Instability of the Ankle
Murray J. Penner MD, FRCSC

Ankle instability, from the patient’s perspective, is a sense of “giving way” or a feeling that the ankle is about to “give way.”
Ankle instability should be classified as either true- or pseudo-instability, with true instability composed of dynamic or static instability. Because aspects of all three may be present in one ankle, it is helpful to try to determine which component is currently predominant, and which component may have been the initial problem.
Pseudo-ankle instability is a sensation of giving way, accompanied by, and often immediately preceded by an acute pain stimulus. Treatment is directed at the pain stimulus.
Acute ankle instability is most often a result of a sprain of the lateral ankle ligament complex. Treatment is almost always nonsurgical, consisting of rest, ice, compression, and elevation, followed by rehabilitation. Most ankle sprains heal uneventfully, but about 20% lead to chronic ankle instability.
Acute injury of the ankle ligaments is the most common injury associated with recreational and sports activities and the most common orthopedic injury overall. Sprains make up 75% of all ankle injuries, with the vast majority involving the lateral ankle ligaments.
Ankle sprains make up 45% of all basketball injuries, 31% of all soccer injuries, and 25% of all running injuries.
Surgical stabilization may be indicated in patients who have symptoms that are not restricted to risk activities and find problems with bracing and those that have had previous surgery that has removed some of the stabilizing structures.

Ankle instability can be defined, from the patient’s perspective, as a sensation of the ankle “giving way” under load, or a sense of apprehension that the ankle is about to “give way”. These symptoms may be due to true ankle instability, as defined by objective signs of ankle joint laxity, or they may be due to pseudo-ankle instability, where objective findings of laxity are lacking. Distinction between these two is critical.
Pseudo-ankle instability is generally associated with a sensation of giving way, accompanied by, and often immediately preceded by, an acute pain stimulus in the ankle (Table 48-1). The sensation of the ankle giving way is the result of brief reflex inhibition of the ankle musculature, generally caused by the pain stimulus. This is analogous to a knee “buckling” on stairs as a result of patellofemoral chondromalacia. Treatment of pseudo-ankle instability is directed at treatment of the pain stimulus. There is often overlap between true and pseudo-ankle instability, with many of the lesions described in Table 48-1 often being found in association with, and often being caused by, objective ankle laxity.
The focus of this chapter is true ankle instability, from here on simply referred to as ankle instability.
Acute ankle instability occurs when the ankle is subjected to forces outside of “normal” loading conditions, causing subluxation of the talus in the ankle mortise. Most often, this occurs as the result of a plantarflexion and inversion stress, resulting in a sprain of the lateral ankle ligament complex (1). Treatment is almost always nonsurgical, consisting of rest, ice, compression, and elevation, followed by a rehabilitation program. Most acute sprains heal uneventfully, with no persistent symptoms; however, much like in the shoulder,
where acute dislocation is often the initiator of chronic recurrent shoulder instability, acute ankle sprains will lead to chronic ankle instability in as many as 20% of cases (2,3).
where acute dislocation is often the initiator of chronic recurrent shoulder instability, acute ankle sprains will lead to chronic ankle instability in as many as 20% of cases (2,3).
TABLE 48-1 Lesions to Consider in Patients with Symptoms of Chronic Ankle Instability: Potential Causes of Pseudo-instability or Secondary Results of True Instability | |
---|---|
|
Chronic ankle instability has been divided into either functional or mechanical instability. Functional instability, as described by Freeman (2), is the subjective giving way of the ankle, as noted by the patient. To avoid confusion with pseudo-instability, functional instability is now more readily understood as true dynamic instability.
Dynamic instability is defined as insufficiency of the dynamic stabilizers of the ankle, allowing subluxation of the ankle joint while under “normal” loads, within the confines of stable ligamentous restraints. This may be brought about by primary neuromuscular (4) or tendon problems (5), or as the result of lower extremity mal-alignment that places the dynamic stabilizers at a comparative disadvantage (6). Demonstration of dynamic instability can be difficult in the clinical setting, and is often a diagnosis of exclusion, after pseudo-instability triggers and mechanical ligamentous laxity have been ruled out (7).
Mechanical instability has previously been defined as objectively documented hypermobility of the ankle joint, demonstrated with stress radiography (2). It can now be more concisely considered as true static instability or insufficiency of the static ligamentous stabilizers of the ankle. Currently, just as cruciate ligament insufficiency of the knee and gleno-humeral instability of the shoulder are diagnoses based on clinical examination, so, too, is static ankle instability.
Understanding of the definitions, or classification, of ankle instability is critical for understanding the condition, for focusing the evaluation of the unstable ankle, and for communication with other physicians and therapists.
In summary, ankle instability should be thought of as either true- or pseudo-instability, with true instability composed of dynamic or static instability. Because aspects of all three may be present in one ankle, it is helpful to try to determine which component is currently predominant, and which component may have been the initial problem. For example, a patient with a cavovarus foot may have dynamic instability due to malalignment; repetitive giving way may lead to osteochondral injury of the talar dome, resulting in a new pain stimulus, contributing to pseudo-instability. A subsequent major giving way episode may result in a severe sprain of the lateral ankle ligaments, potentially leading to static instability. Recognition of all of the components of such a patient’s instability is important to avoid incomplete treatment of the condition.
Localizing Instability
Lateral ankle instability, where the tibio-talar joint gives way into hindfoot inversion, is by far the most common form of ankle instability (1). It is due to insufficiency of the lateral ankle stabilizers, dynamic or static. Not infrequently, a portion of this instability is due to inversion “laxity” of the subtalar joint (8,9). Rarely, this aspect can become the predominant
aspect of the problem, and this is then primarily termed subtalar instability (10).
aspect of the problem, and this is then primarily termed subtalar instability (10).
Even more rarely described is medial ankle instability (11). This condition as an isolated entity remains somewhat controversial, although it has been described as occurring in conjunction with recalcitrant lateral instability and with adult acquired flat foot disorder (12). It is felt to be present when there is a sense of giving way into hindfoot eversion on a recurrent basis, usually associated with anteromedial ankle pain.
The Scope of Ankle Instability
Acute injury of the ankle ligaments is not only the most common injury associated with recreational and sports activities (13,14), but is also the most common orthopedic injury overall (15). Ankle sprains make up 75% of all ankle injuries, with the vast majority involving the lateral ankle ligaments (14). Reported rates of acute ankle sprains range from 7 to 37 per 1,000 people per year (3,16). The association with sports is very strong, with ankle sprains comprising 45% of all basketball injuries, 31% of all soccer injuries (14), and 25% of all running- and jumping-sport injuries overall (17). These injuries are most common among males, particularly between the ages of 20 and 30 years, though after age 40, where sporting mechanisms no longer dominate, women are more frequently injured (16). On a sport-specific basis, however, the incidence is approximately equal between males and females (14).
Most acute sprains go on to satisfactory recovery with nonoperative therapies. However, 20% to 40% of patients with acute sprains will have ongoing symptoms of instability (18). As a result, persistent instability of the ankle constitutes a major ongoing therapeutic problem.
This chapter describes the anatomy and biomechanics of the ankle and briefly touches on the most relevant aspects of the clinical evaluation of ankle instability. Current nonoperative and operative treatment options are then reviewed.

Underpinning the concept that differentiates ankle instability into static and dynamic subtypes is the fact that the mechanical function of the ankle is afforded by, and constrained by, both static and dynamic stabilizers. The static constraints are provided by the ankle ligaments and the osseous architecture of the joint itself, while the dynamic constraints are the tendons.
Osseous Architecture
Bony constraints are felt to contribute approximately 30% of the resistance to rotational forces about the ankle, with the remainder attributed to the soft tissues (19). The trapezoidal shape of the talus, wide anteriorly and narrow posteriorly, provides strong bony constraint to the tibio-talar joint in neutral flexion or dorsiflexion. But this also allows laxity of the talus within the mortise in plantarflexion, increasing dependence on the soft tissue constraints in this position, and increasing the risk of inversion injury (20).
Recent studies have shown that variations in the osseous anatomy of the ankle and hindfoot are a significant contributor to ankle lateral ankle instability. Van Bergeyk et al. (21) demonstrated a trend toward increased hindfoot varus on computed tomography (CT) scan in patients with chronic lateral instability when compared with controls. It is postulated that this configuration may increase varus forces about the ankle, increasing the likelihood that the soft tissue stabilizers will be overcome in inversion, resulting in giving way and recurrent sprains.
Berkowitz and Kim (22) have verified the earlier hypothesis of Scranton et al. (23) that posterior positioning of the fibula in relation to the ankle mortise is associated with, and potentially a cause of, lateral ankle instability. These studies put forward the concept of an “open ankle mortise,” where posterior positioning of the fibula reduces the relative constraint of the fibula on the talus, possibly allowing increased internal rotation and inversion of the talus. This mechanism remains to be confirmed.
Static Stabilizers—Ligaments
Static instability of the ankle results from insufficiency of the static stabilizers of the ankle, namely the ligaments. Understanding the anatomy of these ligaments is important both for understanding the biomechanics of instability and for achieving satisfactory restoration of ankle and subtalar mechanics at the time of surgery.
The lateral ligament complex (Fig 48-1) is made up of the anterior talo-fibular ligament (ATFL), the calcaneo-fibular ligament (CFL), the posterior talo-fibular ligament (PTFL), and the lateral talo-calcaneal ligament (LTCL). The ATFL spans across the anterolateral ankle joint, blending with the anterior ankle capsule. Its origin on the anterior edge of the fibula is centered 10 mm proximal to the tip of the lateral malleolus. It inserts into the lateral talar neck, immediately distal to the articular surface, 18 mm above the subtalar joint (24). The PTFL originates from the posteromedial surface of the lateral malleolus, stretching transversely medially to insert broadly on the nonarticular posterior surface of the talus (20). The CFL does not originate at the tip of the lateral malleolus. Rather, it originates just below the ATFL origin on the anterior aspect of the fibula, 8.5 mm proximal to the fibular tip (24). It is directed plantar, posterior, and medial (deep to the peroneal tendons), attaching to the lateral aspect of the calcaneus, 13 mm distal to the subtalar joint. It spans both the ankle and subtalar joints and is aligned perpendicular to the posterior facet of the subtalar joint, but parallel to the
subtalar joint axis in the sagittal plane. The angle between the ATFL and CFL in the sagittal plane averages 105 degrees, ranging from 70 degrees to 140 degrees. The LTCL has a variable configuration. It is generally found as a rectangular or triangular structure, spanning the lateral subtalar joint just anterior to the CFL (24). Additional important stabilizers of the subtalar joint are the interosseous ligament (IL) (more deeply located in the sinus tarsi), the anteriorly located cervical ligament (CL), and the lateral root of the inferior extensor retinaculum (IER).
subtalar joint axis in the sagittal plane. The angle between the ATFL and CFL in the sagittal plane averages 105 degrees, ranging from 70 degrees to 140 degrees. The LTCL has a variable configuration. It is generally found as a rectangular or triangular structure, spanning the lateral subtalar joint just anterior to the CFL (24). Additional important stabilizers of the subtalar joint are the interosseous ligament (IL) (more deeply located in the sinus tarsi), the anteriorly located cervical ligament (CL), and the lateral root of the inferior extensor retinaculum (IER).
In dorsiflexion, the CFL is taut in a position nearing vertical, allowing it to function as a true collateral ligament resisting varus talar tilt (20). With plantarflexion, however, the axis of CFL, along with the axis of the subtalar joint, becomes more horizontal and tension in the CFL decreases. This results in the CFL being much less able to resist varus talar tilt in plantarflexion (24). The ATFL becomes taut and more vertical in plantarflexion, taking on the function of a true collateral ligament. It is in the plantarflexed position, where the osseous architecture of the ankle is least stabilizing and the CFL is disadvantaged, that the ATFL acts as the “sole” stabilizer, placing it at most risk for inversion injury.
Motion in the ankle and hindfoot, however, is more complex than simple sagittal plane flexion and frontal plane varus. It also includes rotation of the talus and hindfoot about the long axis of the tibia, as well as anterior/posterior translation of the talus relative to the tibia (24). Cass and Settles confirmed that hindfoot inversion is always accompanied by obligatory external rotation of the tibia (relative to the hindfoot), and that this primarily occurs through the subtalar joint in intact ankles (25). They further showed that increased varus talar tilt is not necessarily seen after isolated release of the ATFL or CFL, as one might expect, though tilting occurs after release of both; however, external rotation of the tibia relative to the hindfoot is increased significantly with release of the ATFL, the CFL, or both. This increase in external tibial rotation does not occur through the subtalar joint (the site of normal tibial external rotation). Rather, it occurs between the talus and the tibia. This work strongly supports the concept that the kinematics of lateral ankle instability includes, at least in part, rotation as well as inversion. This concept fits well with the following observations: (a) true static instability can be seen in the absence of radiographically demonstrable talar tilt (24), (b) the anterior drawer test is primarily rotatory rather than translational (26), and (c) posterior fibular position (which, in effect, externally rotates or “opens” the ankle mortise) predisposes to lateral instability (22). The effect of this is seen in Figure 48-2. Here, opposing lesions on the lateral tibial and talar joint surfaces strongly suggest an injury mechanism where the lateral talar dome experienced edge-loading under the anterolateral tibial lip, a situation only possible with internal rotation of the talus within the mortise. Understanding of this rotational component
is imperative in the assessment and treatment of lateral ankle instability and the associated lesions.
is imperative in the assessment and treatment of lateral ankle instability and the associated lesions.
The subtalar joint is stabilized against inversion primarily by the CFL, with contributions by the LTCL, IL, CL, and IER (10). In neutral or dorsiflexion, where the CFL contribution to overall hindfoot inversion stability is maximal, the ankle is inherently more stable due to bony architecture, reducing the overall risk to the CFL. This likely explains why the CFL is rarely injured in isolation, usually being exposed to injury only when the ankle is in plantarflexion, a situation where the ATFL is most likely to be injured first. This relatively “protected” position of the CFL and the number of additional ligaments which stabilize the subtalar joint likely contribute to the relative rarity of isolated subtalar instability. The LTCL, IL, CL, and IER all act as subtalar stabilizers independent of subtalar or ankle position (27), suggesting that injury to these structures may occur in any position. Only the CFL demonstrates positional variance. It is most susceptible to isolated injury when inversion is imposed on the dorsiflexed hindfoot (28,29). It is this condition that is felt to be necessary to produce isolated subtalar instability (30). This has led some authors to suggest that the best indicator of isolated subtalar instability is a sense of giving way or apprehension when the foot is loaded and in neutral or dorsiflexion, rather than in the plantarflexed position typically associated with ankle instability (31).
The deltoid ligament is the medial stabilizer of the ankle joint (Fig 48-3). Wide variations in the description of its anatomy have been reported. This is not surprising because large variation between specimens is typically noted. A recent study by Milner and Soames (32) described six different component bands of the deltoid ligament. They were divided into four superficial bands (tibiospring and tibionavicular [constant]; superficial posterior tibiotalar and tibiocalcaneal ligaments [nonconstant]), and two deep bands (deep posterior tibiotalar [constant]; deep anterior tibiotalar ligaments [nonconstant]). These findings are somewhat similar to the description of Boss and Hintermann (33), who found five distinct components, three lying superficial (tibiospring, tibiocalcaneal, superficial posterior tibiotalar ligament [nonconstant]) and two deep (deep posterior tibiotalar, deep anterior tibiotalar [nonconstant]). They did not find the tibionavicular to be a distinct ligament, but rather a strong fibrous layer of the ankle capsule. It appeared to be the largest portion of the superficial layer.
Instability of the medial ligaments remains poorly understood. So too does the relationship between instability, acquired flat foot syndrome, and ankle arthritis, although some authors have felt such a relationship exists (12). It has been shown, however, that the deltoid ligament does play a key role in coupling tibial rotation to ankle flexion (34). As a result, the competence of the deltoid ligament may influence
not only medial stability, but lateral stability as well, through this rotational coupling mechanism. External rotation of the leg has been shown by Rasmussen et al. (35,36) to selectively rupture the deep layers of the deltoid, while the superficial ligaments were the primary stabilizer against talar valgus tilt. Clinical medial ankle instability, where the patient senses giving way medially, is not always associated with radiologically or clinically apparent talar valgus tilt; however, it is associated with attenuation of the deltoid components, particularly the deep portion (12). In view of Rasmussen’s findings, this suggests medial instability has a consistent rotational component, again hinting at a connection between medial and lateral ankle instability, as suggested by Hintermann et al. (12).
not only medial stability, but lateral stability as well, through this rotational coupling mechanism. External rotation of the leg has been shown by Rasmussen et al. (35,36) to selectively rupture the deep layers of the deltoid, while the superficial ligaments were the primary stabilizer against talar valgus tilt. Clinical medial ankle instability, where the patient senses giving way medially, is not always associated with radiologically or clinically apparent talar valgus tilt; however, it is associated with attenuation of the deltoid components, particularly the deep portion (12). In view of Rasmussen’s findings, this suggests medial instability has a consistent rotational component, again hinting at a connection between medial and lateral ankle instability, as suggested by Hintermann et al. (12).
Dynamic Stabilizers—Tendons
The peroneal tendons are the dynamic stabilizers of the lateral ankle and subtalar joints. They provide the majority of the eversion force to the hindfoot, with 35% attributed to the peroneus longus (PL) and 25% to the peroneus brevis (PB) (37). Both also function as weak ankle plantarflexors, and the peroneus longus as a plantar flexor of the first ray, potentially inducing forefoot valgus, and secondarily, hindfoot varus. Both tendons run through a fibro-osseous tunnel behind the lateral malleolus, with soft tissue constraint provided by the superior and inferior peroneal retinacula proximally and distally. The floor of the tunnel is defined by the PTFL and the CFL. As the tendons emerge from the sheath near the peroneal tubercle on the lateral calcaneus, the PB continues to insert into the base of the fifth metatarsal, while the PL turns under the cuboid, obliquely crossing the plantar midfoot to insert into the plantar base of the first metatarsal and medial cuneiform.
Although frank weakness of the peroneals has been suggested as a contributor to dynamic lateral ankle instability, this has not been demonstrated (38). Rather than invoking peroneal strength in general as the key to dynamic ankle stability, it is more helpful to consider dynamic ankle stability to be dependent on two factors: (a) the ability to avoid situations where the foot/ankle complex is forced into inversion past the limit that secures stability prior to loading with a compressive force, (b) and the ability, when the ankle is subject to an inversion torque, to prevent unstable situations from progressing to injury of the lateral ligaments and capsule of the ankle/foot complex (4). To put this another way, a person who does not get the ankle complex into situations of excessive plantar flexion and inversion is not at risk, and if the ankle has become subject to an inversion torque, injury can still be prevented if the reaction to counteract the inversion torque is sufficiently fast and powerful (4). The ability to position the foot to avoid injury is under neuromuscular control, and is subject to variation and errors in positioning. Konradsen et al. (39) have demonstrated significant increases in positioning errors after acute ankle inversion injury, even 12 weeks post-injury, suggesting a potential cause of increased susceptibility to recurrent instability. The ability of the peroneals to react quickly to counteract excessive inversion hinges on peroneal reaction time. This has been studied by a number of authors, with the majority indicating increased peroneal reaction time is typically present in patients with dynamic ankle instability (4). These factors form the basis for peroneal recruitment training and proprioception training for the treatment of ankle instability, both dynamic and static.
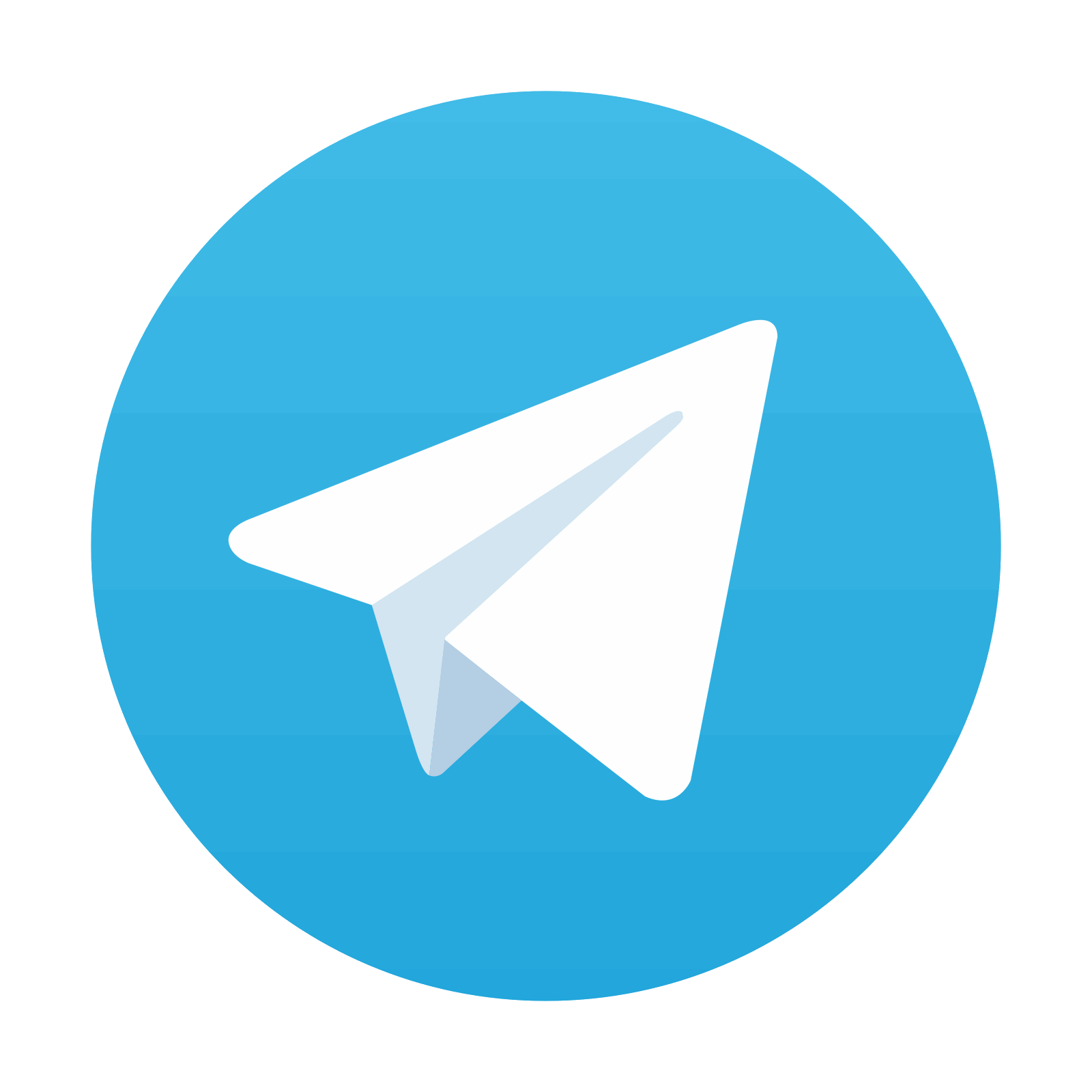
Stay updated, free articles. Join our Telegram channel

Full access? Get Clinical Tree
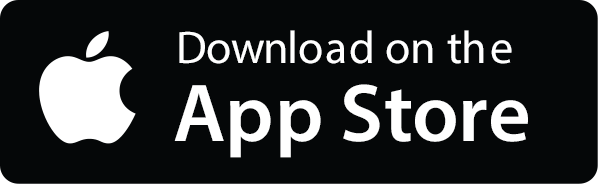
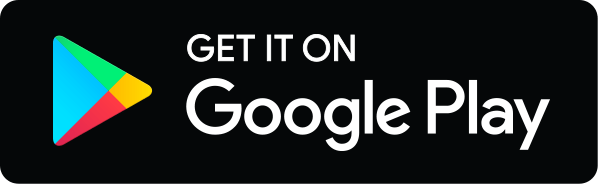