocity movements. Moreover, boundary lubrication combined with the elastohydrodynamic model, creating a mixed model, meets the demands of the human synovial joint (Figure 2-10).
Articular neurology
Articular neurology provides information on the nature of joint pain, the relationship of joint pain to joint dysfunction, and the role of manipulative procedures in affecting joint pain. The spinal viscoelastic structures, including disk, capsule, and ligaments, were found to have abundant afferents capable of monitoring proprioceptive and kinesthetic information.16 Therefore, spinal structures are well suited to monitor sensory information and provide kinesthetic perception for coordinated motor control and movement.
Synovial joints are innervated by three or four varieties of neuroreceptors, each with a wide variety of parent neurons. The parent neurons differ in diameter and conduction velocity, representing a continuum from the largest heavily myelinated A α-fibers to the smallest unmyelinated C fibers. All are derived from the dorsal and ventral rami, as well as the recurrent meningeal nerve of each segmental spinal nerve (Figure 2-11). Information from these receptors spreads among many segmental levels because of multilevel ascending and descending primary afferents. The receptors are divided into the four groups according to their neurohistologic properties, which include three corpuscular mechanoreceptors and one nociceptor.17
Type II mechanoreceptors are found within the deeper layers of the joint capsule. They are also low-threshold and again are stimulated with even minor changes in tension within the inner joint. Unlike type I receptors, however, type II receptors adapt very rapidly and quickly cease firing when the joint stops moving. Type II receptors are completely inactive in immobilized joints. Functions of the type II receptors are likely to include the following:
Type III mechanoreceptors are found in the intrinsic and extrinsic ligaments of the peripheral joints, but they had been previously thought to be absent from all of the synovial spinal joints. However, McLain18 examined 21 cervical facet capsules from three normal human subjects and found type III receptors, although they were less abundant than either type I or type II. These receptors are very slow adapters with a very high threshold because they are innervated by large myelinated fibers. They seem to be the joint version of the Golgi tendon organ in that they impose an inhibitory effect on motor neurons. Although the functions of type III receptors are not completely understood, it is likely that they achieve the following:
Type IV receptors are composed of a network of free nerve endings, as well as unmyelinated fibers. They are associated with pain perception and include many different varieties with large ranges of sensations, including itch and tickle. They possess an intimate physical relationship to the mechanoreceptors and are present throughout the fibrous portions of the joint capsule and ligaments. They are absent from articular cartilage and synovial linings, although they have been found in synovial folds.19,20 They are very high-threshold receptors and are completely inactive in the physiologic joint. Joint capsule pressure, narrowing of the intervertebral disc, fracture of a vertebral body, dislocation of the zygapophyseal joints, chemical irritation, and interstitial edema associated with acute or chronic inflammation may all activate the nociceptive system. The basic functions of the nociceptors include the following:
Postural control represents a complex interplay between the sensory and motor systems and involves perceiving environmental stimuli, responding to alterations in the body’s orientation within the environment, and maintaining the body’s center of gravity within the base of support.21,22 Sensory information about the status of the body within the environment emanates primarily from the proprioceptive, cutaneous, visual, and vestibular systems. Researchers23 24 25 have concluded that individuals rely primarily on proprioceptive and cutaneous input to maintain normal quiet stance and to safely accomplish the majority of activities of daily living, but must integrate information from multiple sensory systems as task complexity and challenge to postural stability increase.
A relationship exists between mechanoreceptors and nociceptors such that when the mechanoreceptors function correctly, an inhibition of nociceptor activity occurs.17 The converse also holds true; when the mechanoreceptors fail to function correctly, inhibition of nociceptors will occur less, and pain will be perceived.17
Discharges from the articular mechanoreceptors are polysynaptic and produce coordinated facilitatory and inhibitory reflex changes in the spinal musculature. This provides a significant contribution to the reflex control of these muscles.17 Gillette19 suggests that a chiropractic adjustment produces sufficient force to coactivate a wide variety of mechanically sensitive receptor types in the paraspinal tissues. The A-δ-mechanoreceptors and C-polymodal nociceptors, which can generate impulses during and after stimulation, may well be the most physiologically interesting component of the afferent bombardment initiated by high-velocity, low-amplitude manipulations. For normal function of the joint structures, an integration of proprioception, kinesthetic perception, and reflex regulation is absolutely essential.
Pain-sensitive fibers also exist within the annulus fibrosus of the disc. Malinsky20 demonstrated the presence of a variety of free and complex nerve endings in the outer one third of the annulus. The disc is innervated posteriorly by the recurrent meningeal nerve (sinuvertebral nerve) and laterally by branches of the gray rami communicantes. During evaluation of disc material surgically removed before spinal fusion, Bogduk26 found abundant nerve endings with various morphologies. The varieties of nerve endings included free terminals, complex sprays, and convoluted tangles. Furthermore, many of these endings contained substance P, a putative transmitter substance involved in nociception.
Shinohara27 reported the presence of such nerve fibers accompanying granulation tissue as deep as the nucleus in degenerated discs. Freemont and associates28 examined discs from individuals free of back pain and from those with back pain. They identified nerve fibers in the outer one third of the annulus in pain-free disc samples, but they found nerve fibers extending into the inner one third of the annulus and into the nucleus pulposus of the discs from the pain sample. They suggest that their findings of isolated nerve fibers that express substance P deep within diseased intervertebral discs may impart an important role in the pathogenesis of chronic low back pain. Abundant evidence shows that the disc can be painful, supporting the ascribed nociceptive function of the free nerve endings.26 27 28 29 30 31 32 33 34 35 36
Because structure and function are interdependent, the study of joint characteristics should not isolate structure from function. The structural attributes of a joint are defined as the anatomic joint, consisting of the articular surfaces with the surrounding joint capsule and ligaments, as well as any intraarticular structures. The functional attributes are defined as the physiologic joint, consisting of the anatomic joint plus the surrounding soft tissues, including the muscles, connective tissue, nerves, and blood vessels (Figure 2-12).
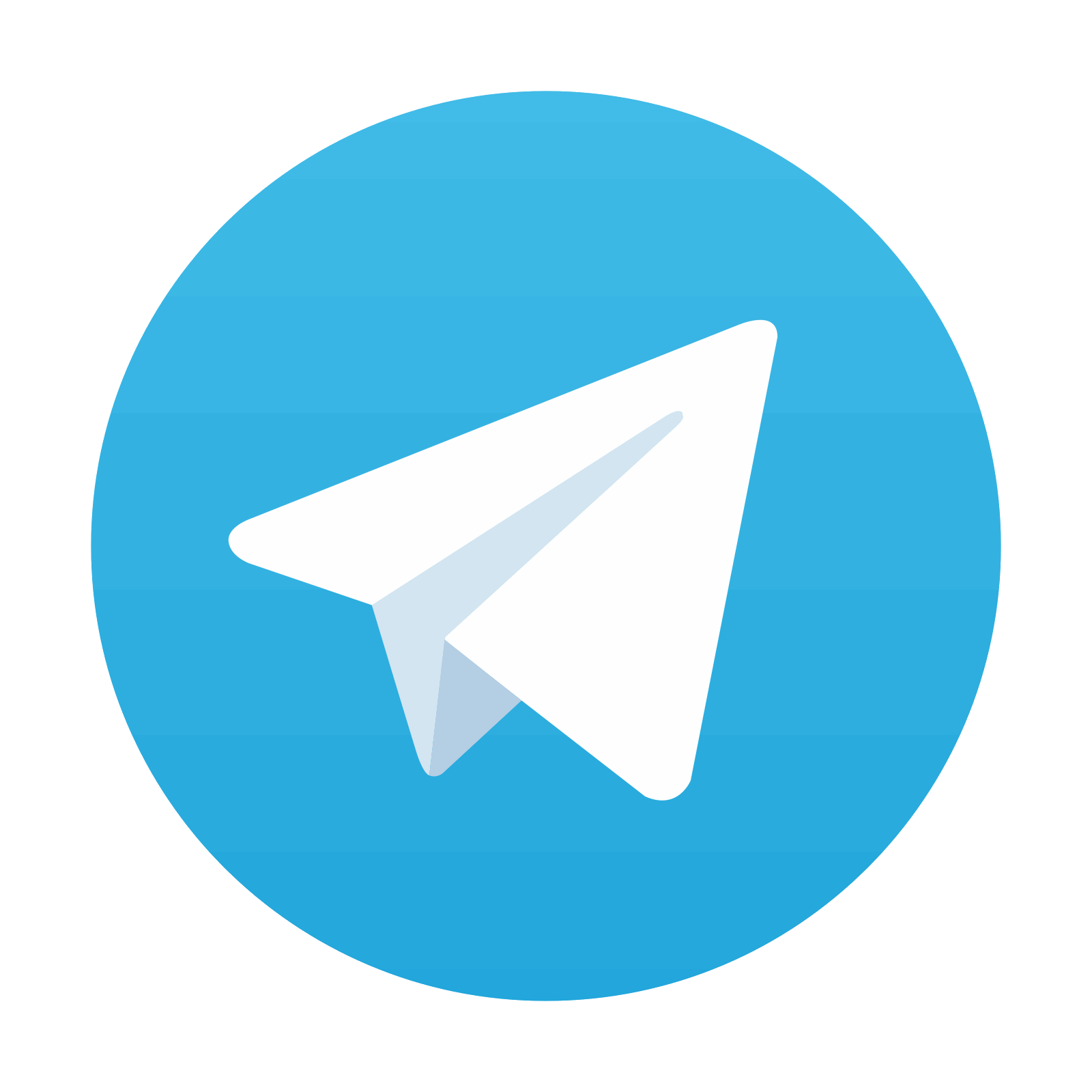
Stay updated, free articles. Join our Telegram channel

Full access? Get Clinical Tree
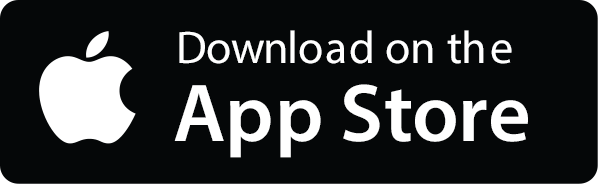
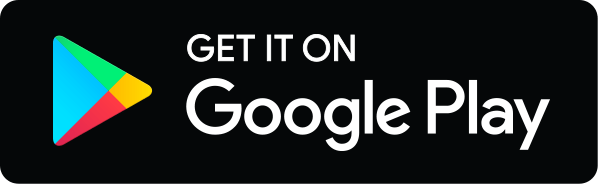